Services on Demand
Journal
Article
Indicators
-
Cited by SciELO
-
Access statistics
Related links
-
Cited by Google
-
Similars in SciELO
-
Similars in Google
Share
CT&F - Ciencia, Tecnología y Futuro
Print version ISSN 0122-5383On-line version ISSN 2382-4581
C.T.F Cienc. Tecnol. Futuro vol.1 no.3 Bucaramanga Jan./Dec. 1997
J. C. RAMÓN*1 and T. CROSS2
1Colorado School of Mines, Geology Department/ Golden CD 80403
E-mail: jramon@slate.mines.edu
2Ecopetrol, Instituto Colombiano del Petróleo, A.A. 4185 Bucaramanga, Santander, Colombia
*To whom correspondence should be sent
ABSTRACT
About 1 .600 m of core from two major oil fields, and 600 m of outcrop sections near to one of the fields, integrated with thousands of core, side-wall core and outcrop porosity and permeability measurements show that petrophysical properties and reservoir continuity change consistently with the Accommodation to Sediment Supply (A/S) ratio. Channel sandstones exhibit regular, recurring motifs that are associated with changes in A/S conditions. Manifesting the lowest accommodation conditions are amalgamated channel sandstones, up to 5-m-thick, with intraclast-rich bases capped by up to 1 -m-thick floodplain mudstones. AT higher A/S conditions, slightly amalgamated channel sandstones have lateral accretion surfaces and are capped by a thicker unit of floodplain mudstones. Channel sandstones in the highest A/S conditions are single-storied, possess conspicuous lateral accretion surfaces with thick mud drapes, and have a thick cover of overbank and floodplain deposits. Detailed well log correlations, oil production and pressure data support that the former are more lateral continuous while the I after form laterally discontinuous bodies embedded in floodplain mudstones. Petrophysical properties are closely associated with subtle variations in fades, particularly degree of preservation of original bedforms, and both are stratigraphically sensitive. Petrophysical properties of identical sedimentological fades change regularly as a function of itsstratigraphic position because of variations in the rates of accumulation and degree of preservation of the sediments. In the case of fluvial strata deposited during an increase in A/S, porosity and permeability are highest in trough cross-stratified sandstones immediately above channel scour bases, and decrease upward to the next scour base. Successive channel sandstones within the same stratigraphic sequence and channel sandstones from one sequence to the next have progressively lower porosity and permeability values in an overall increase in the A/S. An inverse trend is observed during a decrease in A/S.
Keywords: Colombia, fluvial, reservoir characterization, porosity, permeability.
RESUMEN
Este estudio muestra que las propiedades petrofísicas y la arquitectura de los yacimientos en canales fluviales y depósitos de crevasse dependen de las condiciones de acomodación y suministro de sedimentos -A/S -durante la sedimentación. Estos resultados se basan en la integración del análisis sedimentológico de aproximadamente 1 .600 m de núcleos de perforación de dos campos petroleros y unos 600 m de afloramientos en el Valle Medio del Magdalena con medidas de porosidad y permeabilidad de núcleos y afloramientos. La arquitectura y sucesiones de fades en areniscas fluviales se presentan en patrones repetitivos asociados a cambios de A/S. En condiciones de A/S bajas, las arenas de canal tienden a ser amalgamadas, formando cinturones de canal hasta de 5 m de espesor y asociados a delgadas unidades lodosas de llanura de inundación. Bajo condiciones de A/S mayores, el amalgamamiento se reduce y se preservan superficies de acrecían lateral y mayores espesores de depósitos de llanura de inundación. Las arenas de canal de máxima A/S, son más lodosos, poseen claras superficies de acrecían lateral y ocurren como cuerpos aislados enbebídos en lodos de llanura de inundación. La correlación detallada de pozos cercanos, datos de producción y presiones indican que la extensión lateral de los cuerpos de arena es proporcional al grado de canibalismo. Canales amalgamados son más continuos que canales individuales. Las propiedades petrofísicas están íntimamente ligadas al grado de preservación de las fades y cuerpos originales. Como la preservación relativa depende de A/S, esta relación controla los valores de porosidad y permeabilidad incluso en fades idénticas. En arenas fluviales, los valores de porosidad y permeabilidad son mayores en bajas condiciones de A/S, donde el mayor canibalismo entre las arenas elimina los componentes finos y mejora la selección. A mayores condiciones de A/S, la mayor preservación de los componentes originales resulta en una mayor proporción de finos y peor selección.
Palabras clave: Colombia, fluvial, arquitectura de los yacimientos, porosidad, permeabilidad.
INTRODUCTION
Reservoir geometry, compartmentalization, and porosity and permeability values and distributions influence fluid flow, sweep and recovery efficiencies. An understanding of the geologic controls on these attributes is beneficial for reservoir characterization, fluid-flow simulation, optimal field development and enhanced oil recovery.
Geometry, continuity, heterogeneity and petrophysical properties of a reservoir depend on a variety of phenomena including the depositional environment, the accommodation conditions during the time of sediment accumulation, tectonic history, provenance and diagenesis. Most basic lithological, petrophysical, geometrical and reservoir continuity attributes of strata originate during sediment accumulation. Sedimentologic and stratigraphic studies attempt to represent and predict these rock properties in a four-dimensional (time-space) context. Exceptions are rocks which have been extensively altered by diagenesis or fracturing.
Traditionally, rock attributes important to reservoir characterization and production are studied and assessed from the perspectives of depositional environments and facies models (e.g., Amaefule, et al., 1994; Weber and Van Geuss, 1990; Jennings, 1987; Pryor, 1973; Shelton, 1973; le Blanc, 1972). Facies models are considered representative of sediment accumulated within their respective depositional environments independent of time or place. They are constructed from facies assemblages observed in examples of strata considered equivalent in terms of their environment of accumulation (Walker, 1979; Miall, 1996). Facies models assume that deposits of a geomorphic environment are preserved more or less intact; the facies components of strata are products only of the processes operating in the geomorphic environment, not subject to modification or influence by stratigraphic controls.
By contrast, a few previous studies have demonstrated that physical characteristics of facies, facies assemblages and successions, and stratigraphic architecture of identical facies tracts vary with stratigraphic position (Cross et al., 1991; Gardner, 1993; Sonnenfeld and Cross, 1993; Fajardo, 1995). There are direct relations between dynamic changes in accommodation/ sediment supply [A/S] ratio, the numbers and proportions of geomorphic elements preserved in strata, and the character and assemblage of facies preserved. The stratigraphic record produced from identical environments varies as A/S conditions change. Stratigraphic controls contribute to reservoir geometry, facies diversity and petrophysical properties at least as much as do the geomorphic processes of the environment in which the sediments accumulated. Facies models do not account for these dynamic changes in A/S conditions, the degree of preservation of geomorphic elements, and, therefore, for stratigraphically sensitive changes in facies attributes, components and proportions for identical facies tracts.
This chapter illustrates that large-and small-scale reservoir rock properties and production history are better understood and predicted from a stratigraphic perspective than from the perspective of facies models, only For strata of identical environmental origins, changes in sandstone heterogeneity, geometry and lateral continuity, and values and ranges of petrophysical properties are associated with changes in A/S conditions within stratigraphic cycles.
Channel sandstones deposited in low A/S conditions are amalgamated and areally continuous. The finer sand and muddy sediments composing the upper parts of channel bars and most floodplain sediment are not preserved. The resulting reservoir sandstone is more homogeneous, porosity is good, porosity ranges are narrow, and reduced clay content enhances permeability. Higher A/S conditions result in a lower degree of amalgamation and cannibalism of channel deposits and increased preservation of floodplain sediment. Channel sandstones tend to be single storey, isolated bodies embedded in floodplain deposits.The geomorphic elements composing the individual channels are more fully preserved and more mud is preserved. Porosity and permeability profiles decrease strongly upward. Where several channel sandstones are interconnected, major changes in rock properties and internal permeability barriers may exist between channel sandstone elements. The increased proportion of fine sand and mud reduces permeability and increases capillary pressure.
These unidirectional changes in alternately increasing and decreasing A/S conditions can be interpreted from one-dimensional cores and well logs. Stratigraphic and sedimentologic responses to these changes may be used to predict reservoir properties and architecture. This study of subsurface and outcropping strata from the Middle Magdalena basin shows that sedimentologic and stratigraphic attributes important for reservoir characterization, production and management change with A/S conditions, and can be predicted from stratigraphic analysis of core and well logs.
STUDY AREA AND DATA BASE
This study pertains to an area of about 180 km2 in the central part of the intermontane Middle Magdalena basin, Colombia. Most hydrocarbon production comes from fluvial channel and crevasse splay sandstones of the Lower Tertiary La Paz, Mugrosa and Colorado Formations. More than 6.000 ft of cores and 4.117 porosity and permeability measurements from these formations in the Lisama, Tesoro, Casabe and La Cira oil fields were examined. Scintillometer and permeability measurements were taken from outcrops near these oil fields (< 20 km away) in strata of the same ages and facies as in producing intervals. Figure 1 summarizes the sources of data.
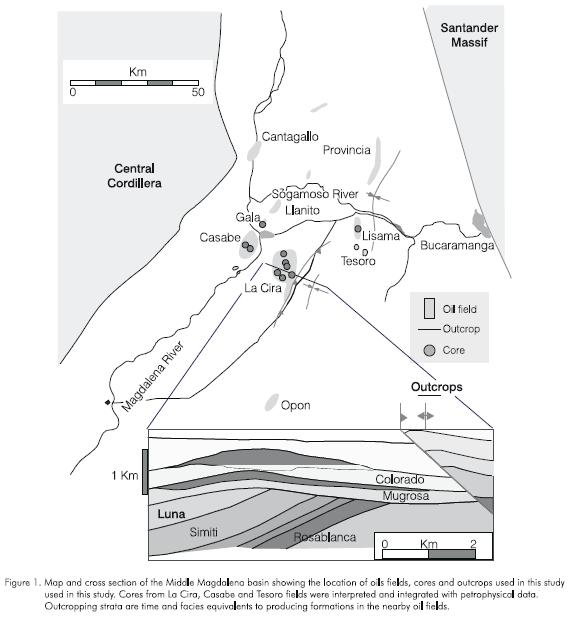
TRADITIONAL FOCUS ON RESERVOIR PROPERTIES AS A FUNCTION OF ENVIRONMENTS, FACIES AND FACIES MODELS
Traditional evaluations of small-and large-scale sedimentologic and stratigraphic attributes important to reservoir characterization and production are conducted from the perspectives of depositional environment and facies models (Amaefule, et al., 1994; Weber and Van Geuss, 1990; Jennings, 1987; Pryor, 1973; Shelton, 1973; Le Blanc, 1972). These attributes include geometry, volume and continuity of lithosomes; degree of heterogeneity; type and degree of compartmentalization; and facies components, diversity, and petrophysical attributes. Extent and continuity of shales that might act as barriers to fluid flow also are evaluated from these perspectives (Mattax and Dalton, 1990, their table 4.3). Porosity and permeability values and distributions are plotted with respect to facies but not with respect to stratigraphic position (Warren and Price, 1961; Harris, 1975; Mattax and Dalton, 1990; Weber and Van Geuss, 1990). This is an extension of the assumption that petrophysical properties are dependent only on environmentally determined facies. Little or no attention is given to stratigraphically controlled variability of rock attributes that are important to reservoir production and management.
Facies models are considered typical of sandstone body morphologies and component facies for their respective environments without regard to time, place or stratigraphic position. For example, progradation of a barrier island or shoreface forms a "tabular" sandstone body (Figure 2a), whereas a fluvial channel tends to form narrow, elongated sandstone bodies (Figures 2b and 3a). Sandstone geometry facies models, such as those illustrated in Figures 2 and 3, are used to describe reservoir geometry early in field development so that the direction and probable extent of specific hydrocarbon-bearing units could be extrapolated from limited data.
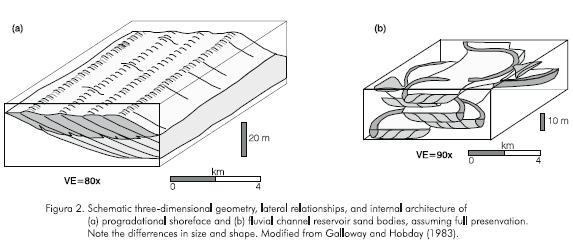
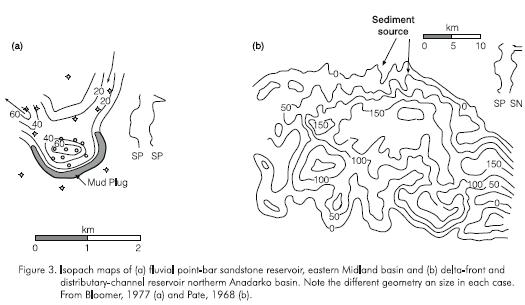
Such facies models are abundant for continental strata. Friend (1983) suggested two dominant modes of occurrence of fluvial sandstones; the areally extensive "sheet" and the more laterally restricted "ribbon" sandstone bodies. Schumm (1977) proposed a fourfold geomorphic classification of fluvial sandstones. Other authors recognized a greater variety of channel sandstone morphology and proposed up to 15 facies models (Miall, 1996).
There is a general opinion that strata of fluvial systems are unorganized because they are heterogeneous and compartmentalized, facies occur in a patchwork mosaic, and lateral transitions are abrupt. Consequently, prediction of reservoir continuity and geometry in continental strata has been considered difficult or impossible (Weber and Van Geuss, 1990). Although Bridge and Leeder (1979) showed theoretical transitional changes in large-scale geometry and architecture of channel sandstones with respect to stratigraphic position, only a few studies have described transitional changes in geometry, morphology, degree of amalgamation, areal continuity and architecture of channel sandstones from a stratigraphic perspective (Sanchez-Moya et al., 1996; Olsen et al., 1995; Cross et al., 1993; Davies et al., 1993; Gardner, 1993; Melvin, 1993; Willis, 1993; Miller, et al., 1990; Puigdefabregas and Van Vliet, 1978).
Theoretical and observed regular transitions in large-scale geometric and architectural attributes should suggest similar regularity in small-scale attributes of strata as a function of stratigraphic position.
Traditionally, however, reservoirs are divided into different facies representing different geomorphic elements or environments. Petrophysical measurements are recorded and evaluated in these facies irrespective of stratigraphic position. Permeability and porosity averaging normally is done with conventional statistics (arithmetic, geometric or harmonic means) or with geostatistical techniques. The means and ranges of petrophysical properties are applied to that facies at all stratigraphic positions. Again, there is little recognition that petrophysical properties of identical facies change parallel with changes in degree of preservation of original geomorphic elements. Analyzing and grouping petrophysical properties by facies alone, irrespective of their stratigraphic position, increases the scatter in the values of the measured attribute.
The following discussions review some large-and small-scale attributes of strata representing identical environments that are stratigraphically sensitive. Stratigraphic variations in geometry, architecture and continuity of facies tracts are related to degree of cannibalization, amalgamation and preservation of original geomorphic elements. Small-scale, stratigraphically controlled variations in identical sedimentologic facies, in turn, are related to stratigraphic variations in porosity and permeability. The combination of stratigra-phically sensitive small-and large-scale attributes strongly influence reservoir performance and production history.
RESERVOIR GEOMETRY AND COMPARTMENTALIZATION
Origins and types of reservoir compartments
In the absence of structural overprinting that influences fluid flow, the most common reservoir compartment boundaries are coincident with the stratigraphic boundaries of genetic sequences (Cross et al., 1993). Hanson (1989) and Little (1994) showed that the limits of producing shoreface reservoirs are defined by depositional limits of the shoreface facies tract within each genetic sequence of the Eagle Sandstone in the Bear Paw and Tiger Ridge gas fields, Montana (Figure 4). Marine shelf mudstones which bound the seaward portions of the genetic units are seals between superposed reservoirs.
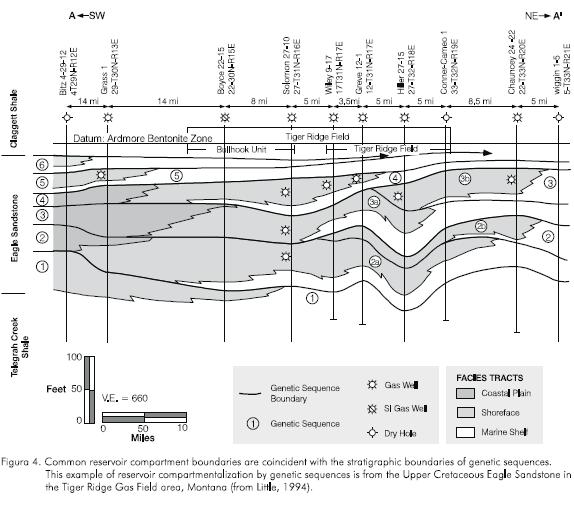
Other common stratigraphically controlled fluidflow compartments are the smaller-scale, offlapping stratigraphic packages arranged as shingles within genetic sequences. These form by episodic progradation of environments with depositional topography. For example, when a prograding delta lobe switches to another position or temporarily ceases to prograde, muds may drape the surface of the lobe. When another lobe returns to the same geographic location, or when progradation resumes, the resulting deposit is an overall coarsening and shallowing-up facies succession interrupted by low-energy mudstone drapes.
In both cases, high-resolution stratigraphic correlation permits accurate representation of reservoir compartmentalization in a spatial and temporal framework. High-resolution correlation is the best way to define heterogeneities in the reservoir that form different fluid-flow compartments and that control fluid-flow pathways and rates.
In alluvial systems the boundaries of the main reservoir compartments also are coincident with stratigraphic boundaries of genetic sequences and facies tract offsets associated with these boundaries. Channel sandstones and in some cases crevasse complexes are the reservoir units; overbank mudstones are seals and fluid flow barriers. The size and geometry of alluvial sandstone reservoirs are defined by the degree of amalgamation and connectivity of fluvial channel sandstones, the concomitant elimination of mudstone by cannibalization, and by the extent of progradation in crevasse complexes.
The continuity and geometry of coarse members and their proportion with respect to the floodplain and lake mudstones depend on a variety of processes including subsidence rate, sediment supply, and avulsion rate, among many others. Allen (1978, 1979), Bridge and Leeder (1979) and Blakey and Gubitosa (1984) suggested that fluvial channelbelt sands deposited during high frequency of avulsion relative to subsidence rate form vertically and laterally interconnected, blanket-like sandstone bodies that could function as single compartment reservoirs. By contrast, channelbelt sandstones of identical depositional systems may occur as isolated, stringer-like reservoir sandstones if deposited during low frequency of avulsion relative to subsidence rate. In this case, each sandstone body would function as a separate fluid-flow compartment.
Vertical transition between these two end situations are observed in the Middle Magdalena basin. Figure 5 shows a correlation between wells in the Aguas Blancas field. Fluvial channels toward the base of the cycles tend to be interconected and associated with few overback mudstones, while toward the top of the cycle floodplain mudstone proportion increase and channels become thinner and laterally discontinuous.
Origin of Changes in Facies Composition, Degree of Amalgamation, and Stratigraphic Architecture
Channel sandstones in the Middle Magdalena basin range transitionally from isolated sandstone bodies embedded in floodplain mudstones to amalgamated multi-storied, areally continuous sheet sandstones (Figure 5). Sandbody width/depth ratios range from about 100 to more than 500. Transitional changes in channelbelt sandstone morphology, geometry, facies components and successions, and diversity of facies tracts are stratigraphically sensitive, and these are related to changes in the A/S conditions through time.
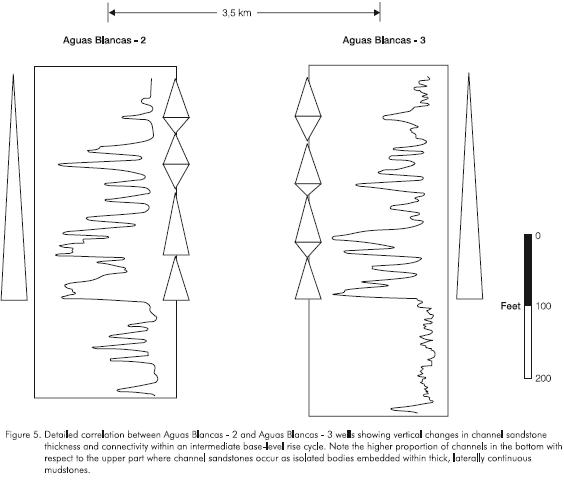
During high A/S conditions most of the original geomorphic elements of continental environments are preserved in strata. The high aggradation rate of the entire alluvial plain produces isolated channel sandstones separated by floodplain, lake or crevasse-splay deposits (Figure 6a). These channel sandstones are composed of fully preserved geomorphic elements of the river, from the base to the top of the channel, resulting in typical pointbar facies successions (Figure 6b).
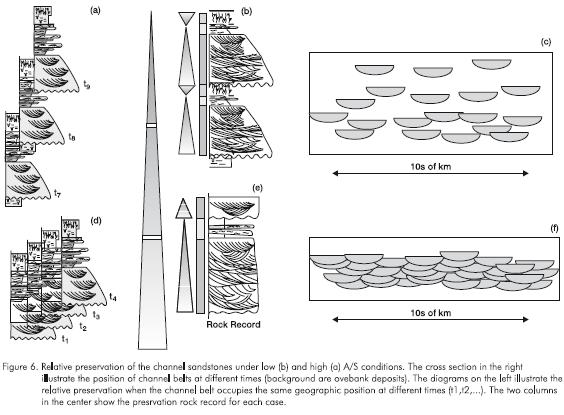
Geomorphic elements of the floodplain also are preserved more or less intact, as they aggrade in tamdem with river bed aggradation. After a series of avulsion events to low areas of the floodplain, a channelbelt might reoccupy the same geographic position it previously occupied. The channel will erode to its base level. If the previously deposited channel sandstones are below this erosion level, they are incorporated into the stratigraphic record without further modification (Figure 6c).
As A/S conditions decrease, geomorphic elements of channels and floodplain are increasingly cannibalized during channel migration and avulsion. As A/S conditions decrease, progressively more of the least easily preserved geomorphic elements are cannibalized and the stratigraphic record is enriched in those portions of geomorphic elements which have the highest preservation potential.
Under low A/S conditions, channel erosion removes the upper parts of previously accumulated sediments (Figure 6d) since they are not carried beneath new base levels through time. The migration of channels and relatively high rate of avulsion with respect to the rate of accommodation space increase produces laterally amalgamated, interconnected channel sandstone belts (Figure 6f). The deposits of a meandering channel consist predominantly of cut-and-fill macroforms composed of amalgamated trough-cross bedded to massive sandstones, because only the geomorphic elements at the base of the channel are preserved (Figure 6e).
Figure 7 illustrates the changes in channel architecture with variations in the A/S conditions trough time. This 220-feet-thick section of the Gala-7 well shows transitional change in channel sandstone facies and architecture in a series of small-scale base-level cycles within longer-term cycles. Note a parallel change in the degree of amalgamation and relative proportion of channel sandstones from bottom to top. Also, facies diversity in channel sandstones increases upward. The channel sandstones toward the base of the cycle (Figure 7c) consist of scour and fill macroforms. No evident trend in grain size is apparent and no floodplain mudstones are preserved. By contrast, the channel sandstone toward the top of the cycle (Figure 7a) have various facies and grain sizes and it is associated with thick floodplain mudstones.
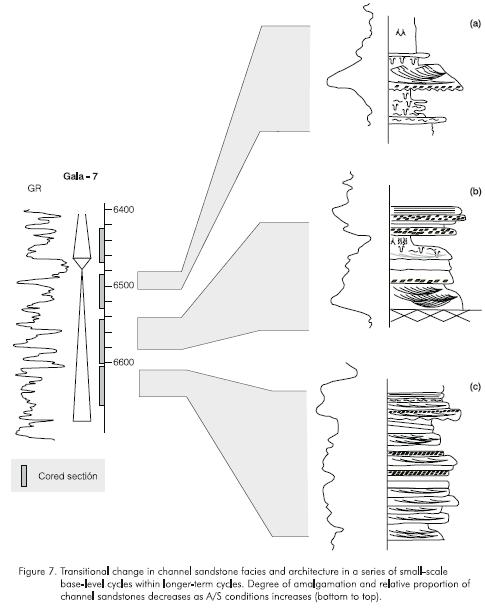
The parallel changes of facies successions and diversity and channel sandstone architecture can be used to predict sandstone geometry and architecture by using stacking pattern analysis in core-calibrated well logs. This is called stacking pattern analysis.
Predicting position, continuity and areal extent of reservoir units within stratigraphic cycles
Stacking pattern analysis is a method for predicting sandstone geometry and position, facies tract continuity and stratigraphic architecture in core-calibrated well logs. Genetic sequences containing shoreface and continental strata provide an easily understood illustration of stacking pattern analysis. In a series of genetic sequences containing shallowing-up shoreface facies successions, the proportions of shallower and deeper facies within one genetic sequence are compared with the proportions of the same facies within the overlying sequence. If the younger sequence has a greater proportion of shallower facies and a lesser proportion of deeper facies, the younger shoreface is displaced seaward with respect to the underlying unit (Figure 8 top). Conversely, if the proportion of deeper facies is greater in the younger sequence than in the older sequence, then the younger shoreface is displaced landward compared with the underlying sequence (Figure 8 bottom).
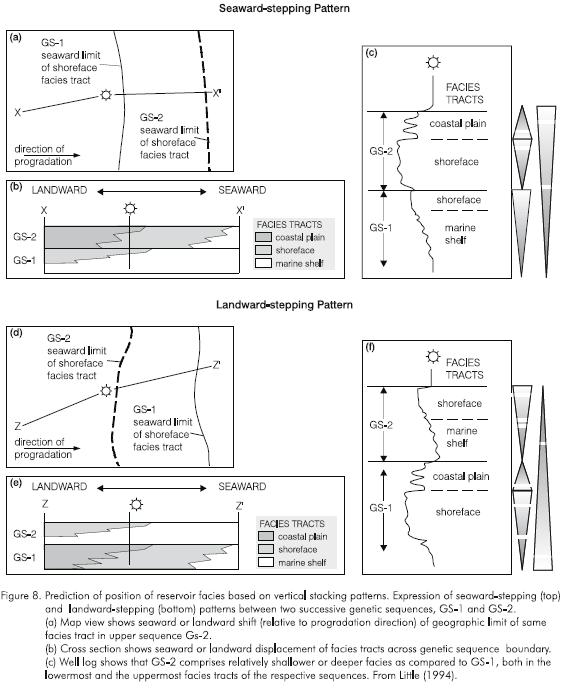
The ability to predict the geographic position of the reservoir facies by stacking pattern analysis has direct impact on development strategy. In this example, if the upper-shoreface sandstone is oil saturated, the next development well will be located seaward if the stacking pattern is seaward stepping (Figure 8b). It will be located landward if the stacking pattern is landward stepping (Figure 8e).
As in shoreface strata, changes in vertical facies successions and proportions can be used to predict position, continuity and areal extent of fluvial reservoir sandstones. Stratigraphic attributes, such as degree of sandstone connectivity, that influence fluid flow in reservoirs may be interpolated between and extrapolated beyond control points through analysis of large-scale stratal architecture and geometry and small-scale sedimentologic attributes. The direct relationship between small-scale sedimentologic (e.g. facies compositions and proportions) and large-scale stratigraphic attributes (e.g. areal extent and continuity) is very powerful for prediction purposes.
In low A/S conditions the greater degree of cannibalism and amalgamation among individual storeys tend to form laterally amalgamated, interconnected, sheetlike sandstone bodies (Figure 9 middle section). These sandstones have low facies diversity, homoge-neous vertical grain-size profiles, and are composed predominantly of amalgamated trough-cross stratified sandstone within large-scale scour and fill macroforms (Figures 6e, 6f).
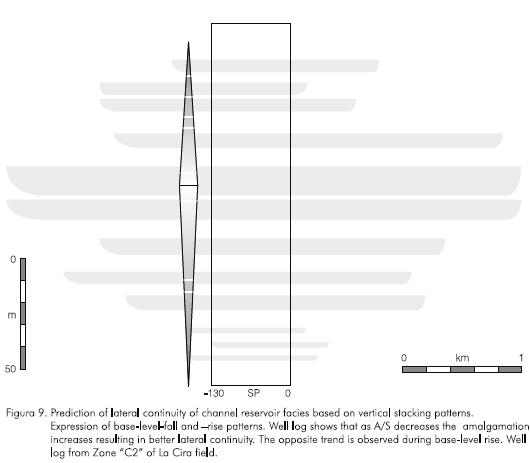
In higher A/S regimes, individual storeys are preserved as isolated sandstone bodies which have reduced areal continuity and connectivity (Figure 9 upper and lower sections). These single-storey channel sandstones are characterized by high facies diversity, complete fining-up profiles of channel sandbodies, and usually occur with floodplain deposits (Figures 6b, 6c).
Summarizing, a vertical trend of increasing facies diversity, grain size heterogeneity and channel sandstone proportion indicate a decrease in the lateral continuity of channel sandstones. This allows prediction of reservoir continuity and compartmentalization from analysis of well logs.
PETROPHYSICAL PROPERTIES
Previous work
Petrophysical properties of sandstones have been studied for years from sedimentologic and engineering perspectives (Darcy, 1856; Muskat, 1937; Levorsen, 1966; among others). These studies have concentrated on identifying relations among petrophysical properties, textural and fabric attributes, and facies.
Several studies have established relationships among porosity, permeability and texture. Grain size, shape and sorting have a strong influence on porosity and permeability (Krumbein and Monk, 1942; Gaithor, 1953; Rogers and Head, 1961; and Pryor, 1973). Theoretically, porosity is independent of grain size for uniformly packed spheres of uniform size (Rogers and Head, 1961). In practice, however, coarser sands tend to have higher porosity than finer sands (Sneider et al., 1977), probably reflecting variations in sorting and nonspherical grain shape. Permeability declines with decreasing grain size because pore and throat diameters decrease and capillary pressure increases (Krumbein and Monk, 1942; Dodge et al., 1971). Porosity and permeability increase with improved sorting (Fraser, 1935; Rogers and Head, 1961; Dodge et al., 1971; Beard and Weyl, 1973; Pryor, 1973). Better sorted sands have less fine matrix which tends to fill and clog pores and pore throats, thus reducing porosity and permeability. Data on the effect of grain shape (roundness and sphericity) are sparse. Fraser (1935) inferred that porosity might decrease with sphericity because spherical grains may pack more closely than irregularly shaped particles.
It is widely recognized that grain size and sorting variations are closely associated with facies changes. Several studies showed regular variations of porosity and permeability within successions of different facies (Chapin, 1991; Hurst, 1991; Jackson, et al., 1991; Jacobsen and Rendall, 1991). In a fining-up meandering facies succession, trough-cross stratified sandstones have the highest porosity and permeability values. Overlying small-scale trough-cross-stratified sandstones, and ripple-and wispy-laminated sandstones have progressively reduced porosity and permeability values (Figure 10). Also, identical facies from different geomorphic elements -for example ripple-laminated or trough-cross-stratified sandstone facies from channel or crevasse splay sandstones -may have different porosity and permeability. Generally, crevasse splay sandstones have lower porosity and permeability than fluvial channel sandstones of the same facies.
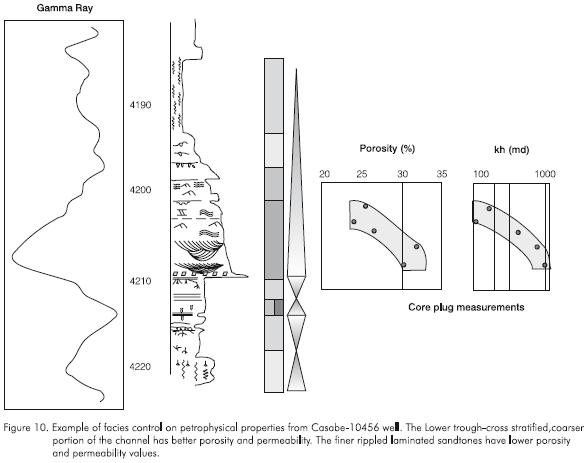
Besides these known changes in porosity and permeability values among different facies, porosity and permeability values change regularly within identical facies as a function of stratigraphic position. Kusumanegara (1994) and Fajardo (1995) showed that at the reservoir scale petrophysical attributes of fluvial channel sandstones are stratigraphically sensitive, as are most other attributes of sedimentary facies.
Porosity and permeability variations of facies as a function of stratigraphic position
Changes in petrophysical properties with respect to stratigraphic position are parallel to changes in degree of preservation of identical facies deposited under variable A/S conditions. Regular, stratigraphically controlled changes in petrophysical properties and other physical sedimentologic attributes occur in constant facies within a genetic sequence and from one genetic sequence to the next.
In fluvial trough cross-stratified sandstones, porosity and permeability changes are exactly coincident with subtle variations in sedimentary facies attributes which change as a function of stratigraphic position. In the case of fluvial strata deposited during an increase in A/S conditions, porosity and permeability are highest in trough cross-stratified sandstones immediately above channel scour bases, and decrease upward to the top of the sandstone. In channel sandstones composed of cut-and-fill macroforms, porosity and permeability decrease from the base to the top of each macroform, and generally from one macroform to the next in stratigraphic succession. Successive channel sandstones within the same stratigraphic sequence and channel sandstones from one sequence to the next one have progressively lower porosity and permeability values in a long-term increase in A/S conditions (Figures 11 and 12). These stratigraphically sensitive changes in porosity and permeability are coincident with reduced amalga-mation and cannibalism of the original dunes that formed the trough cross-stratification, as reflected by increasing set thickness of cross-stratification at the macroform and channel belt scales.
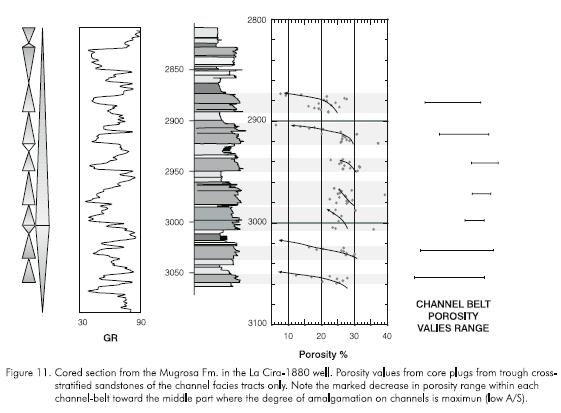
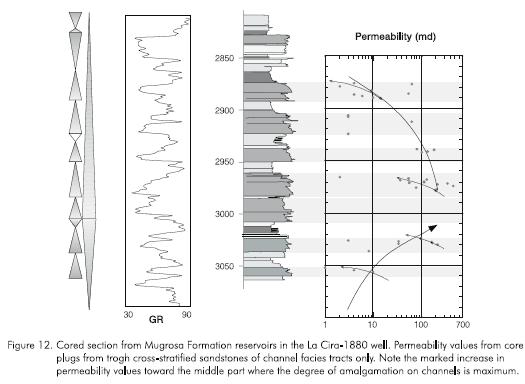
At even higher A/S conditions, decreased cannibalism of previously deposited channel and floodplain deposits, as well as decreased amalgamation of sediments accumulating in the active channelbelt, result in increased facies diversity, decreased channel sandstone continuity, and better preservation of finer grain components (worst sorting). Because the upper parts of channels are better preserved, a higher proportion of lower energy ripple-and wispy-laminated sandstones are preserved and thus the porosity and permeability profiles would strongly decrease upward. This, in turn, results in more heterogeneous reservoirs. Also, in high A/S conditions, mud drapes are more common and more continuous and have a higher chance of functioning as fluid-flow barriers.
Figures 11 and 12 illustrate regular changes in porosity and permeability in several channel trough-cross-stratified sandstone reservoirs though 270' (82 m) of continuous cored section. There is a gradual increase in abundance of channel sandstones from 3.070' to 2.980'. This increase in abundance is accompanied by a decrease in spacing between channel sandstones and a decrease in abundance of wet floodplain and lake facies associations. From 2.980' to 2800', a reversal of this tendency is apparent from the concomitant increase in crevasse splay and soil facies associations and the decreased thickness and frequency of channel sandstone facies associations. The gradual changes in the facies associations and successions within successive small-scale cycles are paralleled by changes in porosity and permeability values. In the interval 3.070' to 2980' there is a reduced dispersion of porosity values within each channel unit. Whereas the trough-cross stratified sandstones at 3.060'-3.052' show decreasing-up porosity values (25% to 13%), the trough-cross stratified sandstones in the 3010"-2960' interval exhibit less variability and no clear trend in porosity change. The mean value for the lower channel sandstone is about 20% whereas the mean value in the upper sandstone is about 25%. From 3.000'-2.876' an opposite tendency is apparent. An increase in dispersion of porosity values and a decrease in the mean porosity in each channel sandstone is observed (Figure 11). At lower A/S conditions (3.000' -2.950') the higher cannibalism among channel storeys removed the upper parts of the channel sediments.
Permeabilities of trough-cross-stratified channel sandstones (Figure 12) show similar variations with respect to stratigraphic position. From 3.060' to 2.970' there is a marked increase in the permeability ranges for each channel unit, from 1 -10 md to 36 -500 md. From 2.970' to 2.870', this tendency is reversed and permeability ranges decrease to 2 -20 md range (Figure 12). The greater reworking of sediments in the multistorey channels at lower A/S conditions causes better sorting and removal of the finer fractions. Better sorted sands have larger and more homogeneous pores and pore throats and possess higher permeabilities. As A/S conditions increase, progressively more of the different facies and grain size populations originally present in the geomorphic environment are preserved in strata. This reduces the pore size and increases pore size heterogeneity, decreasing porosity and permeability values.
Figure 13 shows the changes in porosity and permeability of stacked channel sandstones which accumulated in lower A/S conditions than those shown in Figures 11 and 12. This 90 ft (30 m) thick, sandstonerich section shows parallel changes in preserved channel sandbodies and petrophysical properties. In the lower and upper part of the cored interval channel sandstones are well preserved, exhibit conspicuous fining-up profiles, and are embedded in floodplain and lake mudstones. By contrast, the middle portion of the cored interval contains only amalgamated troughcross-stratified sandstones, probably within cut-and-fill macroforms. The cored interval is interpreted as a symmetrical cycle, with the fall-to-rise turnaround (minimum A/S) in the middle part of the core. Mean porosity values are about 3% higher in the middle, lowest A/S section; permeability is one order of magnitude higher than in the upper and lower channel sandstones which accumulated in higher A/S conditions.
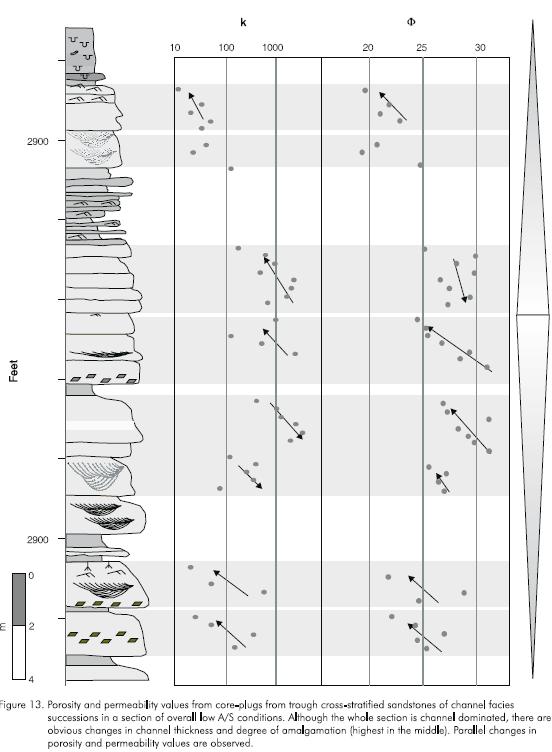
Petrophysical attributes of facies tracts representing other environments also are stratigraphically sensitive or stratigraphically controlled, and co-vary with physical sedimentologic attributes. Figure 14 shows the vertical distribution of porosity, permeability and oil saturation for an approximately 90 ft (30 m) cored section of crevasse splay complexes from the La Cira-1880 well. This section was deposited during a longterm decrease in A/S, or base-level-fall hemi-cycle, as evidenced by the coarser, thicker, more amalgamated character of the upper crevasse splay complex. Porosity, permeability and oil saturation of trough-cross and massive sandstone facies increase upward within each coarsening-up crevasse-splay, as expected from the upward increase in grain size and sand content. Besides this "facies controlled" petrophysical properties, there is an overall increase in porosity and permeability and oil saturation values toward the top of the core, parallel to the decrease in A/S ratio.
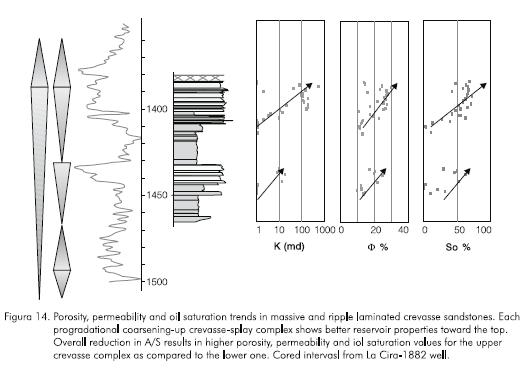
These unidirectional changes in petrophysical properties of constant facies within stratigraphic cycles are present in all wells analyzed. When identical facies within the same stratigraphic cycle are analyzed in several geographic locations the absolute values and ranges of these parameters vary laterally, but the increasing or decreasing trend is the same in every location. Figure 15 shows histograms of porosity averages of trough-cross-stratified channel sandstones toward the base and the top of a long-term base-level-rise hemi-cycle. A1 and A2 sandstones are reservoir zones defined by the field engineers. Porosity values shown in Figure 15 are averages of all trough-cross-stratified channel sandstones within each zone. Regardless of the differences in porosity values and ranges in every well a reduction in porosity is apparent in this base-level rise cycle (higher A/S conditions toward the top).
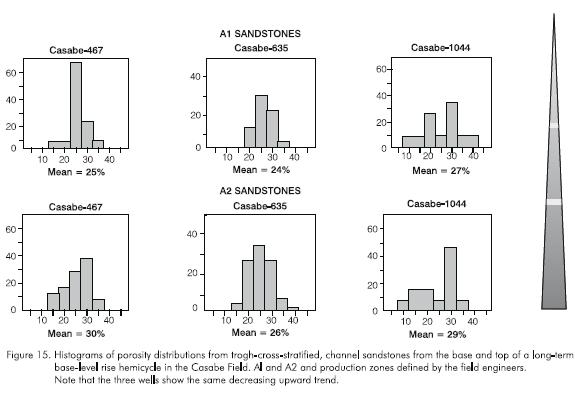
CAPILLARY PRESSURE
Another property normally measured for reservoir characterization is capillary pressure. Capillary pressure is the pressure difference across the interface between two immiscible fluids, one of which wets the surface of a solid. Capillary pressure is illustrated by the common physics experiment of introducing a thin tube into water; the water rises in the tube above the water level. The thinner the tube, the higher the water rises. This phenomenon is the result of the water wetting the tube. Water rises until the weight of the water column balances the wetting force of surface tension.
Capillary pressure in porous rocks depends on wettability, fluid saturations and pore and throat sizes. Capillary pressure increases as pore and throat diameters decrease, other conditions staying constant. The smaller the grain size and the higher the mud content, the higher the capillary pressure. Capillary pressure generally is proportional to permeability.
Air-water capillary pressure curves are measured from core-plugs to estimate irreducible water saturation and reservoir quality. Jennings (1987) showed that the shape of capillary pressure curves can be used to determine pore-size distribution and thickness of the transition zone (between 100% water -to 100% oil-saturated rock) (Figure 16).
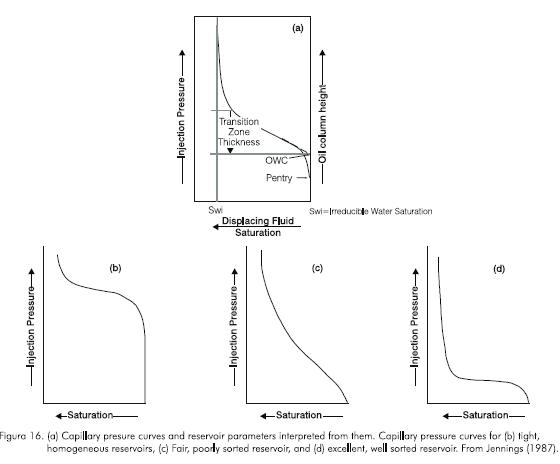
As with other reservoir rock properties, capillary pressure measurements normally are analyzed with respect to facies or environment. An example of such comparisons between different facies of different environments is shown in Figure 17 from the Gala-7 well. Trough cross-stratified channel sandstones and ripplelaminated crevasse splay sandstones have very different capillary pressure curves, as expected from the different environments and grain size distributions. Although crevasse-splay and channel sandstones have the same source of sediment, crevasse-splay deposits contain more fine sand and mud. The higher mud content in crevasse-splay sandstones reduces pore and pore throat sizes, and increases the capillary pressure (Figure 17).
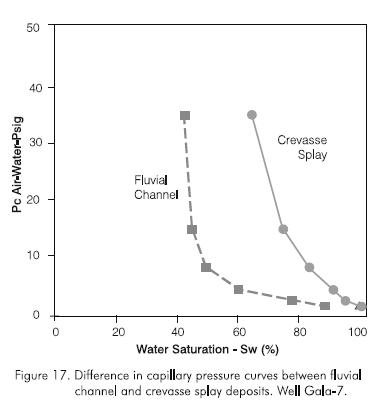
Besides these facies-dependent changes in capillary pressure, A/S conditions also control capillary pressure values of identical facies, similar to the changes observed with porosity and permeability. In fluvial systems, more reworking and cannibalism during low A/S conditions increases grain sorting, eliminates fines, and produces more homogeneous pore throat and pore distributions, higher permeabilities and lower capillary pressures. At higher A/S conditions more geomorphic elements of channels and floodplains are preserved, increasing facies diversity and grain-size distributions. These cause wider porosity ranges, lower permeabilities, and higher capillary pressures.
Figures 18 and 19 show capillary pressure curves for fluvial channel and crevasse splay sandstones deposited in high and low A/S conditions. Both facies associations show an increase in capillary pressure and a decrease in reservoir quality with increasing A/S conditions. Decrease in reservoir quality is moderate in the case of channel sandstones (Figure 18). Crevasse sandstones have a stronger change in capillary pressure. The drastic decrease in reservoir quality is the result of a marked increase in the proportion of silt and clay in the crevasse sandstones. In lower A/S conditions, reworking of crevasse deposits remove fines and substantially increase permeability. Reduced reworking during high A/S conditions result in better preservation of original geomorphic elements and grain size populations. Distal portions of crevasse complexes rich in clay and silt have much lower permeabilities and higher capillary pressures.
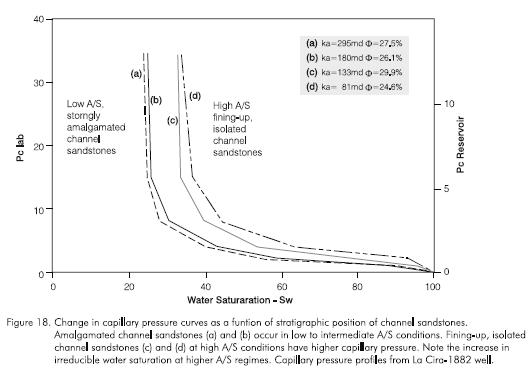
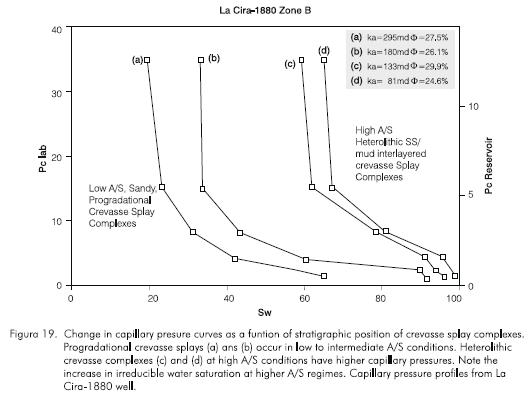
OIL PRODUCTION AND DRIVE MECHANISM
Changes in the areal extent, continuity and petrophysical properties of channel sandstones with respect to stratigraphic position influence oil production decline rate, ultimate oil recovery and efficiency of drive mechanisms.
Low accommodation channel sandstones have greater lateral and vertical interconnection and are more homogeneous. Detailed correlations within oil fields in the study area (e.g. Galan and Lisama) show that they also occupy larger areas and have higher net sand content. Composite sandstone bodies can occupy areas 4*2 km. Associated mudstones are thinner and discontinuous. In combination, these attributes produce larger reservoir compartments and reduce the production decline rate (Figure 20). According to Davies and Bernal (1995) these sandstones in the Lisama field can have high cumulative production (0,5 to > 2 MMBO) from individual wells.
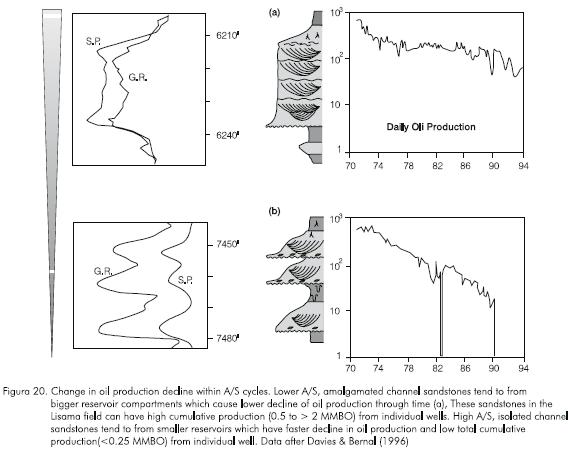
High A/S channel sandstones have less lateral and vertical interconnection because they occur as isolated sandstone bodies embedded in thicker and areally continuous floodplain mudstones. These fully preserved channel sandstones are more heterogeneous and havelower sandstone-to-mudstone ratios. Correlations show that these sandstones have a wide range of lateral continuity. Composite sandstone bodies can be correlated between 100s of meters apart up to 1,5 km. Reservoir compartments are smaller and oil production rates decline faster (Figure 20). Davies and Bernal (1995) reported low total cumulative production (< 0,25 MMBO) from individual wells in the Lisama field.
Solution gas and water encroachment are the most common drive mechanisms in the Middle Magdalena Valley fields. The relative importance and efficiency of each mechanism change with stratigraphic position and is dependent on reservoir size. Because channel sandstones deposited under low A/S conditions generally are areally extensive, have a higher probability of being connected to regional aquifers. Amalgamated, interconnected sandstone bodies in the northern portion of the Lisama field have water recharge. Some laterally-interconnected channel sandstones in the La Cira field are water bearing and the water chemistry indicates meteoric origin (Dickey, 1992). By contrast, isolated, ribbon channel-belt sandstones of low A/S conditions show that the solution gas is the main drive mechanism (Davies and Bernal, 1995).
SUMMARY AND IMPLICATIONS FOR RESERVOIR CHARACTERIZATION AND FLOW SIMULATION
Current engineering and geological approaches to characterize reservoirs and define fluid flow units (Amaefule, et al., 1994; Weber and Van Geuss, 1990; Jennings, 1987) utilize the perspective of facies and environments. Stratigraphic controls on variations in facies attributes, components and proportions, and therefore reservoir properties, are not recognized.
Reservoir heterogeneity is a very important factor in production. Of the seven types of reservoir heterogeneities described by Weber (1986; Figure 21), three or four change with A/S conditions. These are the geometry and size of genetic units, permeability zonation and continuity of bafles within genetic units, and diversity of sedimentary structures. Well data show that within stratigraphic cycles tens to hundreds of meters thick, sandstone connectivity and compartmentalization change dramatically as a funciton of A/S conditions (Figure 22). Channel sandstones deposited in high A/S conditions tend to be isolated, more discontinuous and associated with thick floodplain and lake mudstones (Figure 22a, top and bottom). At lower A/S conditions, amalgamation increases and interconnection between channelbelt sandstones increases substantially (Figure 22a, middle). Such changes in stra-tigraphic architecture and reservoir properties should be utilized in the design of simulation grids (Figure 22b). At higher A/S positions, cells must be smaller and thinner to account for the strong compartmentalization and abundant fluid-flow barriers. By contrast, at lower A/S conditions cells may be larger due to the more homogeneous character of the reservoir and the lower degree of compartmentalization.
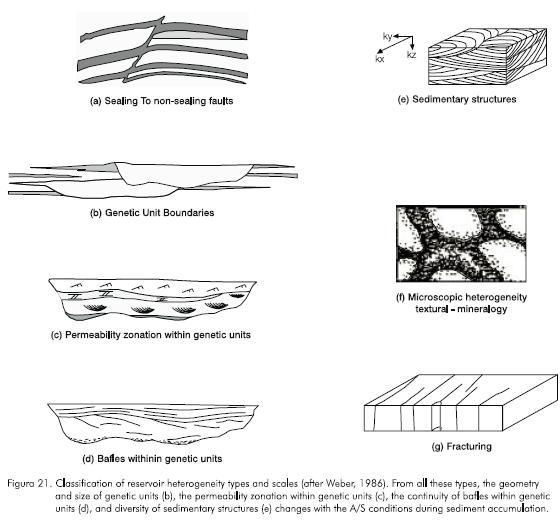
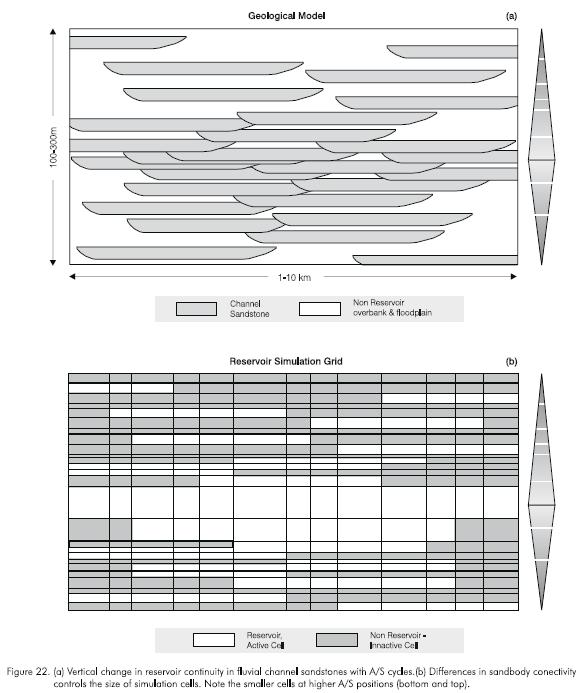
Smaller scale, internal heterogeneities such as permeability zonation and baffle geometry and continuity (Figure 21 cand 21d) also change with changing A/S conditions. Increased amalgamation of low A/S channel sandstones reduces facies diversity and permeability baffles, and generates more homogeneous porosity and permeability distributions (Figure 23a). This causes less tortuous fluid-flow paths. High A/S channel sandstones contain a larger proportion of original geomorphic elements resulting in increased facies diversity and internal heterogeneity, poorer sorting, and increased range in porosity and permeability (Figure 23b). Fluid flow follows more tortuous paths, and oil sweep is less efficient due to high permeability contrasts and more abundant permeability baffles.
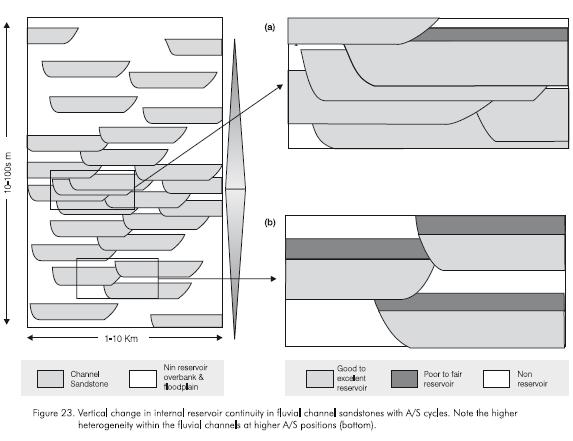
In summary, many attributes of strata important for reservoir production and management are stratigraphically sensitive. Variations in stratigraphic and sedimentologic attributes as a function of stratigraphic position should be incorporated in reservoir assessment and set up for flow simulations. When petrophysical data are plotted in a stratigraphic context, and not just with respect to facies, porosity and permeability values have significantly reduced scatter. Regular changes in petrophysical attributes with respect to stratigraphic position can be incorporated into statistical analyses, such as stratigraphically changing variograms. Since changes in petrophysical attributes are regular and predictable, the cost of laboratory analysis can be reduced by a priori definition of the best places to take and measure samples. Prediction of laterally continuous versus isolated reservoirs and of reservoir heterogeneity will allow to better design waterflood programs and reservoir simulation models.
CONCLUSIONS
- Development of oil and gas fields requires an accurate geological description of reservoir characteristics, especially geometry, size, heterogeneity, and porosity and permeability distribution. This is achieved through an understanding of the depositional environments and the A/S conditions during sediment accumulation. These two factors combined control the shape, size, trend, lateral continuity and quality of sandstone bodies. The resulting reservoir geological model provides the optimum framework for reservoir subdivision, correlation and mapping, estimating reserves and defining simulation models and enhanced recovery programs.
- Sedimentological and stratigraphical studies of outcrop and more than 6.000 ft of core from three big oil fields in the Middle Magdalena basin demonstrate parallel, unidirectional changes in geometry, compartmentalization, and petrophysical properties (porosity, permeability and capillary pressure) of channel and crevasse sandstones. Channelbelt sandstones deposited under low A/S conditions are laterally more continuous and interconnected, forming bigger reservoir compartments that have higher and more homogeneous porosity and permeability. They exhibit lower production decline rates and their dominant drive mechanism is water encroachment. Isolated, laterally discontinuous channelbelt sandstones deposited under high A/S conditions form smaller reservoir compartments that have lower and wider range of porosity and permeability. They exhibit higher decline oil production rates and lower cumulative hydrocarbon production. Solution gas is the dominant drive mechanism.
- These changes in reservoir properties within longterm changes in A/S conditions are parallel to changes in sedimentological and stratigraphic attributes that can be interpreted from cores and calibrated well logs. Thus, prediction of lateral continuity and compartmentalization of channel sandstones and trends in petrophysical properties can be achieved from stratigraphic analysis of well logs and cores.
ACKNOWLEDGMENTS
This research is part of Ramón's Ph.D. project and was supported by grants from Colfuturo, Ecopetrol, and CSM foundation. We received helpful suggestions from Ramona Graves (CSM) and the editorial committee of CT&F. Johan Kusumanegara and Andres Fajardo's results inspired this research. This paper benefited from their input. Special thanks to Maria Helena Mogollón and Claudia Malagón for their help with petrophysical data. We are grateful to Ecopetrol for access to data and permission to publish.
REFERENCES
Allen, J. R. L., 1978. "Studies in fluviatile sedimentation: bars, bar complexes and sandstone sheets (low sinuosity braided streams) in the Brownstones (L.Dev.), Welsh Borders", Sediment. Geol., 26: 281 -293. [ Links ]
Allen. J. R. L. 1979. "Studies in fluviatile sedimentation: an exploratory quantitative model for the architecture of avulsion-controlled alluvial suites", Sediment. Geol., 21: 129 -147. [ Links ]
Amaefule, J. O., Altunbay, M., Ohen, H., Kersey, D. G. and LANE, P., 1994. "A hydraulic (flow) unit-based approach for predicting formation damage profiles in uncored intervals/wells using core/log data", SPE 27365. [ Links ]
Beard, D. C. and Weyl, P. K., 1973. "The influence of texture on porosity and permeability of unconsolidated sand", AAPG Bull., 57: 349 -369. [ Links ]
Blakey, R. C., and Gubitosa, R., 1984. Controls of sandstone body geometry and architecture in the Chinle Formation (Upper Triassic), Colorado Plateau, Sediment. Geol., 38: 51 -86. [ Links ]
Bridge, J. S. and Leeder, M. R., 1979. "A simulation model of alluvial stratigraphy", Sedimentology, 26: 617 -644. [ Links ]
Chapin, M. A., 1989. "Quantification of multiscale rock property variations in fluvial systems for petroleum reservoir characterization", Unpublished M.Sc., Thesis, Colorado School of Mines, Golden. [ Links ]
Cross, T. A., Baker, M. R., Chapin, M. A., Clark, M. S., Gardner, M. H., Hanson, M. S., Lessenger, M. A., Little, L. D., McDonough, K. J., Sonnenfeld, M. D., Valasek, D. W., Williams, M. R., and Witter, D. N., 1993. "Applications of High-Resolution Sequence Stratigraphy to Reservoir Analysis", in Eschard, R. and Doligez, B., eds., Subsurface Reservoir Characterization from Outcrop Observations, Proceedings of the 7th Exploration and Production Research Conference, Paris, Teichnip: 11 -33. [ Links ]
Darcy, H., 1856. Les fountaines publiques de la Ville de Dijon, Paris, V.Dalmont, 674 p. [ Links ]
Davies, D. K. I., Williams, B. P. J. and Vessel, R. K., 1992. "Models for meandering and braided fluvial reservoirs with examples from the Travis Peak Formation, East Texas", SPE Paper 24692, 67th Annual Technical Conf., Washington, D. C.: 321 -329. [ Links ]
Davies, D. K. I., and Bernal, M. C., 1995. "Influence of channel style on reservoir development and production characteristics: Oligocene sandstones of the Lisama Area, Magdalena Valley, Colombia", VI Petroleum Colombian Congress, Bogotá (Oct. 3 -6). [ Links ]
Dikey, P., 1992. "La Cira-Infantas Field-Colombia, Middle Magdalena Basin", in Structural Traps VII (Beaumont E. A. and Foster N. H., eds.), AAPG Treatise of Petroleum Geology, Atlas of Oil and Gas Fields: 323 -347. [ Links ]
Dodge, C. F., Holler, D. P., and Meyer, R. L., 1971. Reservoir heterogeneities of some Cretaceous sandstones, AAPG Bull., 55 (10): 1814 -1828. [ Links ]
Fajardo, A. A., 1995. "4-D stratigraphic architecture and 3-D reservoir fluid flow model of the Mirador Fm., Cusiana field, Foothills area in the Cordillera Oriental, Colombia", Unpublished M.Sc., Thesis, Colorado School of Mines, Golden. [ Links ]
Fraser, H. J., 1935. "Experimental study of the porosity and permeability of clastic sediments", J. Geol., 43: 910 -1010. [ Links ]
Friend, P. F., 1983. "Towards the field classification of alluvial architecture of sequence", Modern and Ancient Fluvial Systems (Ed. by J. D. Collinson & J. Lewin), Spec. Publs int, Ass. Sediment., 6: 345 -354. [ Links ]
Gaithor, A., 1953. "A study of porosity and grain relationships in sand", J. Sedim. Petrol., 23: 186 -195. [ Links ]
Gardner, M. H., 1993. "Sequence stratigraphy of Upper Cretaceous Ferron Sandstone Member of the Mancos Shale, East-Central Utah", Unpublished Ph.D. Dissertation, Colorado School of Mines, Golden, 528 p. [ Links ]
Hanson, M. S., 1989. "Utilization of genetic sequence analysis in the prediction of Eagle sandstone reservoir facies distribution, Montana", Unpublished M.Sc. Thesis, Colorado School of Mines, Golden, Colorado, 150 p. [ Links ]
Harris, D. G., 1975. "The role of geology in reservoir simulation studies", J. Petrol. Techn. (May): 625 -632. [ Links ]
Hurst, A. and Rosvoll, K. J., 1991. "Permeability variations in sandstones and their relationship to sedimentary structures", in Lake, L., Carroll, H., Jr., and Wesson, T., eds., Reservoir characterization II, Academic Press, Inc: 166 -196. [ Links ]
Jackson, S. R., Tomutsa, L., Szpakiewick, M., Chang, M. M., Honarpour, M. M. and Schatzinger, R. A., 1991. "Construction of a reservoir model by integrating geological and engineering information -Bell Creek Field, A barrier/ strandplain reservoir", in Lake, L., Carroll, H., Jr., and Wesson, T., eds., Reservoir characterization II, Academic Press, Inc.: 524 -556. [ Links ]
Jacobsen, T. and Rendall, H., 1991. "Permeability patterns in some fluvial sandstones. An outcrop study from Yorkshire, North East England", in Lake, L., Carroll, H., Jr., and Wesson, T., eds., Reservoir characterization II, Academic Press, Inc.: 315 -338. [ Links ]
Jennings, J. B., 1987. "Capillary pressure techniques: Application to exploration and development geology", AAPG Bull. (10): 1196 -1209. [ Links ]
Krumbein, W. C. and Monk, G. D., 1942. "Permeability as a function of the size parameters of unconsolidated sands", Am. Inst. Min. and Metal. Eng., Tech. Pub. 1492: 1 -11. [ Links ]
Kusumanegara, J., 1994. "Stratigraphic controls on petrophysical attributes and fluid flow pathways in an exhumed fluvial reservoir, Sunnyside quarry, Carbon Country, Utah", Unpublished M.Sc., Thesis, Colorado School of Mines, Golden. [ Links ]
Le Blanc, R. J., 1972. "Geometry of sandstone reservoir bodies", AAPG Memoir, 18: 133 -190. [ Links ]
Levorsen, A. I., 1966. "The geology of petroleum", Oxford: Freeman, 724 p. [ Links ]
Little, L. D., 1994. "Genetic stratigraphic analysis of the Upper Cretaceous Eagle sandstone, Bearpaw Mountains area, North-Central Montana", Unpublished M.Sc., thesis, Colorado School of Mines. [ Links ]
Mattax, C. C. and Dalton, R. L., 1990. "Reservoir Simulation", SPE Monograph, 13. [ Links ]
Melvin, J., 1993. "Evolving fluvial style in the Kekiktuk Formation (Mississippian), Endicott field area, Alaska: base level response to contemporaneous tectonism", AAPG Bull, 77: 1723 -1744. [ Links ]
Miller, D. D., Mcpherson, J. G., Covington, T. E., 1990. "Fluviodeltaic reservoir, South Belridge field, San Joaquin Valley, California", in Barwis JH, McPherson JG, Studlick RJ (eds), Sandstone petroleum reservoirs, Springer, Berlin Heidelberg, New York: 109 -130. [ Links ]
Miall, A. D. 1996. The geology of fluvial deposits: Sedimentary facies, basin analysis and petroleum geology, Springer-Verlag, New York, 582 p. [ Links ]
Muskat, M., 1937. Flow of homogeneous fluids through porous media, New York, McGraw-Hill, 763 p. [ Links ]
Olsen, T., Steel, R., Hogseth, K., Skar, T. and Roe, S. L., 1995. "Sequential architecture in a fluvial succession: sequence stratigraphy in the Upper Cretaceous Mesa Verde Group, Price Canyon, Utah", JSR, B65, (2): 265 -280. [ Links ]
Pryor, W. A., 1973. Permeability-porosity patterns and variations in some Holocene sand bodies, AAPG Bull., 57: 162 -189. [ Links ]
Puigdefabregas, C., and VanVliet, A., 1978. "Meandering stream deposits from the Tertiary of the Southern Pyrenees", in Miall, AD (ed.) Fluvial sedimentology, Can. Soc. Petrol. Geol. Mem, 5: 469 -485. [ Links ]
Rogers, J. J. and Head, W. B., 1961. "Relationship between porosity, mean size and sorting coefficients of synthetic sands", J. Sed. Petrol., 31: 467 -470. [ Links ]
Sanchez-Moya, Y., Sopeña, A., and Ramos, A., 1996. "Infill architecture of a nonmarine half-graben Triassic basin (Central Spain)", JSR, 66 (6): 1122 -1136. [ Links ]
Shelton, J. W., 1973. "Models of sand and sandstone deposits: a methodology for determining sand genesis and trend", Bull. Oklah. Geol. Surv., 118: 122 p. [ Links ]
Schumm, S. A., 1977. The fluvial system, Wiley, New York. [ Links ]
Sneider, R. M., Richardson, F. H., Paynter, D. D., Eddy, R. E., and Wyant, I. A., 1977. "Predicting reservoir rock geometry and continuity in Pennsylvanian reservoirs, Elk City field, Oklahoma", J. Petrol. Tech., 29: 851-866. [ Links ]
Sonnenfeld, M. D. and Cross, T. A., 1993. "Volumetric Partitioning and facies differentiation within the Permian Upper San Andres Formation of Last Chance Canyon, Guadalupe Mountains, New Mexico", in Loucks R. G., and Sarg, J. F., eds., Recent advances and applications of carbonate sequence stratigraphy, AAPG Memoir, 93: 435 -474. [ Links ]
Walker, R. G.(ed.), 1979. Facies Models, Geol. Assoc. of Canada, St. John's, Newfoundland. Geosci. Can. Reprint Ser. 1. [ Links ]
Warren, J. E. and Price, H. S., 1961. "Flow in heterogeneous porous media", AIME. Trans, 222, SPEJ (Sept): 153 -169. [ Links ]
Weber, K. J., 1986. "How heterogeneity affects oil recovery", in Lake, L.W. and Carrol H.B. Jr. (eds), Reservoir characterization, academic press Inc., Orlando: 487 -544. [ Links ]
Weber, K. J. and Van Geuss, L. C., 1990. "Framework for constructing clastic reservoir simulation models", JPT (October). [ Links ]
Willis, B. J., 1993. "Evolution of Miocene fluvial systems in the Himalayan foredeep through a two-kilometer-thick succession in northern Pakistan", Sed. Geol., 88: 77 -121. [ Links ]