Services on Demand
Journal
Article
Indicators
-
Cited by SciELO
-
Access statistics
Related links
-
Cited by Google
-
Similars in SciELO
-
Similars in Google
Share
CT&F - Ciencia, Tecnología y Futuro
Print version ISSN 0122-5383
C.T.F Cienc. Tecnol. Futuro vol.5 no.2 Bucaramanga Jan./June 2013
IMPROVEMENT OF LIPID PRODUCTIVITY ON Chlorella vulgaris USING WASTE GLYCEROL AND SODIUM ACETATE
MEJORAMIENTO DE LA PRODUCTIVIDAD LIPÍDICA EN Chlorella Vulgaris UTILIZANDO GLICEROL RESIDUAL Y ACETATO DE SODIO
Laura-Lucía Estévez-Landazábal1, Andrés-Fernando Barajas-Solano*1, Crisóstomo Barajas-Ferreira1 and Viatcheslav Kafarov1
1Universidad Industrial de Santander, Bucaramanga, Santander, Colombia
e-mail: cisyc@uis.edu.co
(Received: Jul. 16, 2012; Accepted: Apr. 24, 2013)
* To whom correspondence should be addressed
ABSTRACT
Although microalgae have potential as a raw material for biodiesel production it is necessary to increase biomass and lipids productivity. One way to achieve this goal is the implementation of mixotrophic cultures and the regulation of carbon/nitrogen ratio. The present work aims to improve the productivity of biomass and lipids in Chlorella vulgaris UTEX 1803 using waste glycerol from biodiesel production (1, 5 and 10% v/v) and sodium acetate (5, 10 and 20 mM) as carbon sources, with modification of the initial concentration of nitrogen (1.02, 1.47 and 2.94 mM de NaNO3). All experiments were performed at 23±1ºC, with light-dark cycles of 12:12h during five days.
In biomass production, a significant increase was achieved (80% higher that cultures without modification). Lipid productivities were also found 2.83 and 3.5 times greater than control.
Results show the possibility of increasing the production of biomass and lipids by applying the carbon/nitrogen ratio, using waste glycerol. This opens up great possibilities for the re-use of this residue, thus increasing the sustainability of the process in general. Also, it has been proved that -due to its low cost- carbon/nitrogen ratio using sodium acetate is an interesting alternative.
Keywords: Mixotrophic cultures, Microalgae, Biomass, Lipids, Biodiesel.
RESUMEN
Aunque las microalgas poseen potencial como materia prima para la producción de biodiesel, es necesario aumentar la productividad de biomasa y lípidos. Una forma de lograrlo es con la implementación de cultivos mixotróficos y la regulación de la relación carbono/nitrógeno. El presente trabajo tiene como objetivo mejorar la productividad de biomasa y lípidos en Chlorella vulgaris UTEX 1803 usando como fuentes de carbono glicerol residual de la producción de biodiesel (1, 5 y 10% v/v) y acetato de sodio (5, 10 y 20 mM), y modificando la concentración de nitrógeno inicial (1.02, 1.47 y 2.94 mM de NaNO3). Las condiciones de cultivo fueron: 23±1ºC, ciclos luz-oscuridad 12: 12h durante periodos establecidos de cinco días.
Se logró un aumento significativo en la producción de biomasa (80% mayor que cultivos sin modificación). Se encontraron productividades lipídicas 2.83 y 3.5 veces mayores que el control.
Los resultados demuestran la posibilidad de aumentar la producción de biomasa y lípidos aplicando la relación carbono/nitrógeno empleando el glicerol residual como posibilidad de reciclo de este residuo, aumentado así la sostenibilidad del proceso en general; se determinó además que el acetato de sodio puede ser utilizado como una alternativa económica para controlar la relación carbono/nitrógeno.
Palabras clave: Cultivo Mixotrófico, Microalgas, Biomasa, Lipidos, Biodiesel.
RESUMO
A inda que as microalgas possuam potencial como matéria-prima para a produção de biodiesel, é necessário aumentar a produtividade de biomassa e de lipídios. Uma forma de consegui-lo é com a implantação de cultivos mixotróficos e com a regulação da relação carbono/nitrogênio. O presente trabalho tem como objetivo melhorar a produtividade de biomassa e de lipídios em Chlorella vulgaris UTEX 1803 usando como fontes de carbono glicerol residual da produção de biodiesel (1, 5 e 10% v/v) e de acetato de sódio (5, 10 e 20 mM) e, modificando a concentração de nitrogênio inicial (1.02; 1.47 e 2.94 mM de NaNO3). As condições de cultivo foram: 23±1ºC, ciclos luz-escuridão 12: 12h durante períodos estabelecidos de cinco dias.
Conseguiu-se um aumento significativo na produção de biomassa (80% maior que cultivos sem modificação). Encontraram-se produtividades lipídicas 2.83 e 3.5 vezes maiores que o controle.
Os resultados demonstram a possibilidade de aumentar a produção de biomassa e de lipídios aplicando a relação carbono/nitrogênio empregando o glicerol residual como possibilidade de reciclagem deste resíduo, aumentado assim a sustentabilidade do processo em geral; além disso determinou-se que o acetato de sódio pode ser utilizado como uma alternativa econômica para controlar a relação carbono/nitrogênio.
Palavras-chave: Cultivo Mixotrófico, Microalgas, Biomassa, Lipídios, Biodiesel.
1. INTRODUCTION
In recent years, alternative fuels have become very important due to the rapid depletion of oil reserves and the deterioration of ecosystems (Song, Fu & Shi, 2008; Meng et al., 2008; Kalia & Purohit, 2008). With in the proposed organisms for the production of biodiesel, microalgae are emerging as a viable alternative. Since some species have high lipid content transesterificables, they don't compete with food production. These species can use non-arable lands instead of large tracts of land. (Chisti, 2007; Williams, 2007; Song et al., 2008). The biodiesel obtained from these microorganisms is biodegradable, renewable, does not contribute to the release of sulfur into the atmosphere and generates less gaseous pollutants than conventional fossil fuels (Vicente, Martínez & Aracil, 2004; Pinto et al., 2005). In addition, the burning of the latter is associated with global warming (Gavrilescu & Chisti, 2005).
Currently, the most common method for microalgae culture is the autotrophic culture. In this, the cell uses light as energy source and CO2 as the carbon source (Pérez-García, Escalante, de Bashan & Bashan, 2011). Microalgae cultures used for production of biomolecules biofixation in carbon dioxide have the potential not only to reduce costs in obtaining value-added products (biofuels, dyes, proteins, vitamins), but also to compensate carbon emissions (Heredia-Arroyo, Wei & Hu, 2010).
Although microalgae can efficiently use light, growth in large scale cultures is slow due to the limited penetration of light caused by the thickness of the water column and high cell densities (Suh & Lee, 2003). In view of these disadvantages associated with autotrophic culture, a viable alternative for some species is the use of heterotrophic cultures, replacing CO2 fixation with dissolved organic carbon sources in the culture medium.
Heterotrophy is defined as the use of organic compounds for cell growth (Droop, 1974). A variant of this scheme is the mixotrophic growth, in which CO2 and the organic carbon source are simultaneously assimilated (Lee, 2004). However, to make that cultivation economically feasible, it requires a source of inexpensive carbon. It is also important to acquire knowledge on which are the most favorable concentrations (Jeon, Cho & Yun 2006), as certain ones can increase growth rates and obtain biomolecules (Chisti, 2008; Liang, Sarkany & Cui, 2009), while others are inhibitory or toxic to algae (Jeon et al., 2006).
Mixotrophic culture of C. vulgaris integrates some advantages of heterotrophic and phototrophic configurations (Lee, 2001), while overcoming the difficulties associated with these two types of cultures (Lee, Ding, Hoe & Low, 1996). The benefits are associated with a significant increase in growth rate and productivity due to high organic carbon incorporation with in cell, particularly in the formation of metabolites such as lipids, polysaccharides and proteins (Richmond, 1986). This is particularly important, as increases of up to five times have been reported compared with autotrophic cultures of microalgae (Syrett, Bocks & Merret, 1964). Another advantage of mixotrophic cultures is the use of light, which induces and regulates the production of high value macromolecules such as pigments (Lee & Zhang, 1999) and fatty acids (Li, Xu & Wu, 2007). It also reduces CO2 production compared to heterotrophic cultures (Goulding & Merrett, 1966).Another plus is the wide variety of carbon sources available for use in mixotrophic cultures, such as acetate, glycerol, ethanol, organic acids, sugars, cassava starch hydrolyzate, hydrolyzed corn starch and byproducts of industrial processes as alpechín (Loera-Quezada & Olguín, 2010). It has been shown that C. vulgaris can oxidize and utilize acetate as carbon source and energy for growth in the dark (Huang et al., 2010), which causes changes in the tricarboxylic acid cycle, because the Acetyl -CoA is not derived from pyruvate but form the acetate ion. The biochemical mechanism that allows C. vulgaris to grow by converting acetate, is known as glyoxylate cycle (Goulding & Merrett, 1966).
The cost of organic carbon source (usually glucose) is high compared with all the necessary nutrients (Liang et al., 2009). To overcome this difficulty, an economical source should be found. Glycerol is the by-product of the transesterification reaction between fats and oils and alcohol in the production of biodiesel (Schenk et al., 2008). The crude glycerol obtained is impure and of little economic value (Pyle, 2008). Given the current glut of crude glycerol in the market, mainly due to the global biodiesel industry booming, various studies on alternative uses have been developed (Pyle, 2008): combustion (Johnson & Taconi, 2007), composting, animal feed (Cerrate et al., 2006), thermochemical conversions (Alhanash, Kozhevnikova & Kozhevnikov, 2008) and biological conversion methods (Holm, Lomborg, Oleskowicz & Ebensen, 2008). Very few studies have used glycerol as a carbon source for microalgae growth (Pyle, Garcia & Wen, 2008; Liang et al., 2009; Heredia-Arroyo et al., 2010). These studies are largely focused on the ability of algae to metabolize this compound, but there is no information about which are the most appropriate concentrations for the cultivation of microalgae, and which are the effects of this compound in the production of biomolecules (Pyle et al., 2008).
Acetate can also be used as carbon source in microalgae mixotrophic cultures, and the incorporation of acetate is a process dependent on both anabolic and catabolic metabolism (Bouarab, Dautab & Loudikia, 2004). Research on microalgae growth of different species in the presence of acetate have been conducted for several decades (Qiao & Wang, 2009). However, its use is problematic because its effect is concentration dependent (Hagen, Grünewald, Xylander & Rothe, 2001). Some authors found that concentrations above 1 g/L or less may cause growth inhibition due to algae intoxication (Jeon et al., 2006), while lower concentrations stimulate the growth and lipid accumulation (Qiao & Wang, 2009; Degrenne et al., 2010). Other authors found the effect of acetate on growth minimal and easily ignored (Hu & Gao, 2003).
In the present study, we examined on lab scale the influence of residual glycerol and acetate in the growth and lipid deposition in C. vulgaris, in order to find an application in the production of different types of fuel, allowing a recycling process in which biomass is generated with different applications.
2. EXPERIMENTAL DESIGN
Culture Methods
Chlorella vulgaris UTEX 1803 was acquired from the strain collection of the University of Texas (Austin, Texas, USA); the algae was cultured on Bold Basal Media which composition in mg/L is: NaNO3 (2.94), MgSO4.7H2O (3.04x10-1) NaCl (4.28x10-1), K2HPO4 (4.31x10-1), KH2PO4 (1.29), CaCl2.2H2O (1.70x10-1) and micronutrients (mg/L) ZnSO4.7H2O (3.07x10-2), MnCl2.4H2O (7.28x10-3), MoO3(4.93x10-3), CuSO4.5H2O (6.29x10-3), Co(NO3)2.6H2O (1.68x10-3), H3BO3 (1.85x10-1), EDTA(1.71x10-1), KOH (5.53x10-1), FeSO4.7H2O (1.79x10-2).
Cylindrical airlift reactors with an internal diameter of 14 and 35 cm height with a culture volume of 2 L were used. The reactors were attached to an aeration system with flow of 2 L/min.
Waste Glycerol Production
The glycerol was obtained following the protocol for biodiesel production of Plata, Kafarov and Moreno (2010). Using a 2 L sealing glass reactor equipped with a reflux condenser, a temperature controller and a magnetic stirrer set at 600 rpm. The reactor was filled with 250 g of palm oil and heated. When the expected temperatures were reached, sodium hydroxide and methanol were added to the oil. After cooling, the layer of biodiesel and residual glycerol were separated by decantation.
Pre-treatment of Waste Glycerol
After recovering the waste glycerol, a pretreatment was performed following the protocol of Chi et al. (2007), which involves mixing the glycerol with distilled water in a 1:4 ratio (v/v), adjusting the pH to 6.5 with hydrochloric acid, to convert the fraction of soluble soaps and insoluble free fatty acids, followed by a phase separation by decantation.
Waste Glycerol Characterization
For the characterization of residual glycerol (glycerol and methanol) a high efficiency liquid chromatograph (Agilent Technologies 1100 series) was used, coupled to a refractive index detector Agilent Technologies 1200, using a column SUPELCOGELTM C-610 H. 10 mL of sample was vacuum filtered using qualitative filter paper, diluted and homogenized for 10 min in an ultrasonic bath. Then, the sample was refiltered using syringe filters to Olim Peak of Polyvinylidene fluoride (PVDF) of 0.45 μm. To determine the amount of ash, 2 g of crude glycerol (in triplicate) were placed in pots and previously dried for 2 h at 120°C. The samples were calcined at 750°C for 3h, then allowed to cool in a desiccator and then weighed.
Carbon/Nitrogen Ratio
In order to improve the productivity of biomass and lipids, different carbon/nitrogen ratios under mixotrophic conditions were tested. Industrial grade sodium acetate (Leon laboratories, Bucaramanga) and waste glycerol previously pretreated were used as carbon sources.
For each of these, an experiment design was performed, varying the concentrations of both sodium nitrate and carbon source (Tables 1 and 2).
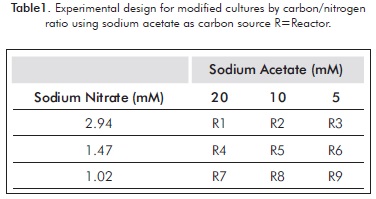
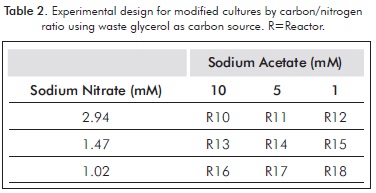
Each experiment (including the control) were performed in triplicate for five days, in cycles of light: dark 12:12 and a filtered air flow of 2 L/min, using membrane filter of 0.2 microns; the initial pH, temperature and light intensity were 6.5±1, 23±1°C and 480±1 µmol photons /m2/s. An inoculum volume sufficient to achieve initial cell concentration of about 0.4 g/L (dry weight) was added. This was achieved by controlling the initial optical density (about 0.8 absorbance at 500 nm), by using a spectrophotometer (300 Spectroquant Merck).
Biomass Quantification
For measurements of biomass concentration, a sample of 6 mL of each culture were diluted in distilled water in a ratio of 1:8. The optical density was measured using a spectrophotometer (Spectroquant® Pharo 300) at a wave length of 500 nm (measurement typical values are between 0.1 to 0.7). The Optical Density (OD) was correlated to the amount of biomass (g/L) in accordance with Equation 1, which has a correlation coefficient of 0.931:

Lipid Extraction
The extraction was conducted following the modified Soxhlet with hexane protocol by González-Delgado and Kafarov (2011), which consists on drying 10 g of biomass at 200°C for 12 h. After this process, the biomass is homogenized using a mortar and again subjected to drying at 125°C for 3 h. Finally, on a filter paper (2 µm) 5 g of homogenized biomass enters the soxhlet. The process lasted 16 h.
Percentage Lipid
Commonly, the lipid content is reported as a percentage of dry weight (Griffiths & Harrison, 2009). Lipid percentage was calculated by dividing the weight of the lipid and dry weight of biomass.
Lipid Volumetric Productivity
A parameter commonly used to compare species and culture methods for the production of biodiesel is lipid volumetric productivity (Nascimento et al., 2013), which summarizes the concentration of biomass and lipid content (Griffiths & Harrison, 2009), allowing to contrast microalgae with different lipid percentages and growth rates. As a result, one could know which will produce a greater amount of lipids at the end of the crop.
In this project, the lipid productivity is reported as milligrams of lipid per liter obtained at the end of the culture period (5 days), calculated by multiplying the biomass concentration per the lipid percentage (Griffiths & Harrison, 2009).
Nitrogen and Phosphorous Quantification Methods
Nitrogen (NO3-N) was quantified by the ultraviolet spectrophotometric method. In the case of phosphorus (PO4-P), we used the vanadomolibdophosphoric acid colorimetric method (Clesceri, Greenberg & Eaton, 1999).
Nitrogen (NO3-N) by Ultraviolet Spectrophotometric Method
1 mL of HCl 1 N was added to 50 mL cells-Free media cells and stirred vigorously. After 10 minutes, the sample was read at 220 and 275 nm, to determine the interference due to dissolved organic material. The values obtained were replaced in Equation 2, which corrects the absorbance noise due to organic matter (Clesceri, Greenberg & Eaton, 1999):

Phosphorus (PO4 -P) by Vanadomolibdophosphoric Acid Colorimetric Method
10 mL of Vanadate-molybdate reagent and 10 mL of distilled water were added to 30 mL cells-Free media cells. After 10 minutes, the sample was read in a spectrophotometer at a wavelength of 470 nm. Absorbance was obtained using the concentration directly from the standard curve (Clesceri, Greenberg & Eaton, 1999).
Statistical Analysis of Data
A priori trials of normality and homogeneity of variance were performed, according to the Kolmogorov Smirnov and Levene tests, respectively (Zar, 1999). These were done using PAST 2.15 (Hammer, Harper & Ryan, 2001) and STATISTICA 10 (StatSoft Inc., 2011).
To identify whether there were significant differences in biomass production, we used a factorial ANOVA, trying different concentrations of waste glycerol and acetate as factors. In cases where the data did not meet normality or homogeneity of variances, the nonparametric Kruskal-Wallis test was applied. Both the KW and the ANOVAS were performed on STATISTICA 10 (StatSoft Inc., 2011). After ANOVA, the Tukey posteriori comparisons test was performed, for variables that showed significant differences in the ANOVA (p> 0.05).
3. RESULTS
Waste glycerol characteristics obtained on a laboratory scale are described in Table 3:
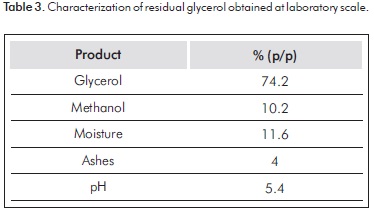
The final concentrations of each of the treatments using sodium acetate as a carbon source after five days of culture can be seen in Figure 1. The highest amount of biomass obtained was 3.4 g/L in the treatment with 20 mM acetate and 2.94 mM nitrate. Cultures with 20 mM acetate had higher biomass concentrations than other cultures; treatments with lower concentration of nitrogen (1.02 mM) had final concentrations of biomass under the control culture (without 2.94 mM acetate and nitrate). In general, the final biomass concentration was positively correlated with the initial concentration of nitrate, indicating the flattering effect of nitrogen in the growth reported in the literature.
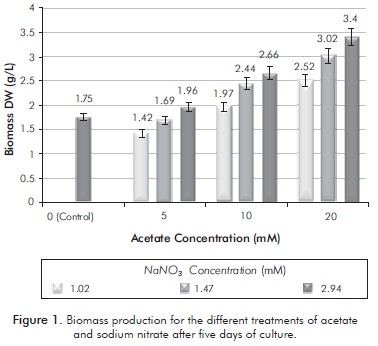
In glycerol-enriched cultures (see Figure 2), the highest concentration of biomass obtained at the end of five days was 3.15 g/L with treatment of 1% v/v glycerol: 2.94 mM of nitrate. On the other hand, cultures treated with 1 and 5% v/v did not show significant statistic differences (p = 0.06830) in the final biomass concentration with the control culture, or with acetate-treated cultures.
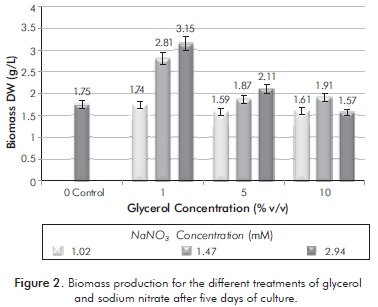
Lipid Production
Total biomass, percentage of lipids produced after five days of culture and percentage of nitrogen and phosphorus consumed for acetate treatments are shown in Table 4.
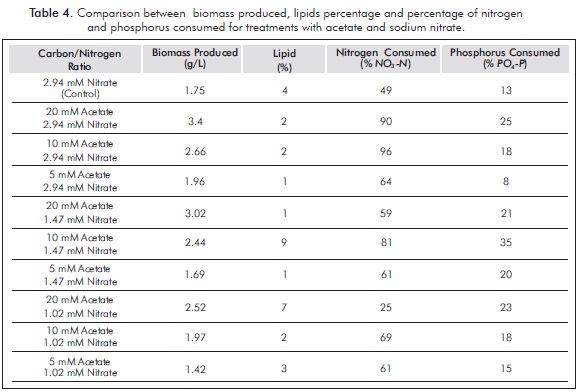
The treatments in which nitrogen intake was high showed high initial concentrations of nitrate, thus allowing elevated growth rates, rapid cell division and low storage metabolites. In the case of treatment of 20 mM sodium acetate and 2.94 mM nitrate, the biomass production was 3.4g/L. However, the concentrations of nitrate resulted in a lower lipid productivity (in this case only 2%). Furthermore, the best lipid productivities are cultures where the initial concentration of nitrogen is low. For the treatment of 10 mM sodium acetate: 1.47 mM nitrate, a 9% lipids and biomass production of 2.44 g/L was obtained. These results confirm that high concentrations of nitrogen favor the production of lipids, which is consistent with results of research such as Lv et al. (2010).
In the case of phosphorus, it was found that those treatments with high intakes of phosphorus (23 to 35%) had the highest percentages of lipids. It was also found that treatment with lower consumption of phosphorus (up to 8%) had low lipid percentages.
The percentage of lipid content with glycerol cultures can be seen in Table 5. Treatments limited by nitrate concentrations were those with higher concentrations of lipids in their cells. Glycerol showed a positive effect on lipid accumulation, as glycerol cultures all had higher lipid concentrations to culture control. It is notorious that neither in cultures with glycerol, nor in cultures with acetate, the highest percentage of lipids corresponds to high values in nitrogen.
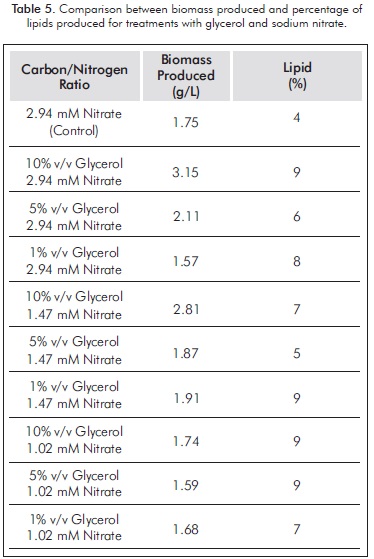
4. DISCUSSION
Table 6 shows the best results in both treatments for the production of biomass and lipids. It can be seen that the control culture was not the best in biomass concentration or lipid levels. Therefore, neither was the best in total lipid productivity, since the latter is related to the concentration of biomass and lipids (Liang et al., 2009). The highest concentrations of final biomass produced by the best treatments differed very little, and both were achieved by adding the highest nitrate concentration used (2.94 mM).
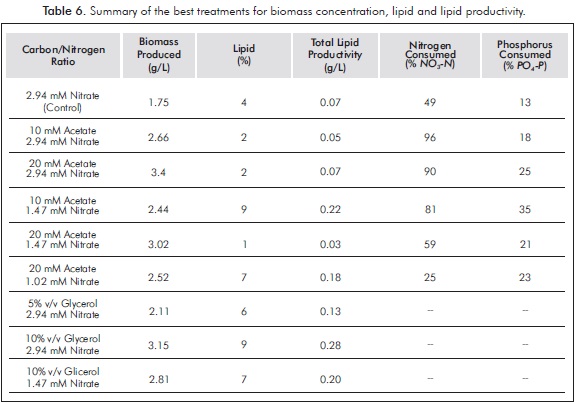
This study shows that C. vulgaris UTEX 1803 can grow successfully in mixotrophic conditions. Higher biomass concentrations were achieved using 20 mM concentrations of acetate and 10% v/v glycerol, showing a significant difference to the other treatments and the control culture (photoautotrophic) and a positive effect on the two carbon sources used, for potential application on a larger scale. The results are consistent with previous experiments in mixotrophic cultures of C. vulgaris, which obtained higher concentrations of biomass under mixotrophic conditions (Liang et al., 2009; Heredia-Arroyo, Wei, Ruan & Hu, 2011). Doucha and Livansky (2011) managed to obtain concentrations of biomass as high as117 g/L. These authors used in their cultures of C. vulgaris solutions of 500 g/L glucose with a lipid content of 9.7% which is similar to that obtained in the best treatments evaluated in this study (9%). However, this is considered unacceptable since there should be no link between the production of biomass for biofuels and food.
It should be noted that these authors did not evaluate different carbon/nitrogen ratios. Besides, the cost of glucose as raw material is too high to be feasible. Liang et al. (2009) report -as this study does-a significant increase in the percentage of fat in C. vulgaris, due to carbon/nitrogen ratio. However, they only obtained 2 g/L in 6 days using glucose on mixotrophic cultures. This value is smaller than those reported in the present study. Commonly, low final biomass concentration is the obstacle in microalgae cultures to make the process commercially available, considering the cost of collection and separation (Heredia-Arroyo et al., 2011). A factor which could be attributed to those results is the initial cell concentration, or metabolic differences between strains, due to the fact that the effect of the substrate concentration is dependent on the strain and the strain used in this study may have better adaptation to glycerol and acetate that the strain used by Liang et al. (2009).
Wang, Fu and Pei (2012), worked on the mixotrophic culture of the oilseed Phaeodactylum tricornutum, obtaining biomass maximum concentrations of 1.16 g/L. Its high productivity lipids were similar to those obtained from this study. Liang et al. (2009) used sodium acetate as a carbon source in concentrations of 10 g/L to obtain 1.0 g/L after 6 days of culture. They also reported that mixotrophic cultures with glucose obtained 1.6 g/L of biomass after 6 days, being these levels lower than those obtained in this study. However, we must note that they used a different strain of C. vulgaris, and worked with different sources of nitrate without modification on the initial concentration. It is possible to highlight the importance of the variation of the concentration of nitrogen as a necessary step in changing the culture medium.
In contrast to the work of Liang et al. (2009), in this study the concentrations of 1 to 5% v/v of glycerol produced greater amounts than they report, but the highest productivity of biomass crops were obtained with concentrations of 10% v/v glycerol, while he found that this concentration is inhibitory. Keep in mind that the residual glycerol has a wide range of purity that can be attributed to various purification methods used by the manufacturers of biodiesel and the different raw materials used in the production of biofuels (Pyle, 2008). Besides the above, they start cultivation with an inoculum of lower concentration to that used in the project, and that the inhibitory effect of organic carbon sources among strains is specific, so it is necessary to evaluate each strain individually (Liang et al., 2009).
Each of the papers mentioned above do not take into account the carbon/nitrogen ratio, they relate only productivities of biomass and lipids with different concentrations and sources of carbon sources, ignoring that the metabolism of carbon and nitrogen are connected because they share organic carbon and energy supplied by the electron transport chain, the tricarboxylic acid cycle, respiration via glycolys is fixed carbon or CO2 fixation and photosynthetic electron transport (Huppe & Turpin, 1994).
Another work to compare with is the one of Lv et al. (2010), in which autotrophic cultures of C. vulgaris obtained biomass and lipid productivities close to this study. This is due to the correlation between the influence of the concentration of nitrates and CO2 with the growth and bio-chemical compositions of the biomass produced. They concluded that lipid productivity can be increased simultaneously increasing cell productivity and lipid content in microalgae.
However this can sometimes be a challenge, because several factors regulate both growth and lipid production in microalgae: differences in species and strains studied, including procedures such as variations in cycles of light, temperature, pH conditions, salinity, and the initial cell concentration of microalgae nutritional history can lead to different results (Hu, 2004). Therefore, various conditions in the crop must be taken into account to successfully increase the productivity to be obtained.
Effect of Mixotrophic Growth on Lipid Content of Biomass
According to Huang et al. (2010) microalgae culture conditions influence the final chemical composition and the tendency to accumulate various metabolites; for example, nutrient limitation acts as an efficient ambient pressure to increase the accumulation of lipids (Khozin-Goldberg & Cohen, 2006; Rodolfi et al., 2009). In the genus Chlorella, there are some strains that under nitrogen limitation accumulate large amounts of starch, where as others accumulate neutral lipids (Richmond, 1986).
It was found that glycerol can be used as substrate for lipid accumulation, which is consistent with a study of Heredia-Arroyo et al. (2010). In C. protothecoides, it was also found that acetate -despite having the potential to be used for production of lipids- by itself does not exert a positive influence on the deposition of lipids. It was also found that by adjusting the carbon/nitrogen ratio it is possible to regulate the uptake of certain nutrients, and that up to 55% of carbon is used for nitrogen assimilation (Huppe &Turpin, 1994).Another important factor is the effect of phosphorus in the synthesis of lipids, mainly in the phospholipid synthesis (Williams & Laurens, 2010).
In Chlorella, phospholipids are synthesized under the presence of light and inorganic Phosphate (iP) (Lodish et al., 2005). Generally, phospholipid synthesis is carried out when the fatty acyl-CoA are esterified with a glycerol backbone and form phosphatidic acid, whose two hydrocarbon chains anchored the molecule to the membrane, and a phosphatase converts phosphatidic acid in diacyl glycerol (Lodish et al., 2005). Subsequently, a polar head is transfered, as phosphorylcholine from CDP-choline to the hydroxyl group is exposed. Finally, flipases proteins catalyze the movement of phospholipids from the cytosolic leaflet of the membrane, in which they are initially formed, towards flake exoplasmatica (Lodish et al., 2005).
As noted, the fatty acids are key components of phospholipids. However, their biosynthesis and ratio vary according to the kind of microalgae (Chen, Jiang & Chen, 2007).
5. CONCLUSIONS
-
Proper regulation of the amount of carbon (in this case residual glycerol or sodium acetate) and nitro-gen not only can increase biomass production, but also promote the deposition of certain metabolites such as lipids. In the present study, the residual glycerol enriched cultures -or those enriched with acetate-performed better in biomass production than in autotrophic cultivation, producing up to 2 times more biomass (from 1.75 to 3.4 g/L for the carbon/ nitrogen ratio 20 mM acetate sodium: 2.94 mM ni-trate and 3.15 g/L for the carbon/ nitrogen ratio10% v/v glycerol residual: 2.94 mM nitrate) after five days of culture. The higher percentages of lipids were obtained for treatment with 10 mM sodium acetate: 1.47 mM nitrate and 10% v/v glycerol: 2.94 mM nitrate, exceeding the concentration of the control culture up to 2 times (4 -9%). In turn, these cultures showed the highest productivity of total lipid increasing to 3 times (from 0.07 to 0.22 and 0.28 g/L respectively), compared to the control after five days of culture.
-
Mixotrophic cultivation of C. vulgaris using glycerol -which is a residue from the production of biodiesel-, or sodium acetate -a compound that can arise from economic biohydrogen production- is an approach with excellent possibilities for the production of biomass and fabrication of biofuel. This is due to the significant increase in lipid productivity, without using expensive compounds or compromising food sources such as glucose, and thus increasing the overall sustainability of the process.
ACKNOWLEDGEMENTS
The authors would like to thank the Ministry of Agriculture and Rural Development for its support through project 2008D32006-6710 "Bioprospecting of Colombian Microalgae for Biodiesel Production", Ecopetrol S.A. - Instituto Colombiano del Petróleo (ICP), and the Departamento Administrativo de Ciencia, Tecnología e Innovación COLCIENCIAS, Colombia for its Francisco José de Caldas scholarship program to support national Phd doctorates. Also, the funding for the project to create and strengthen a transfer network of knowledge and technology between the U.S. and Colombia to develop biorefinery processes to obtain biofuels.
REFERENCES
Alhanash, A., Kozhevnikova, E. F. & Kozhevnikov, I. V. (2008). Hydrogenolysis of glycerol to propanediol over Ru: Polyoxometalate bifunctional catalyst. Catal. Lett., 120: 307-311. [ Links ]
Bouarab, L. Dautab, A. & Loudiki, M. (2004). Heterotrophic and mixotrophic growth of Micractinium pusillum fresenius in the presence of acetate and glucose: Effect of light and acetate gradient concentration. Water Res., 38(11), 2706-2712. [ Links ]
Cerrate, S., Yan, F., Wang, Z., Coto, C., Sacakli, P. & Waldroup, P. W. (2006). Evaluation of glycerine from biodiesel production as a feed ingredient for broilers. Int. J. Poult. Sci., 5(11), 1001-1007. [ Links ]
Chen, G. Q., Jiang, Y. & Chen, F. (2007). Fatty acid and lipid class composition of the eicosapentaenoic acid-producing microalga, Nitzschialaevis. Food Chemistry, 104(4), 1580-1585. [ Links ]
Chi, Z., Pyle, D., Wen, Z., Frear, C. & Chen, S. (2007). A laboratory study of producing docosahexaenoic acid from biodiesel-waste glycerol by microalgal fermentation. Proc. Biochem., 42(11), 1537 - 1545. [ Links ]
Chisti, Y. (2007). Biodiesel from microalgae. Biotechnol. Adv., 25: 294 -306. [ Links ]
Chisti, Y. (2008). Biodiesel from microalgae beats bioethanol. Trends Biotechnol., 26(3), 126 -131. [ Links ]
Clesceri, L. S., Greenberg, A. E. & Eaton, A. D. (1999). Standard methods for examination of water and wastewater. 20th ed. Washington, DC: American Public Health Association. [ Links ]
Degrenne, B., Pruvost, J., Christophe, G., Cornet, J., Cogne, G. & Legrand, J. (2010). Investigation of the combined effects of acetate and photobioreactor illuminated fraction in the induction of anoxia for hydrogen production by Chlamydomonas reinhardtii. Int. J. Hydr. Energ., 35(19), 10741-10749. [ Links ]
Doucha, J. & Livansky, K. (2011). Production of high-density Chlorella culture grown in fermenters. J. Appl. Phycol., 24(1), 35-43. [ Links ]
Droop, M. R. (1974). Heterotrophy of carbon. In: Stewart, W. D. P. (Ed.), Algal Physiology and Biochemistry. Oxford: Blackwell Scientific. 530-559. [ Links ]
Gavrilescu, M. & Chisti, Y. (2005). Biotechnology-a sustainable alternative for chemical industry. Biotechnol. Adv., 23(7-8), 471-99. [ Links ]
González-Delgado, A. & Kafarov, V. (2011). Microalgae based biorefinery: Issues to consider. CT&F -Ciencia, Tecnología y Futuro. 4(4), 5-21. [ Links ]
Goulding, K. & Merrett, M.(1966). The photometabolismof acetate by Chlorella pyrenoidosa. J. Experimen. Bot., 17(4), 678-689. [ Links ]
Griffits, M. J. & Harrison, S. T. (2009). Lipid productivity as a key characteristic for choosing algal species for biodiesel production. J. Appl. Phycol., 21(5), 493-507. [ Links ]
Hagen, C., Grünewald, K., Xyländer, M. & Rothe, E. (2001). Effect of cultivation parameters on growth and pigment biosynthesis in flagellated cells of Haematococcus pluvialis. J. Appl. Phycol. 13(1), 79-87. [ Links ]
Hammer, Ø., Harper, D. & Ryan, P. (2001). PAST. Paleontological statistics software package for education and data analysis. Palaeontologia Electronica, 4(1), 1-9. [ Links ]
Heredia-Arroyo, T., Wei, W. & Hu, B. (2010). Oil accumulation via heterotrophic/mixotrophic Chlorella protothecoides. Appl. Biochem. Biotechnol., 162(7), 1978-1995. [ Links ]
Heredia-Arroyo, T., Wei, W., Ruan, R. & Hu, B. (2011). Mixotrophic cultivation of Chlorella vulgaris and its potential application for the oil accumulation from non-sugar materials. Biomass Bioeng, 35(5), 2245-2253. [ Links ]
Holm, J. Lomborg, C., Oleskowicz, P. & Ebensen, K. (2008). On-line near infrared monitoring of glycerol-boosted anaerobic digestion processes: Evaluation of process analytical technologies. Biotechnol. Bioeng., 99(2), 302-313. [ Links ]
Hu, Q. (2004). Environmental effects on cell composition. In: Richmond, A. (Ed.), Handbookof microalgal culture. Biotechnology and applied phycology. Oxford: Blackwell Publishing. 83-88. [ Links ]
Hu, H. & Gao, K. (2003). Optimization of growth and fatty acid composition of a unicellular marine picoplankton, Nannochloropsis sp., with enriched carbon sources. Biotechnol. Lett., 25(5), 421-425. [ Links ]
Huang, G., Chen, F., Wei, D., Zhang, X. & Chen, G. (2010). Biodiesel production by microalgal biotechnology. Appl. Energy, 87(1), 38-46. [ Links ]
Huppe, H. & Turpin, D. (1994). Integration of carbon and nitrogen metabolism in plant and algal cells. Annual Rev. Plant Physiol. Plant Mol. Biol., 45: 577-607. [ Links ]
Jeon, Y., Cho, C. & Yun, Y. (2006). Combined effects of light intensity and acetate concentration on the growth of unicellular microalga Haematococcus pluvialis. Enz. Microb. Technol., 39: 490-495. [ Links ]
Johnson, D. & Taconi, K. (2007). The glycerin glut: options for the value-added conversion of crude glycerol resulting from biodiesel production. Environmental Progress, 26(4), 338-348. [ Links ]
Kalia, V. & Purohit, H. (2008). Microbial diversity and genomics in aid of bioenergy. J. Ind. Microbiol. Biotechol., 35(5), 403-419. [ Links ]
Khozin-Goldberg, I. & Cohen, Z. (2006). The effect of phosphate starvation on the lipid and fatty acid composition of the fresh water eustigmatophyte Monodus subterraneus. Phytochem., 67(7), 696-701. [ Links ]
Lee, Y. (2001). Microalgal mass culture systems and methods: Their limitation and potential. J. Appl. Phycol., 13(4), 307-315. [ Links ]
Lee, Y. (2004). Algal nutrition. Heterotrophiccarbon nutrition. In: Richmond, A. (Ed.), Handbookof microalgal culture. Biotechnology and applied phycology. Oxford: Blackwell Publishing. 116-124. [ Links ]
Lee, Y., Ding, S., Hoe, C. & Low, C. (1996). Mixotrophic growth of Chlorella sorokinianain outdoor enclosed photobioreactor. J. Appl. Phycol., 8(2), 163-169. [ Links ]
Lee, Y. & Zhang, D. (1999). Production of astaxanthin by Haematococcus. In: Cohen, Z. (Ed.), Chemicals from microalgae. New York: CRC press, Taylor & Francis Group. 173-190. [ Links ]
Li, X., Xu, H. & Wu, Q. (2007). Large-scale biodiesel production from microalga Chlorella protothecoides through heterotrophic cultivation in bioreactors. Biotechnol. Bioeng., 98(4), 764-771. [ Links ]
Liang, Y., Sarkany, N. & Cui, Y. (2009). Biomass and lipid productivities of Chlorella vulgaris under autotrophic, heterotrophic and mixotrophic growth conditions. Biotechnol. Lett., 31(7), 1043-1049. [ Links ]
Lodish, H., Berk, A., Matsudaira, P., Kaiser, C., Krieger, M., Scott, M., Zipursky, L & Darnell, J. (2005). Biología celular y molecular. Madrid: Panamericana (5th ed). [ Links ]
Loera-Quezada, M. M. & Olguín, E. J. (2010). Las microalgas oleaginosas como fuente de biodiesel: Retos y oportunidades. Rev. Latinoam. Biotecnol. Amb. Algal, 1(1), 91-116. [ Links ]
Lv, J., Cheng, L., Xu, X., Zhang, L. & Chen, H. (2010). Enhanced lipid production of Chlorella vulgaris by adjustment of cultivation conditions. Bioresour. Technol., 101(17), 6797-6804. [ Links ]
Meng, X., Yang, J., Xu, X., Zhang, L., Nie, Q. & Xian, M. (2008). Biodiesel production from oleaginous microorganisms. Renew. Energy, 34(1), 1-5. [ Links ]
Nascimento, I. A., Marques, S. S. I., Cabanelas, I. T. D., Pereira, S. A., Druzian, J. I., de Sousa, C. O., Vich, D. V., de Carvalho, G. C. & Nascimento, M. A. (2013). Screening microalgae strains for biodiesel production: lipid productivity and estimation of fuel quality based on fatty acids profiles as selective criteria. Bio. Energy. Research, 6(1), 1-13. [ Links ]
Pérez-García, O., Escalante, F., de-Bashan, L. & Bashan, Y. (2011). Heterotrophic cultures of microalgae: Metabolism and potential products. Water Research, 45(1), 11-36. [ Links ]
Pinto, A., Guarieiro, L., Rezende, M., Ribeiro, N., Torres, E., Lopes, W., Pereira, P. & de Andrade, J. (2005). Biodiesel: An overview. J. Braz. Chem. Soc., 16(6B), 1313-1330. [ Links ]
Plata, V., Kafarov, V. & Moreno, N. (2010). Optimization of third generation biofuels production: biodiesel from microalgae oil by homogeneous transesterification, Chem. Eng. Trans., 21: 1201-1206. [ Links ]
Pyle, D. (2008). Use of biodiesel-derived crude glycerol for the production of omega-3 polyunsaturated fatty acids by the microalga Schizochytrium limacinum. M. Sc. Thesis, Faculty of Biological Systems Engineering, Virginia Polytechnic Institute and State University, Virginia, 72pp. [ Links ]
Pyle, D., García, R. & Wen, Z. (2008). Producing docosa hexaenoic acid (DHA)-rich algae from biodiesel-derived crude glycerol: Effects of impurities on DHA production and algal biomass composition. J. Agricul. Food Chem., 56(11), 3933-3939. [ Links ]
Quiao, H. & Wang, G. (2009). Effect of carbon source on growth and lipid accumulation in Chlorella sorokiniana GXNN01. Chinese J. Oceanol. Limnol., 27(4), 762-768. [ Links ]
Richmond, A. (1986). Microalgae of economic potential. In: Richmond, A. (Ed.), Handbook of microalgal mass culture. Boca Raton, Florida: CRC Press, Inc. 199-243. [ Links ]
Rodolfi, L., Zittelli, G. C., Bassi, N., Padovani, G., Biondi, N., Bonini, G. & Tredici, M. R., (2009). Microalgae for oil: Strain selection, induction of lipid synthesis andoutdoor mass cultivation in a low-cost photobioreactor. Biotechnol. Bioeng., 102(1), 100-112. [ Links ]
Schenk, P., Thomas, S., Stephens, E., Marx, U., Mussgnug, J., Posten, C., Kruse, O. & Hankamer, B. (2008). Second generation biofuels: High-efficiency microalgae for biodiesel production. Bioenerg. Res., 1:20-43. [ Links ]
Song, D., Fu, J. & Shi, D. (2008). Exploitation of oil-bearing microalgae for biodiesel. Chinese J. Biotechnol., 24(3), 341-348. [ Links ]
Statsoft Inc. (2011). STATISTICA 10. [Computer program]. Available in: <http://www.statsoft.com/> [ Links ]
Suh, I. S. & Lee, C. (2003). Photobioreactor engineering: Design and performance. Biotechnol. Biopro. Eng., 8(6), 313-321. [ Links ]
Syrett, P., Bocks, S. & Merrett, M. (1964). The assimilation of acetate by Chlorella vulgaris. J. Exp. Bot., 15(1), 35-47. [ Links ]
Vicente, G., Martínez, M. & Aracil, J. (2004). Integrated biodiesel production: A comparison of different homogeneous catalysts systems. Bioresour. Technol., 92(3), 297-305. [ Links ]
Wang, H., Fu, R. & Pei, G. (2012).Astudy on lipid production of the mixotrophic microalgae Phaeodactylum tricornutum on various carbon sources. Afr. J. Microbiol. Res., 6(5), 1041-1047. [ Links ]
Williams, P. B. (2007). Biofuel: Microalgae cut the social and ecological costs. Nature, 450(7169), 478-478. [ Links ]
Williams, P. & Laurens, L. (2010). Microalgae as biodiesel & biomass feedstocks: Review & analysis of the biochemistry, energetics & economics. Energy Environ. Sci., 3(5), 554-590. [ Links ]
Zar, J. (1999). Biostatistical analysis. New Jersey: Prentice Hall (4th Ed. [ Links ]).