Services on Demand
Journal
Article
Indicators
-
Cited by SciELO
-
Access statistics
Related links
-
Cited by Google
-
Similars in SciELO
-
Similars in Google
Share
CT&F - Ciencia, Tecnología y Futuro
Print version ISSN 0122-5383On-line version ISSN 2382-4581
C.T.F Cienc. Tecnol. Futuro vol.3 no.2 Bucaramanga Jan./Dec. 2006
Caroll López¹ and German Y. Ojeda²
¹Universidad Industrial de Santander, Escuela de Geología, Bucaramanga, Santander, Colombia. e-mail: clopez@uvic.ca
²Ecopetrol S.A – Instituto Colombiano del Petróleo, A.A. 4185 Bucaramanga, Santander, Colombia
(Received Jun. 15, 2005; Accepted Sept. 1, 2006)
ABSTRACT. Geothermal heat flow in gas hydrate zones can be determined theoretically from the Bottom-Simulating Reflector. A Bottom-Simulating Reflector (BSR) has been observed in multichannel seismic data from the Caribbean offshore Colombia. This anomalous reflector, known to correspond with the base of the Gas Hydrate Stability Zone (GHSZ), can be used to estimate a range of possible values for the local heat flow using the stability field of gas hydrates and the thermal conductivity of the sediments. The study area extends from northern Guajira in the north to the Gulf of Uraba in the south, covering some 18 000 km2. The heat flow was found to be 27,6 ± 4 mW/m2 off the coast of Barranquilla, 37,9 ± 3,6 mW/m2 for northern Guajira, 33,2 ± 5,5 mW/m2 for the central Guajira and 34,7 ± 9,4 mW/m2 for the Cartagena –Gulf of Uraba offshore areas. These results are comparable to heat flow estimates of 30 and 42 mW/m2 obtained for the south Caribbean by others using alternate methods. The estimated heat flow is relatively low in relation to other continental margins. The continuous and fast sedimentation rate from the Magdalena River may explain the anomalously low heat flow in the river delta area. The Cartagena-Gulf of Uraba zone evidences the presence of advection heat loss in the accretionary wedge of the South Caribbean deformation front.
Keywords: gas hydrate, bottom simulating reflector (BSR), heat transfer, Caribbean area, Colombia.
RESUMEN. El flujo de calor en zonas con presencia de hidratos de gas puede ser determinado teóricamente a partir de la ocurrencia del reflector simulador de fondo (BSR por su sigla en inglés), el cual ha sido detectado en registros de reflexión sísmica adquirida costa afuera del Caribe Colombiano. Este reflector anómalo, el cual se cree que coincide con la base de la zona de estabilidad de la capa de hidratos de gas, permite estimar un rango de valores de flujo de calor a partir de los diagramas de estabilidad de los hidratos y la correspondiente conductividad termal de los sedimentos. En este estudio se determinó el flujo de calor en el área costa afuera que se extiende desde la alta Guajira hasta el golfo de Urabá con un área interpretada de BSR de aproximadamente 18 000 km2. Así, en estas regiones, el flujo de calor calculado varía de 27, 6 ± 4 para el sector costa afuera de Barranquilla en la zona correspondiente al delta del río Magdalena,
37,9 ±3,6 y 33,2 ± 5,5 mW/m2 para el sector costa afuera de la alta Guajira y Guajira central respectivamente y 34,7 ± 9,4 para el sector costa afuera de Cartagena - Golfo de Urabá. Estos resultados son congruentes con estimaciones obtenidas en el Caribe Sur empleando métodos alternos, los cuales varían entre 30 y 42 mW/m2. Los resultados obtenidos indican un flujo de calor estimado relativamente bajo en relación con otras márgenes continentales. La rápida y contínua sedimentación en el delta del río Magdalena parece disminuir el flujo de calor característico de esta zona. No hay evidencia de calor friccional en el frente de deformación del Caribe Sur. Sin embargo, la zona del golfo de Urabá en Cartagena evidencia la presencia de calor por advección en la cuña de acreción del frente de deformación Caribe Sur.
Palabras claves: hidratos de gas, reflector simulador de fondo, transferencia de calor, Caribe, Colombia.
INTRODUCTION
Knowledge about the flow of heat generated inside the earth is fundamental in order to understand local hydrodynamics (Hyndman & Davis, 1990), for geo-chemical modeling, (Tissot & Welte, 1984), and continental margin geo-dynamics (Grevemeyer & Villinger, 2001). Geothermal heat is transferred by conduction, convection, and/or advection, and distributed throughout the crust, and from there on, to the hydrosphere and the atmosphere, decreasing gradually outward (Turcotte & Schubert, 1982). Simplified heat flow is calculated according to Fourier’s law as follows (Turcotte & Schubert, 1982):
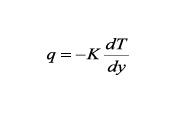
Where K is the material thermal conductivity, and is the geothermal gradient.
Several techniques can be used to measure heat flow. In continental zones, heat flow can be obtained directly at drilling sites. At the ocean bottom, heat flow can be measured with the aid of thermal probes, which penetrate into the surficial sediment, to estimate local heat flow. Indirectly, estimations derived from the depth of the limit of thermal stability and seismic reflectivity from the base of the gas hydrate layer are also used (Grevemeyer & Vilinger, 2001). In this research, the heat flow was calculated from the geothermal gradient constrained by the hydrate occurrence map since there exists seismic evidence for gas hydrate occurrences in the Colombian Caribbean continental margin.
Gas hydrates
Gas hydrates are solid substances composed of rigid water molecules that enclose natural gas. When the natural gas is mainly methane (>99%), these substances are named methane hydrates. Gas hydrates are important for three main reasons: (i) they represent a great potential energy resource, (ii) they pose a natural submarine slope stability risk, produced by their potential for destabilization, and (iii) they might result in “greenhouse” type climate effects due to their possible emission of methane into the atmosphere (Kennett, Cannariato, Hendy, & Behl, 2003).
Gas hydrates are formed offshore at the ocean bottom, and on land in permafrost zones, where appropriate physical conditions exist (moderately low temperatures and high pressures), and gas is available (e.g. zones saturated in gas and water) (Collett, 2002).
Previous work
Colombian Caribbean gas hydrate occurrence was first described by Shipley et al. (1979) base on the Bottom-Simulating reflector (BSR). Nevertheless, only few studies have derived thermal information from the occurrence of hydrates.
Based upon four seismic lines acquired by Lamont Doherty Observatory in 1977, Ladd et al. (1984) interpreted the BSR along the South Caribbean deformed belt, between depths of 2,3 to 4,2 kilometers. They calculated the geothermal gradient from the observed BSR, obtaining a value of 313,15 K/km. Gomez and León (2000), evaluated the existence of methane hydrates in the Colombian Basin, concluding that hydrate deposits could store methane gas in the order of 22 TCM, based on regional seismic lines. Applying gas flow in porous media, heat conduction, and gas hydrate properties equations, Caicedo and Pinto (2003), validated a mathematical model obtained from de-pressurization of a hydrate deposit in contact with a gas layer, by using computer software (SDYHGAS).
López (2005) determined the geothermal gradient from the BSR assuming that it represents the base of the gas hydrate stability zone. However a map of the regional heat flow variation does not yet exist. The heat flow maps derived from the BSR distribution in the study area mapped by López (2005) are shown in this paper.
The bottom simulating reflector
The occurrence, mapping, and estimations of the volume of marine gas hydrate accumulations are inferred mainly from the presence of the BSR in seismic sections. BSR’s are anomalous seismic reflectors resulting from a change in acoustic impedance produced by a drop in the speed of sound, which is caused mainly by the presence of trapped gas under the hydrate stability zone, i.e. the BSR marks a transition between the gas hydrate zone and the free gas zone (Kvenvolden, 1998). Consequently, the BSR seems to match closely the base of the hydrate stability zone. This reflector can be identified by three particular characteristics: (i) it emulates the shape of the ocean floor, (ii) it can cut transversely across stratigraphic reflectors, and (iii) it has an opposite polarity to the ocean bottom reflector (Kvenvolden, 1998).
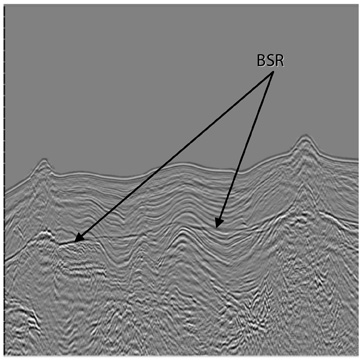
On the other hand, the absence of a BSR does not necessarily indicate an absence of gas hydrates, i.e. not all oceanic gas hydrate accumulations have associated BSR’s. Gas hydrates in the absence of BSR have been found in marine regions in Mexico, the United States, and other parts of the world (Collett, 2002).
STUDY AREA
The region of interest is located in the Colombian Caribbean Sea, and it extends from the Gulf of Uraba, in the south, to high Guajira, in the north, with a lateral extent ranging from 60 to 170 kilometers from the coastline. The area is located on and Southeast of the South Caribbean deformed belt, which is located along the Northwest border of the North Andean Block, from the Gulf of Uraba to the Guajira Peninsula (Ladd et al., 1984).
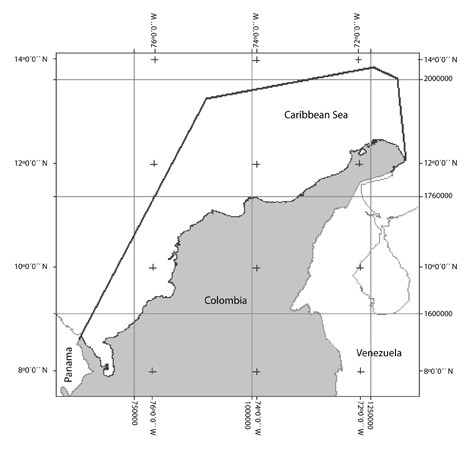
HEAT FLOW CALCULATION FROM BSR
The heat flow for the study area was estimated from the geothermal gradient data obtained by López (2005), which was itself indirectly estimated from the BSR observed in 2-D seismic lines. The method used by Lspez (2005) is summarized in five steps: (1) interpretation of BSR and the seafloor from the seismic reflection data; (2) time-depth conversion of the BSR and ocean bottom maps by means of a velocity-depth function; (3) estimation of the pressure exerted by water column and sediments with methane hydrates, in order to obtain the total pressure at the base of the hydrate layer; (4) conversion from pressure to temperature, based upon the methane hydrate stability diagrams by Shipley et al. (1979), in order to determine the temperature at the base of the hydrate layer; and (5) estimation of the geothermal gradient from the differences in temperature and depth between the ocean bottom and the base of the GHSZ, assuming that hydrates have a 96,5% methane content and 3,5% NaCl. According to López’s work (2005), the geothermal gradient is 30 ± 276,15 K/km for high Guajira, 26 ± 277,75 K/km for Central Guajira, 22 ± 276,65 K/km for Santa Marta – Barranquilla sector, and 27 ± 281,15 K/km for Cartagena – Gulf of Uraba sector (Figure 3).
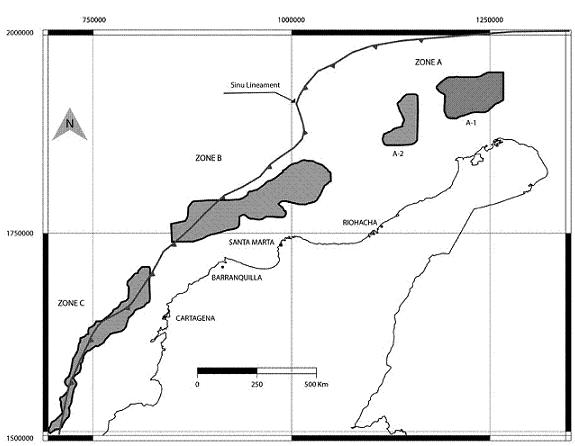
In order to obtain heat flow distribution maps, López’s geothermal gradient maps (2005) were used in this study assuming constant thermal conductivity. Thermal conductivity is one of the variables generating most of the uncertainty in heat flow calculation, since it can vary widely in marine sediments (Minshull, Singh, & Westbrook, 1994). Since there are no in situ data available from the study area, a 1,25 W/m°K constant value suggested by Grevemeyer and Villinger (2001) and Minshull et al. (2005) was used. They claim that the variation of thermal conductivity with depth is small, and negligible with respect to the effects of gas hydrates. The 1,25 W/m°K value corresponds to the average of the Chilean (Grevemeyer & Villinger, 2001) and Cascadia (Davis, Hyndman, & Villinger, 1990) margins, and it is consistent with the average thermal conductivity recorded at ODP well 99, where conductivities vary from 0,8 to 1,6 (Sigurdsson, Leckie, & Acton, 1997 ). Uncertainty in flow results thus calculated is typically 10%, even though in some cases it might be larger (Grevemeyer & Villinger, 2001).
A highly used empirical expression for the average thermal conductivity (k) from the surface was obtained by Davis et al. (1990): k= 1,07+5,86x10-4z – 3,24x107z2. This expression is used to analyse the variability of thermal conductivity with respect to gas hydrate thickness. As the thickness may be uncertain since the BSR does not show the top of GHSZ, heat flow may change from about 1,1 W/m°K (assuming a 0,1 m of thickness for the gas hydrate layer) to 1,33 W/m°K (assuming the maximum thickness of 900 m for the gas hydrate layer in the Colombian Caribbean). These possible changes in thickness of the gas hydrate layer render an uncertainty in heat flow results that can vary from 1 to ~20%.
Regional heat flow variation
Heat flow in the Colombian Caribbean shows spatial variations, both parallel and perpendicular to the current continental margin, and it is different for the three study areas. Table 1 shows mean values and standard deviations for each work zone.
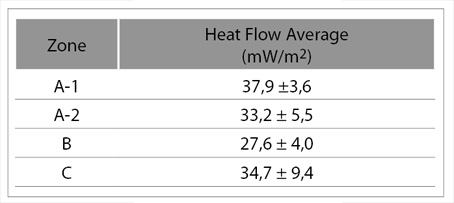
The Sinú Lineament marks the NW limit of the South Caribbean deformed belt, and it works as a reference line in order to analyze the variation of heat flow within the study zone. This lineament was drawn based upon the map published by Audemard, Machette, Cox, Dart and Haller (2000). The Sinu Lineament intersects Zone C longitudinally, it intersects and is tangential to Zone B, and lies some 60 kilometers north and west of Zone A (Figure 3).
Heat flow maps provide an indication of heat flow base values and variability in the Colombian Caribbean (Figures 4, 6 and 8). Mean values in these maps indicate heat flow is relatively high in the High Guajira, where it reaches 37,9 mW/m2. Its standard deviation, which can be interpreted as measure of local variation, is relatively low (3,6 mW/m2), compared to the remaining zones and could be due to variations in thermal conductivity. In Central Guajira, the mean value is 33,2 mW/m2, and it varies some 5,5 mW/m2 around this value. Zone B shows the lowest mean within the study area, with a value of 27,6 mW/m2, and a standard deviation of 4 mW/m2. Zone C, which is dissected by the Sinú Lineament, shows a 34,7 mean value, and the largest local variation, with a 9,4 mW/m2 standard deviation.
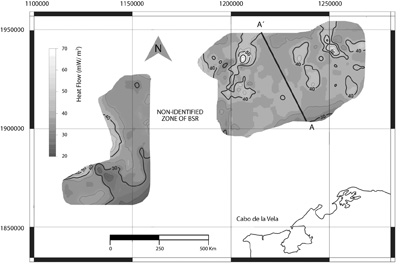
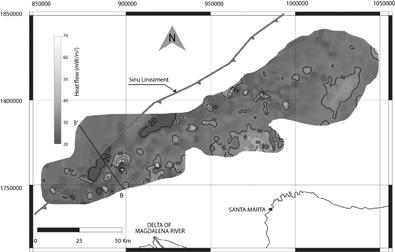
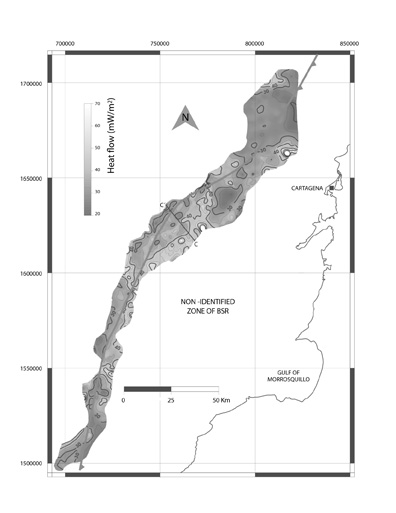
The heat flow variations in the direction perpendicular to the Sinu Lineament are showed in figures 5, 7 and 9. Cross section A-A’ shows the heat flow variation offshore High Guajira. This cross section shows a slight regional heat flow variation, which seems to decrease towards the continent. The trend line obtained via regression suggest a heat flow decreasses towards the continent within the study zone at a rate of 0,16 mW/m2 per kilometer along the section line.
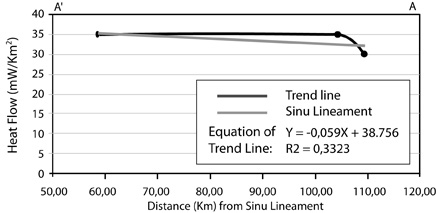
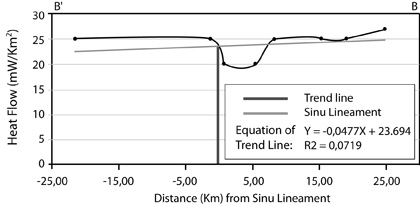
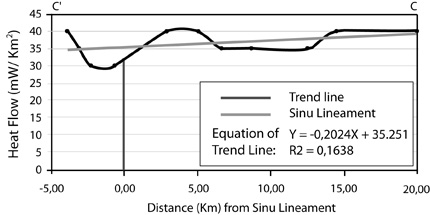
In contrast to the High Guajira section, section B-B’ seems to indicate a 20 to 27mW/m2 increase of heat flow toward the continent (Figure 5). Initially, 35 kilometers away from the coast line, a slight heat flow decrease around 2 mW/m2 is observed, after which it is constant up to 50 kilometers from the coast. From that point on, a low close to 5 mW/m2 is observed. The heat flow shows another deflection, which matches the Sinu Lineament boundary and is consistent with sediment thermal blanketing (Zhang, 1993) (Figure 6).
In the 25 kilometers long Section C'-C, heat flow fluctuates between 30 and 40 mW/m2 moving from east to west (Fig. 9). It is constant along the first 7 kilometers, decreasing to 35 mW/m2 along a 7 kilometers distance, which corresponds to a local low, beginning 307 kilometers from the coastline. Some 321 kilometers away, where the Sinú lineament intersects the profile, a deflection of the trend line is found again as in Zone B, but in the opposite sense, that is, the gradient decreases west of the lineament, beginning 2 to 3 kilometers away from it. From this point on, it gradually climbs back to 40 mW/m2 (Figures 8 and 9). The regression line shows a 0,25 mW/m2 increase per linear kilometer toward the continent.
Zone B is rather homogeneous, with a slight tendency toward increased heat flow toward the continent (Figures 6 and 7). This heat flow behavior in the B zone might be related to the presence of the Magdalena River delta in this area, and its decreasing sediment contribution away from the coast, which influences heat flow to be smaller in the higher sedimentation zone. Magdalena River is the river contributing with the largest amount of sediment to the Colombian Caribbean coastline.
DISCUSSION
The results of heat flow obtained with this technique are consistent for Zone C, with 30 mW/m2 calculated in the Gulf of Morrosquillo by García (1991), and with the 41,8 mW/m2 heat flow estimated by (Epp, Grim, & Langseth, 1970) for the Caribbean considering the appropriateness of the thermal conductivity assumptions, that are included in the standard deviation of every calculation (as found in García, 1991), suggesting the technique is sound. The heat flow values obtained in the present research for the different delimited zones (Table 1) give results similar to the ones that were calculated on the margins of the Gulf of Mexico, Nicaragua and Costa Rica (Table 2).
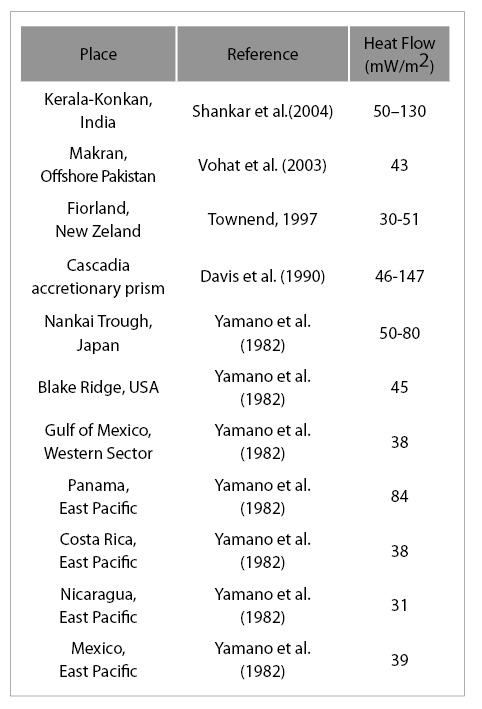
Zone C is interesting because it is being intersected by the boundary of Sinu lineament, which represents the front of the South Caribbean deformed belt, an accretionary prism, produced by the subduction of the Caribbean plate beneath the South American plate (Kellogg & Bonini, 1982). The thermal regime in accretionary prisms is controlled by many parameters and processes such as the age of the subducting plate, basal frictional heating, radiogenic heat production, the thermal and mechanical properties of the sediments, and the deformation pattern of the prism (Wang, Hyndman, & Davis, 1993). The heat flow behavior in zone C is opposite to that observed at other accretionary prisms, where heat flow decreases toward the continent, such as Cascadia, Makran, and the Middle American Trench (Ganguly, Spence, Chapman & Hyndman, 2000; Kaul, Rosenberger, & Villinger, 2000; Mishull et al., 2005). Heat flow in zone C might indicate that the tectonic piling in the accretionary prism of the South Caribbean generates a thermal wedge effect that attenuates heat in the outbound direction from the coast, or might indicate topographically driven advective heat transfer from fluids being expelled from deeper levels of the accretionary prism, commonly observed in this type of structures.
There is no local positive anomaly observed in the heat flow maps in areas where the Sinú Lineament is intersected. This might imply that the Sinu Lineament has not been active recently to generate enough heat to produce a positive anomaly, or that the lineament is a zone of active faulting where heat dissipates without a characteristic thermal abnormality taking place. The latter effect could be explained, for example, by an active hydrological system that might exist along the faults and permeable conduits, in such a way that these would allow cooling and consequently thermal equilibration of the accretionary prism. This behaviour is observed in Nankai, and Cascadia (Hyndman & Davis, 1990), and it also might explain the differences along the strike of the deformation zone, since it is possible to expel hot waters as well as cool ones, as is typical of the cold and hot springs observed in thrust belts (Osadetz et al., 1992).
Generally speaking, the heat flow values obtained do not reveal great variations in the continental shelf and slope in the Colombian Caribbean. However, off the coast at Barranquilla-Santa Marta (zone B), the region corresponding to Magdalena River delta shows relatively smaller heat flows than the rest of the study area. Zone B is a high sedimentation area, showing mud diapires (Duque-Caro, 1984), and consequently with higher gas and water concentrations, which might imply low thermal conductivity. Additionally, it is possible that the continuous supply of sediments does not allow the thermal state of the basin to stabilize. On the other hand, independently of the sedimentation rate, the delta sediments (sands and mud) might exert a thermal isolating effect, since they have a relatively low thermal conductivity. Low thermal flow values might result from the effect of fast and continuous sedimentation (Zhang, 1993).
As it might be observed in each of the zones, A, B, and C, some local heat flow highs and lows appear in the 5 mW/m2 range. There are several possible explanations for the local heat flow variations. Some authors explain the local heat flow variations as environmental processes perturbing superficial flow (Vanneste, Poort, Batist, & Klerkx, 2003). Others consider hydrothermal circulation within the ocean crust, as is the case in Cascadia (Davis et al., 1990). In most cases, however, it is considered that fluid migration plays the main role in these local variations, contributing not only to the formation of the gas hydrates, but also to their dissociation (Chand & Minshull, 2003). In the study area it is common to find diapirs and mud volcanoes (Duque-Caro, 1984; Ojeda, Hernandez, & Olaya, 2004), which might affect the heat flow locally, because of their role in advective heat transfer by fluids migrating in the accretionary complex.
An important issue regarding fluid migration is that along with gas, heat migration is produced. This might cause local dissociation of gas hydrates, which might be observed seismically at the BSR level. This process might result in a BSR migration or formation of a double BSR in some cases (Chand & Minshull, 2003). Faults, for instance, may act as conduits transporting fluid flows (water and gas) and thus heat flow. Figure 10 shows a sector of a seismic line from zone B. In this section, a local BSR dislocation is observed, as an effect of a fault, and the heat flow of the footwall is larger than that of the hanging wall. Nevertheless, this evident variation in heat flow in Figure 10 is in the 3 mW/m2 range. This figure might indicate low fluid flow through this fault, or, alternatively, that its movement is recent enough to have perturbed the heat flow substantially. To the right of this slight decrease in heat flow, a subsequent increase in the 3 mW/m2 range is observed, which is coincident with a diapir and mud volcano, and hence it shows the heat generated by mud rise.
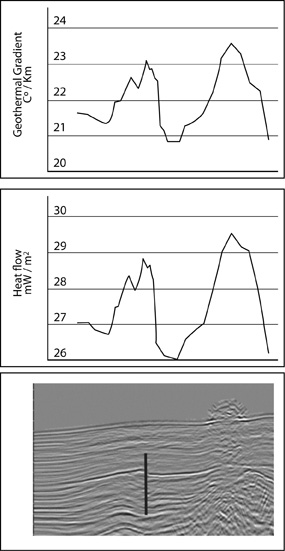
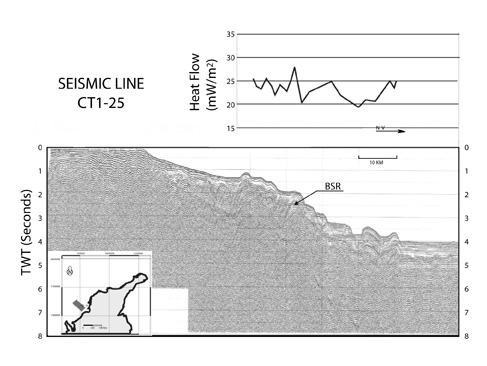
Likewise, in Figure 10, seismic line CT1-25, published by Lu et al. (1983) can be seen. It is located on zone C, with some heat flow points graphed on it. Heat flow behavior along this section is very irregular, which might suggest a larger flow of crustal fluids in this zone.
CONCLUSIONS
• With this study, the spatial heat flow variation in the Colombian Caribbean was assessed. It is characterized as being relatively homogeneous in each zone, with some local highs and lows in the 5 mW/m2 range above and below the base value for each zone. Even though there are local variations, there is not a regional trend of heat flow increase or decrease in a certain direction. These values are distributed from north to south within a 37,9 ±3,6, 33,2 ± 5,5 mW/m2 range for the high and central Guajira, respectively; 27,6 ± 4 mW/m2 for Santa Marta-Barranquilla, and 34,7 ± 9,4 mW/m2 for Cartagena-Gulf of Uraba. The sector corresponding to the Magdalena River (B zone) shows low heat flow, possibly due to low conductivity of sediments, active and rapid sedimentation (which is characteristic of this zone) and large thickness of the unconsolidated sedimentary column.
• The results of this study provide evidence of advectional heat in the accretionary wedge. At zone C, which contains the trace of the South Caribbean deformation front, the increase of flow toward the continent enables inferring that the accretionary prism generates a thermal wedge effect, which attenuates heat in the outbound direction from the coast, and that fluid migration processes could be taken place in this zone.
• The most probable explanation for the spatially irregular heat flow behavior at zone C is the presence of faults and fractures, which might operate as conduits for fluid flow. The small variations in heat flow at the northern part (zone A and B) appear to be dependant on the thermal conductivity assumptions. Finally, it can be concluded that the Colombian Caribbean continental margin is a region with a relatively low heat flow compared to other continental margins.
ACKNOWLEDGEMENTS
The authors gratefully acknowledge the financial support received from Ecopetrol S.A., the Instituto Colombiano del Petrsleo (ICP) and from International Anonimous Evaluators.
REFERENCES
Audemard, F., Machette, M., Cox, J., Dart, R., & Haller, K. (2000). Map and database of quaternary faults in Venezuela and its offshore regions. United States Geological Survey, Open File Report 00-0018. [ Links ]
Beauchamp, B. (2004). Natural gas hydrates: myths, facts and issues. Geological Survey of Canada, 336: 751–765. [ Links ]
Caicedo, J., & Pinto, N. (2003). Esquemas de explotación de Yacimientos de hidratos de gas, primera aproximación de un modelo matematico para el esquema de despresurización. Tesis de pregrado, Escuela de Ingeniería de Petróleos, Universidad Industrial de Santander, Bucaramanga, 150pp. [ Links ]
Chand, S., & Minshull, T.A. (2003). Seismic constraints on the effects of gas hydrate on sediment physical properties and fluid flow: A review. Geofluids, 3: 275-289. [ Links ]
Collett, T. (2002). Energy resource potential of natural gas hydrates. AAPG Bulletin, 86 (11), 1971-1992. [ Links ]
Davis, E., Hyndman, R., & Villinger H. (1990). Rates of fluid expulsion across the northern cascadia accretionary prism: contraints from new heat flow and multichannel seismic reflection data. J. Geophys. Res., 95: 8869-8889. [ Links ]
Duque-Caro, H. (1984). Structural style, diapirism, and accretionary episodes of the Sin?-San Jacinto Terrane, Southwestern Caribbean border, in Bonini, W., Hargraves, R., & Shagam R., Eds., The Caribbean South American Plate Boundary and Regional Tectonics. Geological Society of America Memoir, 162: 303-316. [ Links ]
Epp, D., Grim, P. J., & Langseth. M. J., Jr. (1970). Heat flow in the Carribean and Gulf of Mexico. J. Geophys. Res., 75: 5655–5669. [ Links ]
Ganguly, N., Spence, G.D., Chapman, N.R., & Hyndman R.D. (2000). Heat flow variations from bottom simulating reflectors on the Cascadia margin. Marine Geology, 164: 53-68. [ Links ]
García, M. (1991). Hydrocarbon maturation and clay diagenesis in the Tol? area, Northwestern Colombia. M. Sc Thesis, Department of Geology and Geophysics, University of Wyoming, Wyoming. 154 pp. [ Links ]
Gómez C., & Lesn J. (2000). Recuperación de gas metano en yacimientos de hidratos de gas en la cuenca Colombia como futura fuente de energía. Tesis de pregrado, Ingeniería Química, Universidad Industrial de Santander, Bucaramanga. [ Links ]
Grevemeyer, I., & Villinger, H. (2001). Gas hydrate stability and the assessment of heat flow through continental margins. Geophys. Journal, 145: 647-660. [ Links ]
Hyndman, R.D., & Davis, E.E. (1990). A mechanism for the formation of methane hydrate and seafloor bottom-simulating reflectors by vertical fluid expulsion. J. Geophys. Res., 97: 7025-7041. [ Links ]
Kaul, N., Rosenberger, A., & Villinger, H. (2000). Comparison of measured and BSR-derived heat flow values, Makran accretionary prism, Pakistan. Marine Geology, 164: 37-51. [ Links ]
Kellogg, J.N., & Bonini, W.E. (1982). Subduction of the Caribbean Plate and basement uplifts in the overriding South American plate. Tectonics, 1: 251-276. [ Links ]
Kennett, J., Cannariato, K.G., Hendy, I.L., & Behl, R.J. (2003). Methane hydrates in quaternary climate change: The clathrate gun hypothesis. Geophys., Washington, D.C., 216 pp. [ Links ]
Kvenvolden, K.A. (1988). Methane hydrate – A major reservoir of carbon in the shallow geosphere. Chemical Geology, 71: 41-51. [ Links ]
Kvenvolden, K. A. (1998). A primer on the geological occurrence of gas hydrates, in: J.-P. Henriet, & J. Mienert, (Eds.). Gas hydrates - relevance to world margin stability and climate change. The Geological Society, London, 9-30. [ Links ]
Ladd, J., Truchan, M., Talwani, M., Stoffa, P. L., Buhl, P., Houtz, R., Mauffret, A. & Westbrook G. (1984). Seismic reflection profiles across the southern margin of the Caribbean. Geological Society of America Memoir, 162: 153-159. [ Links ]
López, C. (2005). Determinación del gradiente geotérmico a partir del reflector simulador de fondo. Tesis de pregrado, Universidad Industrial de Santander, Bucaramanga, 100 pp. [ Links ]
Minshull, T.A., Singh S.C., & Westbrook. (1994). Seismic velocity structure at a gas hydrate reflector, offshore western Colombia, from full waveform. J. Geophys. Res., 99 (B3): 4715-4734. [ Links ]
Minshull, T.A., Bartolome, R., Byrne, S., & Danobeitia, J. (2005). Low heat flow from young oceanic lithosphere at the Middle America Trench off Mexico. Earth and Planetary Science Letters, 239: (1-2), 33-41. [ Links ]
Ojeda, G.Y., Hernandez, R., & Olaya, I.D. (2004). Mud volcanoes on the Seafloor of the Colombian Caribbean Sea: undesirable lumps or exploration tools, Proceedings of the 2nd Technical Convention, Asociación Colombiana de Geólogos y Geofísicos del Petróleo, Bogota, Colombia. [ Links ]
Osadetz, K.G., Jones, F.W., Majorowicz, J.A., Pearson, D. E., & Stasiuk, L. D. (1992). Thermal history of the cordilleran foreland basin in western Canada: A review; in: R. W. Macqueen and D. A. Leckie (Eds.), Foreland Basins and Foldbelts. American Association of Petroleum Geologists Memoir, 55: 259-278. [ Links ]
Shankar, U., Thakur, N.K., & Reddi, S.I. (2004). Estimation of geothermal gradients and heat flow from bottom simulating reflector along the Kerala-Konkan basin of Western Continental Margin of India. Current Science, 87: (2). [ Links ]
Shipley, T.H., M.H. Houston, R.T. Buffler, F.J. Shaub, K.J. Mcmillen, J.W. Ladd, & J.L. Worzel, J.L. (1979). Seismic reflection evidence for the widespread occurrence of possible gas-hydrate horizons on continental slopes and rises. AAPG Bulletin, 63: 2204-2213. [ Links ]
Sigurdsson, H., Leckie, R.M., Acton, G.D. (1997). Proc. ODP, Init. Repts, 165: College Station, TX, USA. [ Links ]
Tissot, B.P., & Welte, D.H. (1984). Petroleum formation and ocurrente. (2nd. ed.) Springer – Verlog Berlin Heidelberg New York. Tokyo, 458. ISBN 0-387-13281-3. [ Links ]
Townend, J. (1997). Estimates of conductive heat flow through bottom-simulating reflectors on the Hikurangi and southwest Fiordland continental margins, New Zealand. Marine Geology, 141: 209-220. [ Links ]
Turcotte, D., & Schubert, G. (1982). Geodynamics. New York: Jhon Wiley and Sons Publications, p. 446. [ Links ]
Turcotte, D., & Schubert, G. (1982). Geodynamics. New York: Jhon Wiley and Son Publications, p.135. [ Links ]
Vohat, P., Sain, K., & Thakur, N.K. (2003). Heat flow and geothermal gradient from a bottom simulating reflector: A case study. Current Science, 85: (9), 1263-1265. [ Links ]
Yamano, M., Uyeda, R.M., Aoki, Y., & Shipley, T.H. (1982). Estimates of heat flow derived from gas hydrates. Geology, 339-343. [ Links ]
Yuan, T., Hyndman, R.D., Spence G.D., & Desmons, B. (1996). Seismic velocity increase and deep-sea gas hydrate concentration above a bottom-simulating reflector on the northern Cascadia continental slope. J. of Geophysical Research, 101: (B6), 13655-13671. [ Links ]
Vanneste, M., Poort, J., De Batist M., & Klerkx, J. (2003). Atypical heat-flow near gas hydrate irregularities and cold seeps in the Baikal Rift Zone. Marine and Petroleum Geology, 19: 1257-1274.
Wang, K., Hyndman,R.D., & Davis, E.E. (1993). Thermal effects of sediment thickening and fluid expulsion in accretionary prisms - Model and parameter analysis. J. Geophys. Res., (ISSN 0148-0227), 98: (B6), 9975-9984. [ Links ]
Zhang, Y.K. (1993). The thermal blanketing effect of sediments on the rate and amount of subsidence in sedimentary basins formed by extension. Tectonophysics, 218: 297-308. [ Links ]