INTRODUCTION
The principle of cellular therapy consists of repairing the damaged tissues or organs with the replacement of non-functional cells for normal cells. The SCs present in the organism, naturally, perform this function, therefore, when the lesions are more extensive, the SCs are not capable of correcting them and the disease is established. Under such situations, the cell therapy with those cells aims at enhancing the natural correction mechanism, concentrating them at the injury site so that they can act more effectively 1. With the advances in medicine, nowadays it is now possible to collect, cultivate and REINFUNDIR the stem cells with therapeutic purpose, forming different tissues, such as bone, liver, and neural 2.
Among the cell therapy options as an adjuvant to the osseous healing, the use of stem cells has been recommended, especially, the mesenchymal stem cells (MSC) by presenting a higher plasticity and bioavailability than the other SC cells. It is expected that these cells, under pathological conditions, help the body overcome the lack of osteoprogenitor source of the other cells involved with the repair and soluble factors for the recruitment of new cell types 3.
In certain types of fractures, the bone grafts, many times are indicated for replacement of a total bone segment. Depending on the type of graft chosen, its function will be a mechanic support and osteoconduction. Thus, the incorporation time is delayed and with complications risk 4.
In the cortical graft the incorporation starts more by the osteoclasts, where the same is revascularized, reabsorbed and replaced slowly by host tissue, with the graft by acting as a support for the formation of new bone. During this resorption phase, the graft is weakened and predisposed to catastrophic failure 4. Its resistance can only be from 50% to 60% of the original in approximately 12 months after transplantation, and sometimes only recovered two to three years after the grafting 5.
In this context, the MSC are currently very researched as a therapeutic alternative in bone grafts, due to their high osteoinduction capacity, i.e., differentiation into osteogenic cells, and their effectiveness in bone recovery is proven 6. This review aims to describe the stem cells characteristics, their current applications in the fractures healing and, especially, their contribution to the bone grafts consolidation.
Stem cells (SC) characteristics. The stem cells differ from other organism cells because they are undifferentiated or not specialized and because they have the ability to generate not only new SC, but also, under certain physiological conditions and experiential, specialized cells with different functions 7.
The SCs can be divided as to their nature in embryonic or adult cells. The embryonic cells are found in the internal cell mass of the blastocyst (embryo). These cells when separated and placed in appropriate conditions are able to cause any of the multiple cell types of an individual. As they are capable of forming a complete body, they are called Pluripotent. The adult SCs are found in any individual after the blastocyst phase. Several tissues (or perhaps all of them) have stem cells, which constitute a reserve from which the organism uses to replace cells that die by apoptosis or lesions caused by wounds or disease. However, in general, the adult SCs are infrequent and their identification is difficult, because they do not have morphological characteristics or others that distinguish them from other cells of that organ 1.
Some types of adult SCs have different degrees of plasticity, ranging from very low as the epithelial SCs, to the very high as the MSCs. The capacity that the MSCs have to originate the most varied of cell a type makes them very interesting for use in cell therapy 1.
The MSCs plasticity mechanisms are not fully understood yet. There are two theories proposed to describe for their differentiation capacity. One of them is the transdifferentiation in which the cell would alter its gene expression to a cellular lineage totally different, resulting in distinct cell types. The transdifferentiation can occur directly, when the cell changes its cytoskeleton and its protein synthesis to redifferentiate into another specific or indirect cell type, when they dedifferentiate in a more primitive stem for, later redifferentiate into another cell type. Another theory proposed is the cell differentiation through fusion. Through this theory the MSCs would be able to merge to an adult-target cell, assuming the gene expression standard of the adult to which it merged. In addition, the MSCs secrete a variety of chemokines, besides expressing receptors for cytokines and growth factors. Therefore, such cells interact with resident cells (niche) and they may induce them, by a paracrine mechanism, to differentiate in distinct lineage cells, according to this signalization 8.
Not only the embryonic cells but also the adult ones are potential application sources. However, due to the practical difficulties of obtaining embryonic SCs arising from ethical, moral and legal aspects, it has been prioritized the use of adult SCs 9.
Before this, studies have been carried out with the objective to induce the pluripotency characteristic to adult SCs. For this reason, researchers have managed to extend the differentiation potential of these cells, by means of nuclear somatic transfer and genetic reprogramming of somatic cells to the embryonic state, through the introduction of determining pluripotency genes (OCT-4, SOX-2, KLF-4, cMYC). These cells are called induced pluripotent stem cells or IPs cells and have a greater capacity of plasticity that the adult SCs found in the body 7.
Mononuclear stem cells and mesenchymal stem cells. The adult SCs can be obtained from bone marrow (BM), peripheral blood, umbilical cord, fetal liver, neural tissue or other various mesenchymal tissues from adult individual, such as synovia, periosteum, muscle tissue or adipose tissue 2.
For many years, the BM was considered the main source of stem cells for applications with stem cell therapy 10. In there exist at least two populations of progenitor stem cells: the hematopoietic progenitor cells (which give rise to blood cells) and mesenchymal progenitor cells (which give rise to the muscle cells, adipose tissue, hepatocytes, osteocytes, chondrocytes and stroma). Both the hematopoietic cells, and the mesenchymal cells are considered to be the mononuclear portion of the BM cells. It is important to emphasize that the MSCs represent a small fraction (0.001-0.01% of the total population of nucleated cells of the BM 11.
So, after collecting the blood from the marrow, the same can be applied directly to the patient 9, centrifuged to obtain mononuclear portion 12 or undergo isolation and expansion in culture 9.
The authors of this review agree that all applications are beneficial, but at the moment that it is applied only the material newly collected from the BM, the concentration of mononuclear stem cells is very low, because this material will also have the blood mature cells, lymphocytes, fragments of stroma and fat. When the blood is centrifuged only to obtain the mononuclear fraction, that is, the hematopoietic and mesenchymal SCs together, it is enhanced a little more the quality of the material applied. However, the option suggested by the authors is, whenever possible, to carry out expansion in culture, because through this procedure it is possible to obtain only the desired lineage of cells in a higher concentration.
In the bone healing several studies report the use of allogeneic and autogenous MSCs. Usually, in these studies, it is used not only the mononuclear fraction of BM, but also the culture expanded of MSCs obtained from various organs. Both can be applied at the injury site, either separately or combined with bone substitutes and biomaterials or by intravenous infusion 3.
Currently, the adipose tissue has been gaining notoriety as being an alternative for obtaining MSC, because in addition to being easily collected in little painful places and in large quantities, studies have shown equal differentiation ability into the tissues of mesodermal origin when compared to the MSC of BM. In this sense, the adipose tissue gains some advantage, since the collection in BM is painful and offers low yield of collected cells 13
When using the adipose tissue as a SC source it is possible to apply the stromal vascular fraction (SVF) 14, which contains a heterogeneous population of cells including circulating blood cells, fibroblasts, PERIÓCITOS and endothelial cells, in addition to the adult SCs; or the SVF can be expanded in culture for obtaining the MSC 7. At the moment, the adipose tissue has been the most explored to MSC collection in the laboratory of cell therapy (LATECER) from our institution (Federal University of Santa Maria, Santa Maria, RS, Brazil.
Although the MSC collection form favors its autogenous use, the possibility of allogenic treatment allows the creation of MSC databases, extending the therapeutic possibilities. Patients in morbid unfavorable conditions could make use of cell cultures already established 3. In LATECER we have been carrying out MSCs cultures derived from adipose tissue (ADSCs) (Figure 1) and subsequent application in animals, allogeneically, obtaining good therapeutic results and not observing reactions of rejection by the animals receiving the cell grafting.
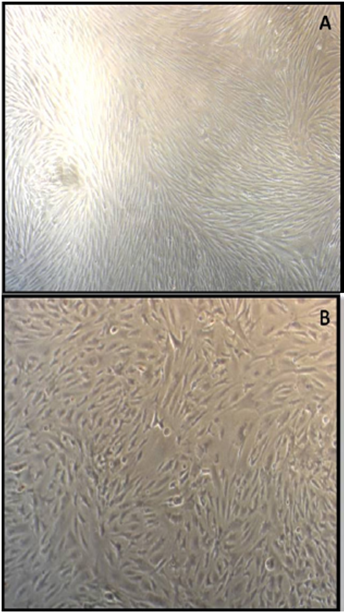
Figure 1 Source: Authors’ personal files. MSCs derived from rabbits’ adipose tissues. (A) ADSCs from interscapular adipose tissue at 21 days of cultivation in laboratory. (B) ADSCs from inter renal adipose tissue at 21 days of cultivation.
The MSCS embryonic origin is the mesodermal, they arise from mesenchymal cells that give rise to connective tissues such as bone, cartilage, fat, as well as the vascular system and hematopoietic 15. Therefore, they can be found in small quantities in perivascular regions of all the adult tissues, including BM, the adipose tissue, the periosteum, muscle tissue and the parenchymal organs 10. Regardless of the source of obtaining, the biological studies show great similarity among these cells 16.
For a population of cells be classified as MSC it is necessary for them to be able to adhere to plastic when grown; that the expressions CD105, CD73 and CD90 be present, and that CD34, CD45, CD14 or CD11b, CD79 or CD19 and HLA-DR are not expressed in more than 95% of cells in culture 17; and that these cells are able to differentiate and give rise to osteoblasts, chondroblasts, hepatocytes, neurons, epithelial cells, renal and cardiac, among others 10. Their high plasticity provides therapeutic promising perspectives, becoming a focus of research around the world 3.
The employment of ADSCs has been considered in the treatment of chronic degenerative diseases, such as in muscular dystrophy linked to the X chromosome of mice, besides being promising for the reconstructive surgical treatments of traumatic origin or not, as in lipoatrophy, congenital or acquired 7. In horses, many studies have already shown their beneficial action in dealing with tendonitis and joints infirmities 15.
In 2011 a study evaluated the transplantation of the total fraction of bone marrow mononuclear cells and the stromal vascular fraction of adipose tissue, associated with the use of cellulose membrane, in rabbits’ skin wounds. Group A received no treatment, Group B received only cellulose membrane and the groups C and D, in addition to the membrane, were also subjected to autologous transplantation of total mononuclear cells or stromal vascular fraction. At the end of the evaluation period, the three treated groups presented statistically significant differences in the wound area compared to the control group. The group that received the stromal vascular fraction from adipose tissue showed the lowest time of wound healing 18.
Performance and identification of mesenchymal stem cells. The MSCs have migratory behavior, i.e., they are able to reach the ill place after the intravenous, intralesional, or perilesional application routes. The cytokines released in sites of inflammation or injury promotes the chemical signals to guide the implanted cells migration. These cells migrate selectively to areas of injury, inflammation and tumors, being involved in tissue repair and formation of stroma associated with the tumor. The MSCs are capable of self-regulating the inflammation cascade factors, including pro-inflammatory cytokines, interleukin-1β, tumor necrosis factor-α and Interferon-γ. These anti-inflammatory effects can have profound influences on the local tissue site and also influence the researchers’ ability to use allogeneic stem cells without inducing a host’s immune response 15.
Moreover, these cells also secrete growth factors that promote the effective tissue revascularization, besides presenting antiapoptotic effect, important in the restoration and maintenance of tissue function. Studies have shown that the progenitor stem cells, particularly those derived from bone marrow, promote tissue repair through secretion of factors that increase the regeneration of damaged cells, as well as stimulate the proliferation and differentiation of endogenous stem cells in different tissues and reduce the inflammatory and immune reactions 15.
Markers of cell surface are bioactive molecules introduced or situated at the outside surface of all cells. These molecules act as receptors, allowing the cells to communicate with each other or bind specifically to certain biological or cellular structures. These molecules that reflect the cellular function can also be used for the marking and identification purpose of specific cell types, including the stem cells. The designation “cluster of differentiation” (CD) refers to markers of cell surface used to characterize cell types. So far no marker was found to identify definitely the MSC being necessary, a combination of markers for characterization of a homogeneous population 15.
The variable expression of many of the lineage markers can be due not only to the different methods of cellular isolation and culture characteristics, but also to the variation in the tissue origin and the various species 17. The lack of a specific marker that sets the MSCs emphasizes their identification based on identification of a set of surface markers and in their ability to differentiate into adipocytes, chondrocytes and osteocytes 16.
The MSC in the presence of beta-glycerol phosphate, ascorbic acid, dexamethasone and bovine fetal serum acquire the morphology of osteoblasts. The chondrogenic differentiation requires that the MSCs be grown in tridimensional environment and in medium with low concentrations of fetal bovine serum and in the presence of TGF-α. The adipogenic differentiation requires a monolayer cell grown in the presence of isobutylmethylxanthine. For differentiation in myocytes it is indispensable the presence of 5-azacytidine 16.
The MSCs are capable of differentiating also in vascular cells or contribute for the formation of blood vessels in the adult individual. It was reported that the MSC, after direct injection into the heart, have the ability to differentiate into cells with endothelial phenotype, cardiomyocytes, vascular pericytes and smooth muscle cells. As seen above, the MSCs can differentiate into cells of various tissues, although the mechanisms that participate in these processes are not fully known 16.
Stem cells as adjuvant in the healing of fractures. The bone healing has three phases: inflammation, in which there is the infiltration of leukocytes that determine the chemotaxis of mesenchymal cells and fibroblasts that will differentiate into osteoblasts; reparation, in which we have the formation of soft callus (loose connective tissue and fibroblasts) and then the hard callus (immature bone tissue); and finally remodeling, in which the bone tissue is formed 2.
The base of the bone in formation and in repair are the MSCs, which respond and produce regenerative cytokines, replicate and differentiate themselves, form the structural matrix and respond to the mechanical demand to restore the functions of the skeleton 19.
Local and systemic growth Factors (GF) stimulate the MSCs to migrate to the focus of the fracture and through the osteoinduction, to differentiate into chondroblasts and osteoblasts and thus, form the bone callus. Peptides BMPs and TGF-β are the main growth factors responsible for the recruitment and differentiation of MSCs to the bony callus formation and subsequent formation of lamellar bone during the remodeling phase 6.
Among the cell therapy options as an adjuvant to the osseous healing, the use of MSCs has been recommended due to presenting a higher plasticity and bioavailability than the other adult SCs. Bone defects in experimental models treated with MSC have demonstrated that favorable biological and mechanical environments result in proliferation and differentiation of MSC into osteoblasts and chondrocytes, and that the presence of stem cells in experimental bone defects decreases the defect healing time 19.
Researchers have created tibial defects in dogs and treated with three different treatments: Sponge of gelatin soaked in saline solution (G1), sponge of gelatin soaked with inhaled BM processed (G2) and a sponge of gelatin soaked with inhaled BM processed and bone morphogenetic protein (rhBMP-2) (G3). The healing was assessed by radiographic studies, and the presence of mononuclear SCs was identified by means of nanocrystal markers Qtracker, in microscopy with fluorescent light, one week after the surgery. Among the cells identified by the marker, cells of bone lineage were found and the radiographic assessments demonstrate accelerated bone growth in animals of G2 and G3. There were significant differences between the G1 and G3 in all time periods, and between G1 and G2 in 30 and 45 days. The researchers concluded that the adult mononuclear SCs supplemented or not with rhBMP-2 is an alternative for bone growth in experimental acute defects of dogs’ tibia 20.
In 2014 a study evaluated the standardization of techniques and the test of association of platelet-rich plasma (PRP) and mononuclear SCs. To this end, dogs’ tibial defects were performed, in a pilot study, which were filled with six different treatments. In dog I, the failure was filled with saline solution; in the second, with PRP; in III, with the total fraction of mononuclear cells (TMCF); in the IV, with the SVF; in V, with the PRP associated to the TMCF; in VI, with the association PRP and SVF. It was carried out clinical evaluations, degrees of lameness, x-rays, perimeter of the thigh, biomechanics and biopsies. The TMCF reached a count and average viability of 2.0x108 cells and 90%, respectively, while the SVF achieved 3x106 cells and 50%. The PRP concentrated, on average, seven times the initial number of platelets out of the total blood, from 250,000 μl-1 platelets in the total blood to 1,750,000 μl-1 platelets in PRP. The authors obtained adequate standardization of the techniques, enabling the test of association between mononuclear stem cells and PRP, as well as their single use, the repair of cortical bone defects, indicating the possibility of the TMCF association and PRP to be the best treatment 14.
Vaz et al 21 in 2010 applied a centrifuged osteogenic of BM in experimental fractures of rabbits’ fibula bone and observed an average increase in bone mineral density of the calluses of 40.3% and the relative amount of bone tissue in 9.4%, without a significant increase in the relative amounts of cartilage or fibrosis. The author considers that the centrifuged favored bone consolidation with qualitative improvement of bone callus.
Kraus and Kirker-Head 19 in 2006 performed a defect of total segment of 21 mm on the femur of dogs and fixed with a plate in order to create a model of non-bone union. After 15 days of the creation of the defect, autologous MSCs of BM were collected, isolated and cultured and associated with a cylinder of hydroxyapatite and implanted in the femur. There was a stimulus for bone formation, with formation of new adjacent bone to the implant, infiltrating into the receptor bone.
Barros et al 22 in 2001 treated failures of total segment of rabbits’ radius with integral BM or BM centrifuged applied percutaneously. The percutaneous route application proved to be a simple method, with minimal trauma to the tissues, not introducing devitalized tissue and, thus, reducing the risk of infection and interference in bone repair. The radiographic evaluation of the operated limbs, held on the second day of grafting and a week after, demonstrated the efficiency of the application of BM and its role in bone formation.
Researchers have investigated the healing potential of calcium alginate associated with the MSCs of bone marrow grown and differentiated into osteogenic cells in cranial bone defects in sheep. After six weeks, it was observed considerable bone growth in the group in which MSCs were used compared with the control group, which received only calcium alginate. After 18 weeks there was almost total reparation in the experimental group when evaluated by computed tomography 23.
Contribution of stem cells in the healing of bone grafts. As the healing of a cortical bone graft passes through a critical period during the incorporation, in which the graft is gradually absorbed for later formation of new bone, becoming weak and vulnerable to rupture 4, one of the resources used by orthopedic surgeons to avoid complications is the application of osteosynthesis material that enhances the stability of the interfaces receptor-graft 24. However, giving up adjuvants to accelerate the healing process to decrease the fracture risk is extremely welcome. The MSCs applications in bone grafts has been used precisely for this purpose, because in addition to their ability to differentiate into bone cells, they are also capable of recruiting FCs to the fracture focus, thus stimulating the additional recruitment of other MSCs present in bone tissue and enhancing the bone repair process 6. These authors state that there are no doubts on their benefits, being BM the main obtaining source for use in bone grafts.
Del Carlo et al 25 in 2007 compared the consolidation of cortical bone allografts of dogs associated with different adjuvants. At the group 1 (control) only the allograft was placed in the tibial defect. At the group 2, the graft-host interfaces were filled up only with autogenous bone graft. In group 3 it was associated with autogenous bone graft, the morphogenetic bone protein (MBP) and finally in group 4, it was associated the SC from BM with MBP. The precocity of radiodensity in the graft and the full integration of the interfaces were observed in greater intensity in groups 4,3.2 and 1, respectively. Microscopically, the largest bone formation took place, by the order in groups 4,2,3 and 1. The authors concluded that the group with association of SC from BM with the MBP contributed to the precocity of the bone formation, resulting in osteogenesis and osteoinduction in the interfaces, respectively.
Gomes 26 in 2008 evaluated the bone healing of the autograft from the iliac crest associated with BM cellular therapy. Bone defects were created in the rabbits’ jaw, and the control group treated only with the autograft from the iliac crest and the experimental group with the autograft associated with the mononuclear BM cells of autogenous of the femur. By the radiographic analysis it was showed a gradual increase of bone density with 85.71% of the animals in the experimental group and 42.85% in the control group presenting bone bridge formation 28 days after surgery. In the histopathological analysis at 15 days, the grafts were easily visualized and the phagocytic cells activity was intense. Whereas at 30 days, its visualization was harder and when it was possible, only a remnant was noticed. Based on the results obtained the authors suggest that the addition of BM mononuclear cells favors the healing of the autograft in rabbits’ mandibular defects.
Li and Li 27 in 2005 used MSC from bone marrow grown and differentiated into osteoblasts, associated with allogenic demineralized bone graft in the filling of rabbits’ mandibular defects. Along the weeks, radiographic examinations were performed to evaluate the healing, being that in the twelfth week it was hardly seen the defect in the experimental group, which presented a density similar to that of the rabbits’ mandibular bone. Whereas in the control group, at the portion below the defect it was still possible to see radiolucent areas.
Researchers carried out defects in mice’s cranial vault, being that at the group 1 the same was rebuilt with bovine lyophilized bone and MSC and at the group 2 it was used only lyophilized bone. The lyophilized bone from group 1 was left on the cultivation plate 24 hours prior to the procedure. There was significant difference between the groups, being that from the point of view of histological regeneration the grafts containing MSCs showed better results when compared to those without combination with cells. The authors concluded that the use of MSCs could mean an improvement in the quality of bone grafts and an optimization in time for the integration of these grafts 28
Pelegrine et al 29 in 2013 carried out bilateral defects in the parietal bone in five experimental groups (n=6) and a control group (=3) of rabbits. In the G1 defects were covered only by a bone substitute of bovine origin (Bio Oss), the G2 the defects were filled by Bio Oss and a fresh bone marrow aspirate, in G3 by Bio Oss and the BM mononuclear fraction, the G4 by Bio Oss and MSC from BM and the G5 by Bio Oss and MSM from adipose tissue. One of the defects of each animal was covered by a collagenous membrane and the other was maintained without coating. After two months, the animals underwent euthanasia and their parietal bones were analyzed through histomorphometry. The authors could observe that in the control group there was no bone formation and the other groups the cellular therapy maximized the regenerative results through an osteoconductive biomaterial. The concentration bone marrow cells, in addition to being less complex than techniques of cell culture, presented regenerative important results, seeming to represent a methodology clinically plausible.
De Mello and Oliveira et al 30 in 2014 created bone defects in the rabbits’ skull, which were reconstructed with titanium cylinders. Subsequently, the animals were divided into two groups: group 1 had the cylinder filled with xenograft and the group 2 with xenograft associated with mononuclear fraction of the bone marrow. After two months the animals were subjected to euthanasia and the cylinders along with the parietal bone were analyzed regarding the bone volume formed inside the cylinders and histophotometry. Once more the groups where there was the association of the mononuclear fraction of the bone marrow showed superiority in the improvement of the bone healing and the bone integration.
Long et al 31 in 2014 grew MSCs from BM around cortical allografts and applied in femoral defects in a group of mice. At the other group, the allograft was applied without the cells. With the radiographic evaluations, histological cuts, and biomechanical tests in up to six weeks after the surgery, the authors concluded that the allograft is associated to the MSC induced the increase of bone callus formation, as well as the osteointegration host-graft. The biomechanical tests also exhibited a significant increase at the structural and functional proprieties of the femurs grafted with the cells. Finally, the allografts associated with MSC exhibited a better osteogenecity during the critical defect repairs, demonstrating the viability of this treatment to accelerate the healing process of bone grafts.
CONCLUSIONS
Most of the studies performed so far have shown promising results regarding the application of adult stem cells in the fractures healing and bone grafts. Thanks to its osteogenic potential the healing time decreases and this is reflected in a lower risk of complications in the postoperative period, such as non-union and the graft weakening, with subsequent fracture.
The obtaining of stem cells from adipose tissue is an alternative to bone marrow with some advantages, such as ease of collection, abundance of content and results in less painful process. In the years from 2007 to 2010, at LATECER mononuclear fraction of BM and SVF from adipose tissue were used, however, from 2011 on researches were begun using cells cultured from tissues that offer lower morbidity in the collection harvest, such as adipose tissue and dental pulp.
The possibility of using not only the total fractions of tissues stem cells, but also their expansion in culture and differentiation allow their application according to the reality of each surgical center, being that regardless of the procedure used, the healing shall always be benefited.