Services on Demand
Journal
Article
Indicators
-
Cited by SciELO
-
Access statistics
Related links
-
Cited by Google
-
Similars in SciELO
-
Similars in Google
Share
CT&F - Ciencia, Tecnología y Futuro
Print version ISSN 0122-5383On-line version ISSN 2382-4581
C.T.F Cienc. Tecnol. Futuro vol.1 no.5 Bucaramanga Jan./Dec. 1999
DESIGN AND EVALUATION OF OXYGEN TRANSFERENCE PROCESSES IN WASTEWATER TREATMENT
Abstract
Aprocess of air injection to bodies of water was developed and evaluated. The system is composed of ejectors operated by pressurized water (where atmospheric air is drawn in), a network of contact and distribution pipelines (tubing), and a dispersion system. The influence and importance of each of these parts, especially the ejectors, was studied in order to create a model system that was compared with the most common types of aerators (oxygenators) used in industrial processes for water treatment. The energy efficiency (expressed as kilograms of oxygen transferred per kilowatt/ hour consumed) is used as the unit of comparison of the different processes studied. Furthermore, the comparative advantages of each of the systems were evaluated, namely maintenance, service factor, operation and installation viability, homogeneity, operational stability, adaptability to changing operating conditions among others. It was found that the appropriate industrial installation of an aeration system like the one proposed here, can generate over 30% in energy savings and a 90% reduction in maintenance costs among some of its major advantages.
Keywords: water treatment, wastewater, aeration, oxygen exchange.
Resumen
Se desarrolló y evaluó un proceso de inyección de aire a cuerpos de agua, el sistema esta compuestos por eyectores operados por agua a presión (en donde se succiona el aire atmosférico), la red de tubería de contacto y distribución y el sistema de dispersión. La influencia y la importancia de cada una de estas partes, en especial los eyectores, se estudió con el fin de lograr un sistema modelo que fue comparado con los principales tipos de aireadores usados en procesos industriales de tratamiento de agua. Se usó la eficiencia energética, expresada en kilogramos de oxígeno transferido por kilovatio-hora consumido, como unidad de comparación de los diferentes procesos estudiados. También se evaluarón las ventajas comparativas de cada uno de los sistemas como mantenibilidad, factor de servicio, facilidad de operación, facilidad de instalación, homogeneidad, estabilidad operacional, adaptabilidad a cambios de condiciones de operación entre otras. Se encontró que la adecuada instalación industrial de un sistema de aireación como el propuesto puede generar ahorros de energía de más del 30% y reducción de costos de mantenimiento del 90% entre sus principales ventajas.
Palabras clave: tratamiento de agua, aguas residuales, aireación, intercambio de oxígeno.
Introduction
The quality of industrial wastewater is a factor that directly affects the environment, particularly water resources. This factor is evaluated by means of standards that regulate amongst others, the content of inorganic substances such as iron, manganese, phosphorous and nitrogen, volatile substances like hydrogen sulfide, carbon anhydride that affect the taste and the smell of water, suspended solids, biological oxygen demand (BOD), chemical oxygen demand (COD), and some organic compounds like hydrocarbons in suspension and volatile organic compounds (VOC)
There are two main types of treatments for these waters; the anaerobic type used mainly in water with a high organic load, and the aerobic type used in large water volume and in relatively low contaminant concentrations. The latter has the main advantages of ease of operation and a low environmental impact because it does not generate unpleasant odors or intermediate contaminant products. Among the operations that include the industrial waste water aerobic treatment is the aeration process, which transfers oxygen to the waste water being treated, oxidizing polluting substances especially in biological treatment systems, it is also useful for the removal of gases and to homogenize the process.
Lately, the selection, specification and evaluation of aeration systems have been widely studied, with the purpose of minimizing energy costs. Due to the fact that in an aerobic system for water treatment, aeration is generally the part of the process that consumes the most energy. Therefore, the selection and design of the most efficient processes is very important.
The current trend is toward processes where the proportion of transferred oxygen per unit of energy consumed increases, improving the system's architecture, increasing the exchange area, the time of suspension of the gas bubbles in the liquid, the concentration of oxygen in the gas, and the turbulence and mixing of the phases. Most of these factors are achieved with micro-bubble air injection systems.
The coefficient of oxygen transferred KLa is affected by other variables, physical as well as chemical, that are characteristic of each aeration system such as temperature, system architecture, and water chemistry.
Water chemistry is the most influential factor in the KLa variation factor in wastewater treatment. Since these water currents hold a great variety of dissolved compounds they give each current special characteristics, depending on the origin of the waters, the presence of tensoactive agents and other organic compounds is highly probable. These molecules can direct themselves in the interface and create a diffusion barrier. One of the most important properties of these tensoactive substances is that in very small quantities they can drastically reduce the KLa.
Given the great variety of factors that affect the oxygen transference rate, the energy efficiency expressed as kilograms of oxygen transferred per Joules consumed (kg·O2exch/J) was determined as the factor of comparison for each of the systems evaluated.
The main purpose of this study is to evaluate the oxygen transference capacity of the ejectors' combined systems, distribution pipelines and dispersors in order to determine the influence of each of these components and compare them with the main type of aerators the marketplace (1,3). In relation to the conditions of the process and energy consumption (Water Environment Federation, American Society of Civil Engineering, 1988)
Experimental description
The equipment and materials used to carry out of this evaluation are as follows:
Water
Table 1 shows the characteristics of the water used in the experiment.
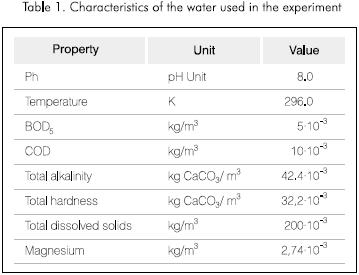
Air. The air injected during the tests was directly taken from the atmosphere with a 70% relative humidity.
Sodium sulfide. A commercial grade sodium sulfide used with 98% purity, with a cobalt catalyst to remove oxygen.
Gauge to measure dissolved oxygen. The gauge used to measure the dissolved oxygen is a digital system, YSI brand MODEL 55.
Aerators. Ejectors with different architectures were used, including an injector built at the facilities of the Instituto Colombiano del Petroleo (ICP). Mechanical pallet (blade) surface aerators were also used with 1.2 and 3.7 w motors, respectively. The scaled pilot plant tests were carried out in 0.22 m3 and 30 m3 tanks.
Theoretical review
The gas-liquid operation is defined as a mass transfer process, in which gas is transferred from one phase to the other, in this case from the gas phase to the liquid phase (2). To accomplish this objective, close contact between the fluids must be sought, so that the interface diffusion of its components can be carried out.
The rate of the gas-liquid mass transference directly depends on the surface exposed between the two phases, so the nature and the dispersion rate of one fluid within another are very important.
Given the low solubility of oxygen in water and consequently a low rate of transference, it often happens that the quantity of oxygen that penetrates the water through the liquid's atmosphere-surface interface is not enough to satisfy the oxygen demand of the aerobic treatment. In order to transfer the large quantities of oxygen necessary, additional interfaces must be created. With this objective, air or oxygen can be introduced into the water or the liquid can be exposed to the atmosphere in the form of droplets. In the latter system, if there are not the right conditions of liquid temperature and the relative humidity of the gas the processes to be carried out will be mainly the cooling of the liquid, or the humidification (moistening) of the air.
Most mass transference in the aeration process occurs during the period in which the bubbles rise to the surface. The time these bubbles remain suspended in the liquid depends on their size. That is why it is important that their diameter be as small as possible sothat they can remain suspended for longer periods of time and therefore a greater mass transference conserving an economical balance with respect to the energy consumed. Generally, the most economical oxygenation processes are those with intermediate transference efficiency (30% - 60%), which correlates the transferred oxygen with the injected oxygen.
In the Double Layer Theory (Metcalf and Eddy Inc, 1995), the transference at the interface is described as the confinement of two thin static films during the gas-liquid interface. This model considers that all the resistance to the mass transference is contained within the two films and none in the interface. For this reason, the interfacial concentrations are in balance and can be determined from the balance distribution curve.
In this manner, the double layer theory is based on a physical model in which there are two layers in the gasliquid interface. The two layers, one liquid and the other one gaseous, show a resistance to the movement of the gas molecules between the phases. During the gas molecule transference, from the gaseous phase to the liquid phase, the force of liquid layer is the most difficult resistance to overcome, for those lightly soluble gases, and as for those highly soluble gases, the gaseous layers show greater resistance. The intermediate soluble gases are subject to a significant resistance by both layers.
In the systems used in the wastewater treatment, the mass transference rate of the gases is generally proportional to the difference between the actual concentration and the dissolved gas balance concentration.
The equation of this correlation can be expressed
as:
where,
dm/dt = gas mass transference rate.
Kg = gas diffusion coefficient
A = area through which the gas diffuses.
Cs = saturation concentration of the dissolved gas.
C = concentration in the liquid of the dissolved gas.
V = volume
Since dm/dt = V dC/dt, the above equation can be written as follows:
In practice, the term Kg · (A/V) is replaced by a proportionality factor, which is identified in literature as KLa, and it is replaced in the last equation. This factor is related to the resistance effect which molecules experience as they move through the phases, furthermore, it is a function of the existing liquidair interface area per unit of fluid volume.
When the double layer model is applied to the oxygen transference rate in a non-stationary state for these water reoxygenation processes, it can be expressed by the following basic model (Jenkins et al., 1980; Huibregtse et al., 1983; American Public Health Association, 1980):
where,
dC/dt = exchange rate of the oxygen concentration
DO KLa = apparent volumetric coefficient of oxygen
transference C* = oxygen concentration in saturated conditions Ct = oxygen concentration in time t.
In the present study the values for the oxygen transferred in kilograms and the quantity of power consumed (Kilowatts) in the oxygenation process were directly gathered in order to obtain the real value of energy efficiency expressed as:
There are other factors that must be taken into consideration such as bubble size and the degree of water agitation.
For a given quantity of air introduced in a liquid system, the surface available through which gas transference can occur increases as the bubble size decreases. Also the turbulence reduces the thickness of the liquid layer and decreases the resistance to the dissolved gas transference and its dispersion once the transference has taken place.
A qualitative analysis of the bubbles' diameter and the systems analyzed is performed to determine, in a preliminary manner, its influence on the efficiency of the mass transference.
Testing procedures
In general, these oxygenation tests were carried out under non-stationary state conditions. Tap water was used. It is quality was considered constant throughout the course of this study.
To eliminate the dissolved oxygen in the test water, sodium sulfide catalyzed with cobalt salts was added, previously diluted, in a proportion from 1.3 to 1.4 which is stoichiometrically required to eliminate the fluid oxygen concentration to less than 0.1 ·10-3 kgO2/m3 (Water Environment Federation, American Society Of Civil Engineering, 1988)
At the time the sulfide was added, a mixing system was engaged in which the flow patterns were generated to distribute in a short period of time the oxygen trapping solution, minimizing the turbulence in the water-air interface, thus avoiding the reoxygenation of the fluid. The dissolved oxygen (DO) was continuously measured at different points in the container. When it did not vary during a 15 minute period, it was considered that the system was ready to start the oxygenation process.
During aeration it was determined that the DO measures were constant at any point of the reactor. This was reported as a result of the flow patterns due to the mixing system present in each of the experiments. For this reason, in most of the tests only one point in the tank was used to establish the DO conditions at an intermediate depth and at 2/3 the distance between the center of the tank and its walls.
In addition to the DO measurements, other facts were tabulated which are important to the aeration process, such as water temperature, energy consumption on line, air flow in those cases where it was possible to measure it given the conditions of the different aerators. During each test, a qualitative evaluation of the air bubbles was performed by measuring their rising time in a three meters water column. The size of the bubble was qualitatively rated on a scale from 1 to 10, where 1 corresponds to micro-bubbles and 10 to macro-bubbles. This bubble size scale is an informal way to evaluate the bubble size for every ejector evaluated.
Results
Different vacuum ejectors, which used water or vapor as motor fluid, were selected to carry out this evaluation. The behavior of most of these ejectors is generally described by the vacuum pressure, the evacuation rate of a closed container, the liquid or gas displacement rate in function of the flow and pressure of the motor fluid. But none of the cases show the comparative characteristics or rates that would allow to determine the one with the best behavior in mass transference systems. The ejectors used to carry out the study were selected by the averages of the variables listed in Table 2.
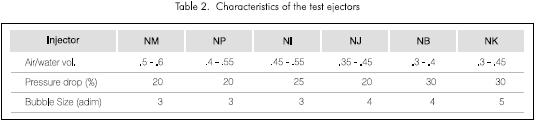
The ejectors selected should show the greatest ratios of absorbed air to pumped water volume, that the pressure drop of the fluid across the nozzle is low and therefore is energetically favorable. And in addition, the bubble size at the ejector's outlet should be as small as possible, given the fact that it increases the bubble's residence time in the water and at the interface area, which allows mass transference. Therefore, the NM, NP and NI ejectors were chosen to continue the study.
Figure 1 shows the preliminary results of the operation of only the ejectors, of the ejectors with the distribution network, and finally of the complete system with the dispersion of the oxygenated (aerated) water in the water to be treated. Initial results show that during the operation of the system, the distribution network and the dispersion system represent on the average a 26% and 18% efficiency of total system respectively. The use of the ejector system itself does not assure a high degree of oxygenation but instead that the dissolved oxygen levels are not homogeneous in the test tanks. Therefore the information presented here in includes complete systems.
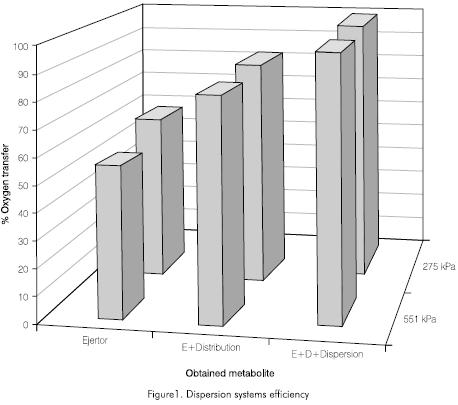
Figure 2 shows the behavior of the DO in function of time for the ejectors selected, at 551.6 kPa (80 psi). It can be observed that injector NM shows the best behavior to the operational conditions so as to improve the DO in the water to be treated. Initially the behavior for the injectors was similar, but after 5 min., injector NI lost its capacity to dissolve oxygen, and only reached DO values of less than 6 · 10-3 kg/m3 (6.00 ppm). While the other two injectors continued behaving similarly, the NM injector had a slight advantage, reaching DO levels close to 8 ·10-3 kg/m3 (8.00 ppm).
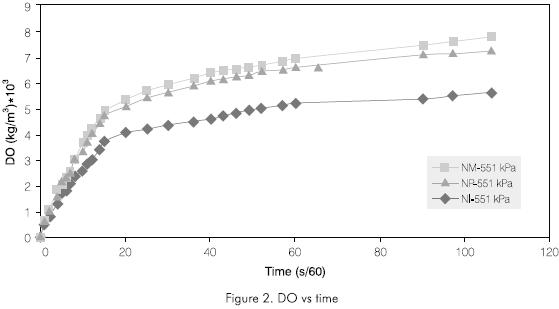
Figure 3 shows that the transference rates for the three injectors is maintained in the same order of efficiency order given at 551.6 kPa (80 psi). Nonetheless, important changes can be observed, the greatest levels at the end of the test are lower than those at 80 psi, the NI injector was the one with the lowest transfer rate. The similarity shown between the NM and NP injectors at 551.6 kPa (80 psi), was not present at 275.8 kPa (40 psi).
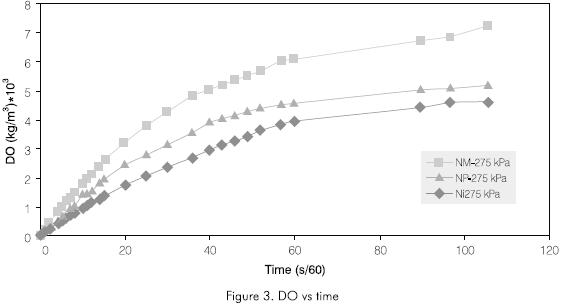
It is noteworthy that the DO levels reached are decreased by more that one unit for all the injectors and especially for the NP injector as shown in Table 3, where the degree of bubble size is reported.
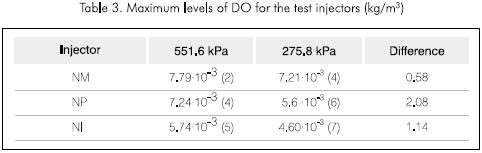
Tables 4, 5 and 6, show the flow of every injection system, the power consumption and the calculated KLa, respectively. It can be observed that the water flow in each of the cases is smaller for the NM injector and thus the power consumption is less. Also the KLa is always greater for the NM injector. These properties of the NM injector led to its selection for further tests under different pressure conditions and its results are shown in Figure 4 and Table 7.
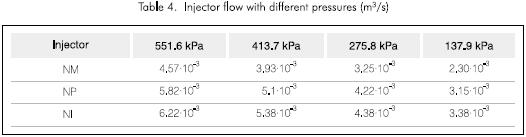
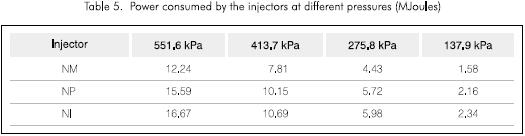
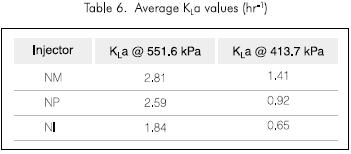
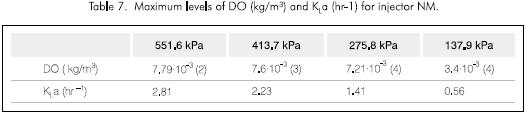
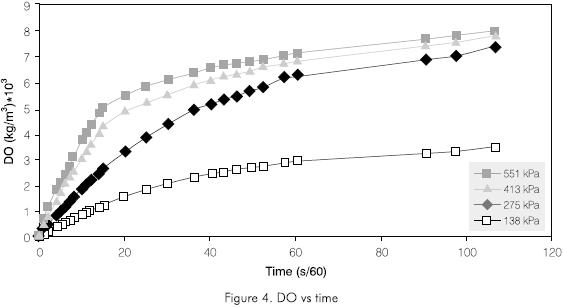
Equation 2 was used to calculate KLa. The reported value is the average of the estimated values for each time interval divided by the data taken from every test.
Figure 4 shows the efficiency behavior of the injector for transferring oxygen in kg·O2 Trans/MJoules.
The energy efficiency of the transference process varies and is a function of time for the case analyzed here; a non-stationary state. However, it is concluded that the most effective pressure for the NM injector is 137,9 kPa (20 psi) (Figure 5).
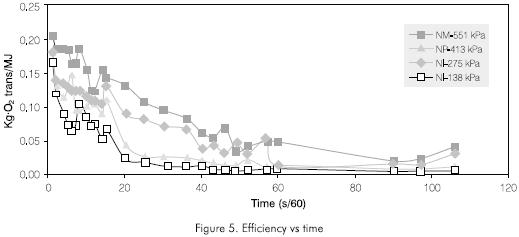
The efficiency of this system was compared with other conventional surface aerator types such as the disk or the propulsion types. These tests results are shown in Figure 6. It can be observed that the proposed injection system is always the most efficient. If the areas under the curves are evaluated at different times, it can be concluded that the injection system is 30% -40% more efficient than the disk aerator and 50% -60% more efficient if compared to the propulsion type.
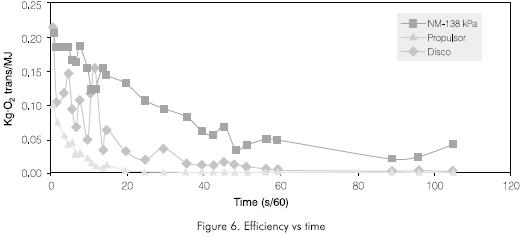
Conclusions
- The air injection system used to transfer oxygen to the water to be treated is energetically more efficient (30% - 60%) than the surface aeration systems (disk, propulsion) in test conditions.
- The aeration efficiency of an ejector without an adequate distribution and dispersion system is reduced by 45%.
- The injection aeration system also improves other processes which are helpful in the treatment of waste-water, such as the separation of solids, grease and hydrocarbons by buoyancy, homogenization and disposal of dissolved gases.
- The installation, operation and maintenance of an injection aeration system are cheaper than those for the surface systems obtaining 90% maintenance savings and 20% energy savings in the industrial systems already working in Ecopetrol.
- This aeration process allows the regulation and control of the quantity of oxygen, rationing energy consumption (cost reduction).
- In addition to its installation it allows the injection of other gases that could be required in the treatment (CO2, N2).
References
American Public Health Association et al., 1980. Standard Methods for the Examination of Water and Wastewater, USA, 15th edition. [ Links ]
Huibregtse, G. et al., 1983. "Factors Affecting Fine Bubble Diffused Aeration", in: Journal WPCF, 55 (8): 1057 - 1064. [ Links ]
Jenkins, D., Greenberg, A. and Connors, J. 1980. Standard methods for the examination of Water and Wastewater, 15th Edition, p: 85 - 90 [ Links ]
Metcalf and Eddy Inc. 1995. Ingeniería de aguas residuales, tratamiento, vertido y reutilización, tercera edición, vol. I y II, Mc Graw Hill, España, p: 314 - 326, 633 - 656. [ Links ]
Treybal, R. E. et al. 1980. Operaciones de Transferencia de Masa, segunda edición, McGraw Hill Co., México, pag: 247 - 294. [ Links ]
Water Environment Federation, American Society of Civil Engineering, 1988. "Aeration a Wastewater Treatment Process", Manual of Practice FD 13. [ Links ]
CT& F - Ciencia, Tecnología y Futuro - Vol. 1 Núm. 5 Dic. 1999. [ Links ]