Services on Demand
Journal
Article
Indicators
-
Cited by SciELO
-
Access statistics
Related links
-
Cited by Google
-
Similars in SciELO
-
Similars in Google
Share
CT&F - Ciencia, Tecnología y Futuro
Print version ISSN 0122-5383On-line version ISSN 2382-4581
C.T.F Cienc. Tecnol. Futuro vol.3 no.2 Bucaramanga Jan./Dec. 2006
Freddy-Humberto Escobar1 and Matilde Montealegre-M.1
1Universidad Surcolombiana, Programa de Ingeniería de Petróleos, Grupo de Investigación en Pruebas de Pozos, Neiva, Huila, Colombia e-mail: fescobar@usco.edu.co e-mail: matildemm@usco.edu.co
(Received May 25, 2006; Accepted Nov. 15, 2006)
ABSTRACT. Because of fluvial deposition or faulting, many reservoirs around the world may possess a long and narrow geometry in which linear flow is expected to take place once radial flow vanishes, if existed. In any system through which a fluid flows, a change in the path direction causes additional pressure drop. Hydrocarbon reservoirs are not the exception to the rule. A change from radial flow to linear flow will be reflected as an additional pressure drop which may be referred as a skin factor, or more specifically, a geometric skin factor.
A couple of studies in the oil literature have named skin factors due to the change in flow patterns. Besides this, most of the commercial softwares may neither include nor calculate these types of skin factors since the simulations are normally run using the image methods, then, skin factors due to changes in flow path may be neglected.
Using the only methodology available to estimate the linear skin factors, it has been found from the experience on some field cases that this parameter is quite high in some cases and very small in other systems. Therefore, this paper is committed to the determination of the factors affecting the value of the linear skin factor and their impact on reservoir management.
It was found that neither reservoir permeability nor reservoir elongation had a significant impact on the linear skin factor value; however, the well stimulation does play an important role. The more damaged the well, the higher the linear skin factor value. From our simulation study, we found out that no matter if the well has a zero mechanical skin factor, still the linear skin factor has a high value. Besides, we demonstrated that the total skin factor increases as the linear skin factor increases with a ratio of 2,7385: 1,7385. Two Colombian field cases were worked with the purpose of verifying the findings of this article.
Keywords: linear flow, radial flow, parabolic flow, well pressure, well bore damage, well stimulation.
RESUMEN. Muchos yacimientos en el mundo poseen una geometría larga y estrecha causada por depositaciones fluviales o fallamiento. En ellos, se espera el desarrollo del flujo lineal una vez que el regimen de flujo radial, si lo hubiere, se desvanezca. En un sistema a través del cual existe flujo de fluidos, cualquier cambio en la trayectoria de flujo origina una caída de presión adicional. Los yacimientos de hidrocarburos no se exceptúan de esta regla. Un cambio de flujo radial a flujo lineal se refleja como una caída de presión adicional la cual podría tratarse como un factor de daño.
Los factores de daño debido al cambio de patrones de flujo han sido mencionados un par de veces en la literatura petrolera. Además de ésto, la mayoría de los programas de computador comerciales no incluyen la determinación de este tipo de factores de daño puesto que sus simulaciones usan el método de las imágenes, y por lo tanto, los factores de daño debido a cambios en la dirección de flujo podrían estar siendo ignorados.
En este trabajo se determinó por la experiencia del uso de la única metodología disponible para estimar los factores de daño lineales en algunos casos de campo, que éstos pueden tener valores muy altos en algunos casos o muy pequeños en otros sistemas. Por lo tanto, el propósito de este artículo es el de determinar los agentes que afectan el valor del factor de daño lineal y su impacto en la administración del yacimiento.
Se encontró que ni la permeabilidad ni la elongación del yacimiento tuvieron un impacto significativo en el valor del factor de daño lineal; sin embargo, el grado de estimulación en el pozo sí juega un papel importante. Entre mayor sea el daño en el pozo, mayor será el valor del factor de daño lineal. En nuestro estudio de simulación, encontramos que aunque el pozo posea cero daño mecánico, todavía el valor del factor daño lineal es alto. Además, demostramos que el factor de daño total se incrementa con el factor de daño lineal en una proporción de 2,7385:1,7385, respectivamente. Trabajamos dos casos de campo en yacimientos Colombianos para verificar los descubrimientos presentados en este artículo.
Palabras clave: flujo lineal, flujo radial, flujo parabólico, presión de pozo, daño de pozo, estimulación de pozo.
INTRODUCTION
We recognize a representative number of pressure tests conducted in channelized systems which may result from faulting, fluvial deposition or deep sea fans. Even though, these types of systems occur quite often in nature, just few studies have been devoted to their analyses.
Ehlig-Economides and Economides (1985) presented an analytical solution for linear flow to a constant-planar source solution in drawdown tests without considering the linear skin factor. They provided a methodology to estimate permeability, channel width and the extent of well drainage area. Also, Wong, Mothersele, Harrington and Cinco-Ley (1986) presented a mathematical model excluding the linear skin factor. They presented new type curves for transient pressure analysis in rectangular systems. They also applied conventional techniques to actual field data. Later, Massonet, Norris and Chalmette (1993) presented a descriptive study of flow simulations in geological complex channelized reservoirs. They did not mention anything related to the additional pressure drop due to the channel geometry. Larsen and Hovdan (1987) presented a conventional method to analyze pressure data in linear-type reservoirs following a similar fashion as for the radial flow regime with some modifications added by the vt plots. This combination permits the determination of reservoir width, flow capacity, skin and well location. Mattar (1997) presents a set of equations for well test interpretation without type curves. Among these, he presented an equation for the estimation of the channel width, but no solution was devoted to estimate the linear skin factor.
Only Larsen and Hovdan (1987) and Nutakki and Mattar (1982) included in their model an additional pressure drop due to the convergence of the flowlines near the wellbore. They, Nutakki and Mattar (1982), did provide a conventional methodology to estimate the linear skin factor from the intercept observed on a Cartesian plot of pressure versus the square root of time. Their equation was dependent mainly on the reservoir width, transmissibity and wellbore radius. However, they only focused on a linear flow term. Much later, Escobar et al. (2004) presented an exhaustive and comprehensive study for interpretation of well pressure test in elongated reservoirs. They employed the TDS technique, Tiab (1993), to provide a step-by-step methodology to determine reservoir parameters. They introduced two new concepts: dual linear flow, when there exist two linear flows taking place along the two lateral sides of the reservoir as depicted by the dashed arrows in Figure 1. This is represented by a half-slope line on the pressure derivative curve of Figure 2. Once the near lateral boundary has been reached by the transient pressure wave, only there remains a (single) linear flow along the longer side of the reservoir, as also shown by the solid arrows (representing streamlines) of Figure 1. Notice that the pressure derivative displays a rapid change of the intercept, although its half-slope still remains (Figure 2). This classification may sound to be unnecessary for either conventional or type-curve methods; however, it results truly important for the application of TDS technique. They provided analytical equations to estimate both the dual linear and linear skin factor from a pressure and pressure derivative plot. Escobar, Muñoz, Sepulveda and Montealegre (2005) further investigated on elongated systems and discovered a new flow regime in these systems which they called "parabolic flow". An equation to estimate the skin factor due to the change from dual linear to parabolic flow is presented by Escobar et al. (2005). The reader is strongly recommended to check this reference for a better understanding of the present document.
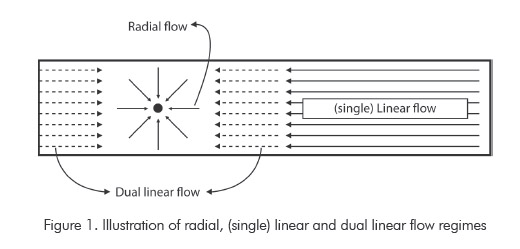
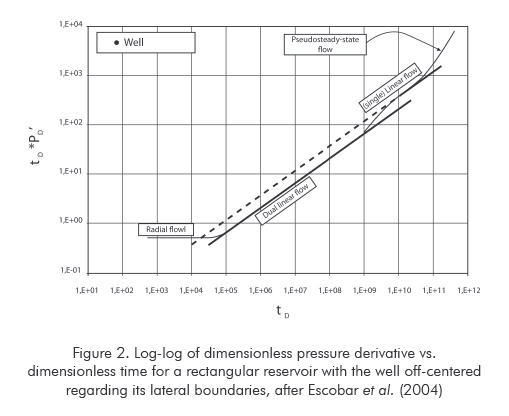
This work uses the mathematical model presented by Escobar et al. (2004) and their methodology for estimation of the linear skin factors to study the effect of reservoir permeability, reservoir geometry and mechanical skin factor on the dual linear skin factor.
FORMULATION
Earlougher (1977) provided the mathematical model to account for the pressure behavior of a vertically fractured well in an infinite system:
The mathematical model presented in equation 13 by Ehlig-Economides and Economides (1985) was:
On the other hand, the mathematical expression responsible of the dimensionless pressure behavior as proposed by Nutakki and Mattar (1982) (their equation 5), and by Larsen and Hovdan (1987) (their equation 5), following Earlougher´s model was:
In which, the dimensionless time were defined as:
Escobar et al. (2004) found out that none of the above models described the actual pressure behavior of a well in a channel reservoir. Then, they provided new governing equations to account for the dimensionless pressure and dimensionless pressure derivative behaviors during linear and dual linear flow regimes in elongated systems, as follows:
It is well known from fluid mechanics that an additional pressure drop takes place when the direction of the fluid changes in any system. Figure 3 clearly explains this phenomenon in two pipe systems with the purpose of establishing an analogy with a reservoir.
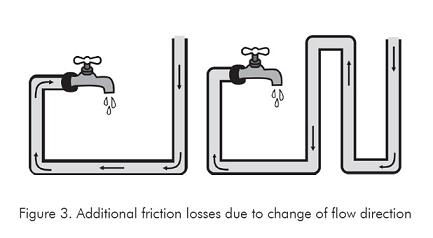
The lower pressure losses are presented in the left-hand system since it possesses less fluid path changes. The fact that the flow direction path changes from radial to linear flow causes an additional pressure drop in a channelized reservoir system.
Escobar et al. (2004) defined the true or total skin factor as:
St = S + SDL + SL (6.a)
If the well is centered along the reservoir, only dual linear flow develops, then Equation 6.a becomes:
St = S + SDL (6.b)
For a off-centered well located inside a closed rectangular reservoir, once dual linear flow vanishes, the parabolic behavior shows up, as explained in detail in the work by Escobar et al. (2005).
St = S + SDL + SPB (6.c)
The dual linear, linear and parabolic geometric skin factors found in channel reservoir as presented by Escobar et al. (2004) and Escobar et al. (2005) were:
It is important to clarify that by the time the work of Escobar et al. (2004) was published; the parabolic flow was not discovered yet, therefore, not included in the oil industry literature. By that time, this flow regime was labeled as “pseudohemispherical flow” since the goal of that work was not characterizing such a flow regime.
The mechanical skin factor, s, which is conventionally estimated by reading the well pressure at time of one hour during the radial flow regime, can be estimated by the TDS technique using equation 2.34 by Tiab (1993). Also, use equation 2.8, reported by Tiab (1993), to calculate reservoir permeability.
ANALYSIS OF VARIABLES IMPACTING THE LINEAR SKIN FACTOR VALUES
Some Colombian reservoirs may possess elongated geometry since they were formed by fluvial deposition especially in the basin of the Magdalena River Valley. Our experience when determining the linear skin factors on some actual field cases has tremendously called our attention. In some cases, we estimated very low linear skin factors, let us say less than 0,01. However, in other cases our estimation of the linear skin factor was so high, even greater than 10. After this, we considered to conduct a study to find out the causes of these huge differences in the values of the linear skin factors, so that, a better well assessment can be taken into account.
Based upon the above considerations, we choose reservoir permeability, reservoir length and the degree of well damage/stimulation as the sensitive variables to affect the value of the linear skin factor.
Impact of reservoir permeability
Using the parameters provided in the first column of Table 1, labeled as Run # 1, we simulated the pressure and pressure derivative behavior of a well for different permeability values of 50, 100, 200, 400 and 800 md. Next, the dual linear skin factor was estimated using Equation 7.b by reading the pressure and pressure derivative values at any time during dual linear flow regime, tDL. Results of these computations are reported in Table 2. It is important to point out that even though the mechanical skin factor was set to zero, the dual linear skin factor is still very high. We also observe in Table 2 that there exists a very small -practically insignificant- reduction of the dual linear factor as the permeability increases.
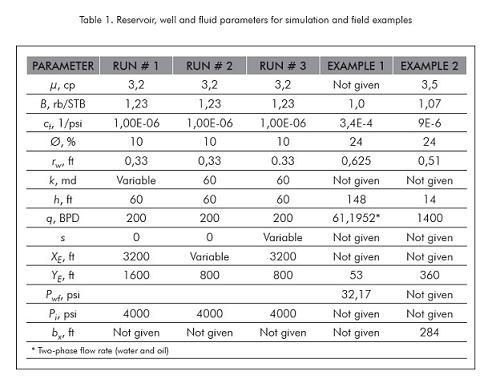
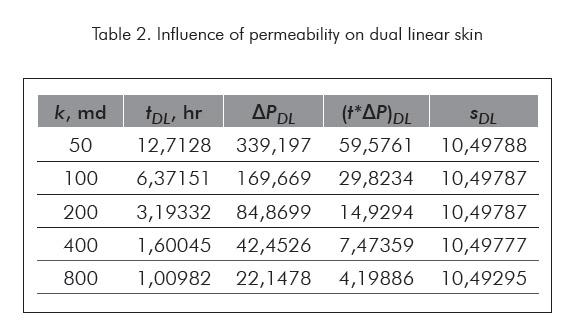
Impact of reservoir length
Since no significant influence of permeability was observed on sDL, the next step was to change the reservoir length. We expected that the effect of reservoir length, XE, could be more significant since the convergence from radial flow to linear flow is more severe as the reservoir becomes narrower. For this purpose, the reservoir and fluid properties of the third column in Table 1 (Run # 2) were used to run the analytical experiments with XE = 1600, 3200, 6400, 12800, 25600, 51200 feet, while YE was kept to 800 feet. This means that the XE/YE ratio of 2, 4, 8, 16, 32, and 64 were employed for the sensitivity analysis.
As for the permeability impact case, Equation 7.b was used to estimate the dual linear skin factor, sDL, for each reservoir length as reported in Table 3. Since the mechanical skin factor was also taken as zero, the dual linear skin factor was so high, too; more than 11. As expected, the higher the XE/YE ratio, the higher the dual linear skin factor increases due to the increase of flow convergence. However, the effect is very small and, for practical purposes, it could be ignored.

Impact of well damage/stimulation
Since not relevant impact of both reservoir permeability and reservoir geometry was obtained in ther former two cases in which we considered the well had zero damage or zero mechanical skin factor, we decided to vary the mechanical skin factor value from -6 to +6. For this particular case, we employed the properties of the fourth column of Table 1, labeled as Run # 3. The simulated pressure and pressure derivative results are reported in Figure 4.
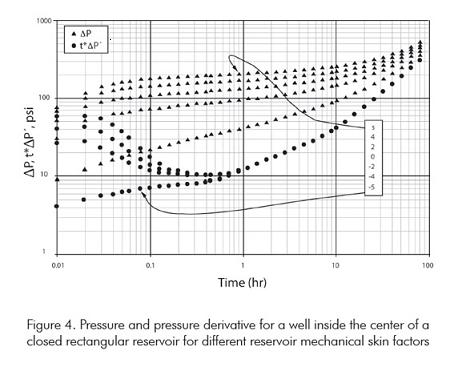
Using equation 2.34 from Tiab (1993), the mechanical skin factor was computed from the pressure and pressure derivative value at any arbitrary time during radial flow, tr, and was found to be in certain agreement with the input values. See results of these calculations in Table 4 at the scalc column. As before, the dual linear skin factor, sDL, was calculated with Equation 7.b using appropriate values of pressure and pressure derivative read at any arbitrary time, tDL, during the dual linear flow regime. Results of these computations are also reported in Table 4. Finally, we computed the total or true skin factor using Equation 6.b as the summation of sassum + sDL.
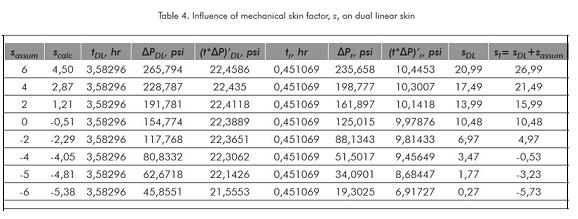
Notice in Table 4 the great impact of the degree of well stimulation on the dual linear skin factor. When the well is stimulated the dual linear skin factor tends to zero. On the other hand, the more severe the well damage, the higher the dual linear skin factor.
Figure 5 was built for comparative purposes. There, we can observe a linear dependence of the dual linear skin factor on the mechanical skin factor. It is then obvious to expect a higher linear dependence of the total skin factor on the mechanical skin factor, as well. It was found out that the total skin factor reduces at a rate of 2,7385 units while the dual linear skin factor reduces at a rate of 1,7385 units as the well becomes more stimulated. It is also advisable to point out that for a zero mechanical skin factor, the dual linear skin factor, sDL, has a value of 10,48. Besides, even though after stimulating the well with a mechanical skin factor s of -2, still a total positive skin factor value remains. From the linear trend equation provided in Figure 5, the well will have zero total damage when the mechanical skin factor is reduced to -6,05.
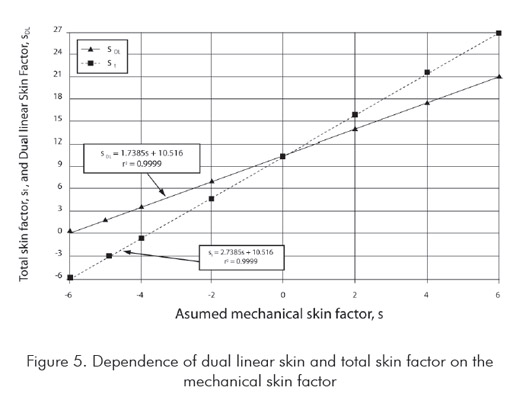
VERIFICATION ON FIELD CASES
It has been found along this article that the degree of damage/stimulation of a well is the key factor responsible for the value of the linear skin factor. We desired to verify this remark on actual field cases, and for that reason, two field cases were taken to follow this observation.
Example 1. A pressure buildup test was run in a Colombian reservoir on the Magdalena River Valley sedimentary basin. Pressure and pressure derivative data are plotted in Figure 6, and fluid, well and reservoir parameters are presented in the fourth column of Table 1.
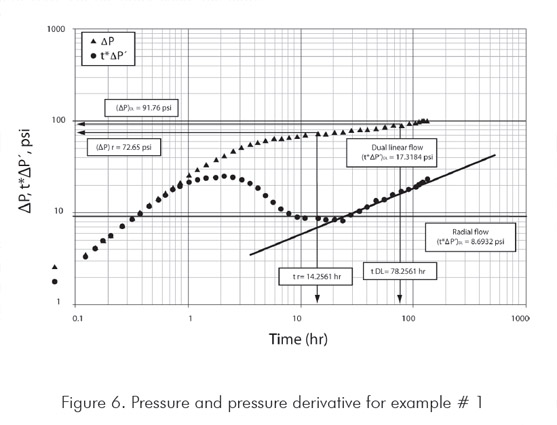
Solution. As seen in Figure 6, once radial flow vanishes, the dual linear flow regime develops. This is formed by two linear flows, one of them occurring along one of the reservoir´s lateral sides and the other along the other side. In other words, isobaric lines flow linearly at opposite sides of the well from the reservoir lateral sides. This flow regime is recognized by a ½-slope line on the pressure derivative curve. As for the example, the dual linear flow is very typical of long reservoirs and masks the single linear flow when the well is centered with respect of the extreme boundaries. It is inferred from the test, that the reservoir has all its boundaries close to flow; therefore, pseudosteady-state is expected to develop once dual linear flow vanishes.
From the pressure and pressure derivative plot, Figure 6, only radial flow and dual linear flow are clearly identified. The following information was read from this plot:

Again, the permeability/viscosity ratio and mechanical skin factor were calculated, respectively, using equations 2.8 and 2.34 taken from Tiab (1993). The dual linear (geometric) skin factor was estimated with Equation 7.b It was obtained k/µ = 3,2568 md/cp, s = 0,8, sDL = 6,61.
This time, the total skin factor is computed with Equation 6.b, resulting to be 7,41. From this field example, we observe that a damage well leads to a high total skin factor, as proposed by the article.
Example 2. Escobar et al. (2004) presented an example of a pressure drawdown test run in a well in a channelized reservoir in the Eastern Planes basin in Colombia. This reservoir has a constant-pressure lateral boundary near the well, therefore, parabolic flow is expected to be developed. Reservoir and well parameters are given in the fifth column of table 1 and pressure and pressure derivative data are given in Figure 7.
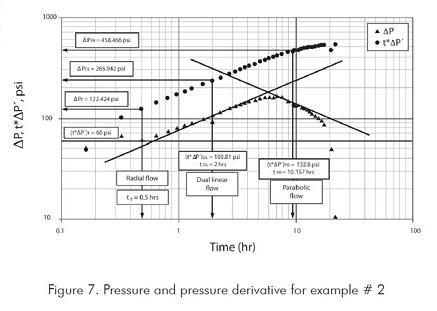
Solution. It is inferred from the test that the well is closer to a constant-pressure lateral boundary; therefore, steady-state flow will dominate the test at later times. From the pressure derivative plot given in Figure 7, we can observe the presence of parabolic flow between, approximately, 7 to 12 hours. This flow regime, characterized by a pressure derivative slope of -½, takes place when the well is near a constant-pressure boundary and the pressure disturbance reaches it. Afterwards, a simultaneous action of the single linear flow on one lateral side of the reservoir and the steady-state, on the other side of the reservoir occurs. We believe that the constant-pressure boundary dominates the transient behavior pressure but its effect is interrupted by the presence of the well, then linear parallel isobaric lines have to be deformed; therefore, the higher pressure drop is seen at the right side of the well at the center of the reservoir. At these points, pressure from the left side is hard to be transmitted to the right side. For more clarification on this issue, the work of Escobar et al. (2005) is recommended to be checked.
As shown in Figure 7, it is observed that radial, dual linear, parabolic and steady state flows are developed. The parabolic flow is due to effect of the constant-pressure boundary once dual linear flow vanishes, and this is well explained by Escobar et al. (2005). The following parameters were read from Figure 7:

Once more, a permeability value of 440,7 md was obtained both from equation 2.8 and a mechanical skin factor, s of -4,53 was estimated with equation 2.34 reported by Tiab (1993). The geometric skin factors, e.g., dual linear skin factor, sDL = 0,44, and the parabolic skin factor, sPB = 0,022, were estimated using Equations 7.b and 7.c, respectively. Then, the total skin factor is -4,07. From this example we verify that, a stimulated well leads to a low dual skin factor. Therefore, the example follows and agrees with the exposed theory. Note: The examples should be worked with more detail using the procedures provided by Escobar et al. (2004).
CONCLUSION
• So far, literature has completely neglected the skin factors due to changes in flow path in elongated reservoirs which leads to an inappropriate evaluation and assessment of well management. The exposed theory and the worked field examples confirmed that as the mechanical skin factor increases, the linear, dual linear and parabolic skin factors contribute with a higher degree to the total well damage/stimulation. Both permeability and reservoir length have a very low influence on the dual linear skin factor. We expect the same influence for the linear and parabolic skin factors.
REFERENCES
Earlougher, R. C., & Jr. (1977). Advances in well test analysis. Monograph Series, 5, SPE, Dallas, TX. [ Links ]
Ehlig-Economides, C., & Economides, M. J. (1985). Pressure transient analysis in an elongated linear flow systems, SPEJ, 839-847. [ Links ]
Escobar, F. H., Saavedra, N. F., Hernandez, C. M., Hernandez, Y.A., Pilataxi, J.F., & Pinto, D.A. (2004). Pressure and pressure derivative analysis for linear homogeneous reservoirs without using type-curve matching. 28th Annual SPE International Technical Conference and Exhibition, Abuja, Nigeria. SPE 88874. [ Links ]
Escobar, F. H., Muñoz, O. F., Sepulveda, J. A., & Montealegre, M. (2005). New finding on pressure response in long, narrow reservoirs. CT&F – Ciencia, Tecnología y Futuro. 3 (1), 51-160. [ Links ]
Larsen, L., & Hovdan, M. (1987). Analyzing well test data from linear reservoirs by conventional methods. 62nd Annual Technical Conference and Exhibition of the Society of Petroleum Engineers, Dallas, TX. SPE 16777. [ Links ]
Massonet, G. J., Norris, R. J., & Chalmette, J-C. (1993). Well test interpretation in geologically complex channelized reservoirs. 68th Annual Technical Conference and Exhibition of the Society of Petroleum Engineers, Houston, TX. SPE 26464. [ Links ]
Mattar, L. (1997). Derivative analysis without type curves. 48th Annual Technical Meeting of the Petroleum Society, Calgary, Alberta, Canada, SPE 97-51. [ Links ]
Nutakki, R., & Mattar, L. (1982). Pressure transient analysis of wells in very long narrow reservoirs. 57th Annual Fall Technical Conference and Exhibition of the Society of Petroleum Engineers, Dallas, TX. SPE 11221. [ Links ]
Tiab, D. (1993). Analysis of pressure and pressure derivative without type-curve matching: 1- skin and wellbore storage. Paper presented at the Production Operations Symposium, Oklahoma City, OK. SPE 25426, 203-216. Also, J. of Petroleum Scien. and Engineer., (1995), 12: 171-181. [ Links ]
Wong, D.W., Mothersele, C. D., Harrington, A. G., & Cinco-Ley, H. (1986). Pressure transient analysis in finite linear reservoirs using derivative and conventional techniques: field examples. 61st Annual technical Conference and Exhibition of the Society of Petroleum Engineers, New Orleans, LA. SPE 15421. [ Links ]