1. Introduction
The tectonic evolution of NW South America, between the Permian and Late Cretaceous-Paleogene, was related to the complex interaction of South American, Caribbean (Plateau and proto-Caribbean) and Farallones plates. The Permian recorded the Pangaea consolidation and the Allaganian Mountains generation (Condie & Sloan, 1997; Yañez, Ruiz, Patchett, Ortega-Gutierrez, & Gehrels, 1991). During the Triassic, the post-Alleganian event marked the rifting onset between Gondwana-Laurentia. Meanwhile, for the Jurassic-Late Cretaceous intra-continental rift (E.g. Cediel, Shaw, & Caceres, 2003 Pindell & Kennan, 2009) or extensional subduction scenarios (E.g. Aspden et al., 1987; Villagómez et al, 2011; Cochrane et al, 2014) have been set out. Two tectonic models explain the geological evolution in the area after these events. First, the allochthone-related models that suggest a Caribbean plate origin (Plateau) linked to the Pacific Plate geological history (E.g. Pindell et al., 1988; Pindell, 1990; Mann et al., 2002; Pindell & Kennan, 2009; Escalona & Mann; 2011). In such models, the NW South America is considered a passive margin between 120 and 84 Myr; and for the Late Cretaceous suggest an arc-continent collision between the Caribbean plate (Plateau) and South America. This collision must have given rise to oceanic crust subduction (Pacific-originated Plateau) occurring since the Campanian (approximately 72 Myr ago; Cediel et al., 2003). On the other hand, autochtone-related models assume that the Caribbean plate was formed between North America and South America. In autochtonous models, subduction is restricted to the proto-Caribbean opening during the Jurassic-Late Cretaceous, and the continental margin starts to behave actively since the Late Cretaceous (8670 Myr) (E.g. Ball et al, 1969; Aubouin et al, 1982; Sykes et al, 1982; Donnelly, 1985; Meschede & Frisch, 1998; James, 2006).
In both scenarios of allochtonous/autochtonous origin, the southeastern margin of the Caribbean/proto-Caribbean plate is characterized by an intra-oceanic arc system called the "Great Caribbean Arc" (sensu Burke, Fox, & Sengor, 1978; Burke, 1988). At the beginning, it was proposed that crystalline rocks from the Greater Antilles, Ave Ridge, Lesser Antilles and Dutch-Venezuelan Antilles represented a single history of the Caribbean Arc (sensu Burke et al, 1978; Burke, 1988). However, some researchers treat these Great Arc components as separate entities, given that they appear to have different tectonic histories and have started as individual arc systems at different times during the Cretaceous through Paleogene (E.g Wright & Wyld 2011; Xie & Mann, 2014).
Finally, and also valid for both, the autochtonous and the allochtonous models during the Cenozoic the region is characterized by accretionary prisms occurence, which NE oriented faults activation and small oceanic crust sections attached to the continental margin of Ecuador, Colombia and Venezuela, suggest a behavior similar to an active margin (E.g. Santamaría & Schubert, 1974; Duque-Caro, 1984; Taboada et al, 2000; Vallejo et al, 2006; Cerón, Kellogg, & Ojeda, 2007; Mantilla-Pimiento, 2007; Toro, Gómez, & Moreno, 2014).
At the north of Colombia (Figure 1), evidence of an active continental margin during the Late Cretaceous, have been reported in magmatic bodies emplaced at the North of the Cordillera Central (CC) (E.g. Ordóñez & Pimentel, 2001a, b; Restrepo-Moreno et al, 2007a, b; Ordoñez, Pimentel, & Laux, 2008; Villagómez et al., 2011), and in the Guajira Peninsula (PG; Cediel et al, 2003; Weber et al, 2009, 2011). However, in other continental margin areas, such as the Lower Magdalena Valley (LMV), there are no reports of igneous activity for the same period and therefore; it has not been considered as part of the geodynamic history. This is a peculiar fact given that for many authors (i.e. Duque-Caro, 1979; Flinch, 2003; López & Barrero, 2003; Cerón et al., 2007) this area is located at the transpressional limit which connected the terranes of the Great Caribbean Arc, the Caribbean plate front limit and the South America North margin.
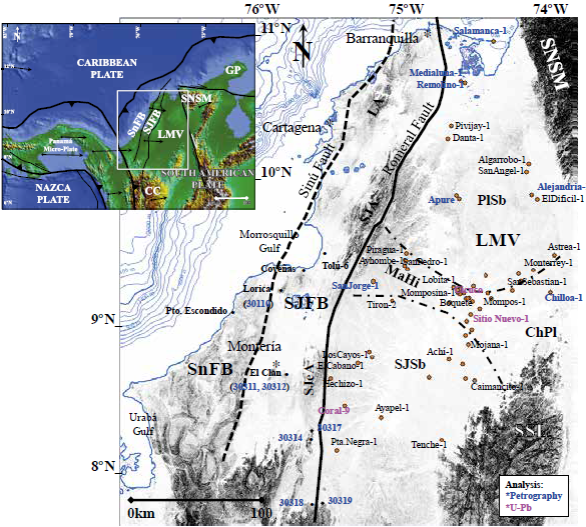
Figure 1 Location map of exploratory wells that drilled basement (Cerón et al., 2007). Also includes the analyses developed in some of the wells and the location of two SJFB outcrops sampled in this study. SnFB: Sinú Fold Belt; SJFB: San Jacinto Fold Belt; SJeA: San Jerónimo Anticline; SJA: San Jacinto Anticline; LA: Luruaco Anticline; LMV: Lower Magdalena Valley; SJsB: San Jorge sub-Basin; PIsB: Plato sub-Basin; MaHi: Magangué High; ChPI: Chimichagua Platform; SNSM: Sierra Nevada de Santa Marta; SLR: San Lucas Range; GP: Guajira Peninsula.
This article integrates information from petrographic analyses of basement samples (crystalline metamorphic and plutonic rocks) from exploratory wells in LMV and outcrops in the San Jacinto Fold Belt (SJFB) area, along with some geochronological dating and geochemical analyses, in addition to a qualitative analysis of magnetic anomalies maps (i.e. Graterol, 2006; Mantilla-Pimiento, 2007). The integration of this information allowed the correlation of basement lithologies existence with magnetic anomalies, providing the basis for a compositional distribution map of the igneous-metamorphic basement and to propose the existence of a magmatic arc in the continental margin at the North of Colombia, specifically in the LMV.
Geological framework
The study area comprises SJFB, part of the Sinú Fold Belt (SnFB), and LMV (Figure 1). The SJFB is between LMV and SnFB, and is separated from them by the Romeral Fault (Northern prolongation of the Romeral Fault System; Grosse, 1926 in: Kammer, 1993; Duque-Caro, 1980; Camargo, 1995; Reyes & Camargo, 1995; Reyes & Clavijo, 1996 in: Ujueta-Lozano, 2007) and the Sinú Fault (Duque-Caro, 1979; Camargo, 1995; Reyes & Camargo, 1995; Reyes & Clavijo, 1996 in: Ujueta-Lozano, 2007), respectively. It encompasses the San Jacinto (SJA) and San Jerónimo (SJeA) anticlines, and it is approximately 360 km long and 60 km wide, with a N20°E trend at the surface (Figure 1; Duque-Caro, 1984). The SnFB is part of the Sinú Accretionary Wedge, which extends to the Colombian offshore, and to the east, it is separated from SJFB by the Sinú Fault (Figure 1). The SnFB is over 500 km long and about 125 km wide. Finally, LMV is delimited to the west by the Romeral Fault and to the east by the Bucaramanga-Santa Marta fault. It is sub-divided in the Plato sub-basin (PlsB), to the North, and the San Jorge sub-basin (SJsB), to the South, both separated by the Magangué High (MaHi), which, according to Barrero, Pardo, Vargas, & Martínez, (2007), controlled the sedimentation in the two depocenters since the Eocene to Late Miocene.
The LMV basement has been interpreted as a continental crust integrated by intrusive and extrusive igneous rocks, and metamorphic rocks (INGEOMINAS, 1997; ICP, 2000; Cerón et al, 2007). The oceanic-affinity basement is exposed in isolated blocks in SJFB (Dueñas & Duque-Caro, 1981; Duque-Caro, 1984; Guzman, 2007: Cerón et al, 2007; Lara et al, 2013). The tectonic limit or suture between the continental crust, to the East, and the considered allochthonous, accretionated terrane of SJFB has been established as the Romeral Fault System (Laverde, 2000). Further West, in the offshore Colombian basin, the seismic refraction and gravity data have identified an anomalously thick oceanic basement with thicknesses of 8 to >20 km (Donnelly, 1994), which consists on a Cretaceous complex of basalt and diabase flows interbedded with sedimentary rocks (Driscoll & Diebold, 1999).
2. Methodology
This study incorporated the petrographic information of 60 exploratory wells (Cerón et al, 2007) and was updated with the inclusion of 14 new petrographic analyses from nine wells (Table 1, Figure 1). Additionally, field work was carried out in the localities of Puerto Escondido, Lorica, Montería and San Jerónimo to the south of SJFB, in order to take samples and verify the nature of outcropping crystalline rocks, through their petrographic characterization (Table 1). The wells and outcrops samples were classified using a 250-point count, in QAPF diagrams (Streckeisen 1976, 1979). The qualitative analysis of magnetic anomalies in the Colombian Caribbean margin delineated the main anomalies using magnetic intensity maps reduced-to-pole (IMTRP. Graterol, 2006. Figure 2a, and Mantilla-Pimiento, 2007. Figure 2B). The latter allowed the expansion of anomalies coverage towards the Chimichagua Platform (ChPl) area.
Table 1 Thin sections analyzed in this study (Figure 1), location, sample type and petrographic description (NA: Not Available; *: petrographic description taken from Cerón ef al., 2007).
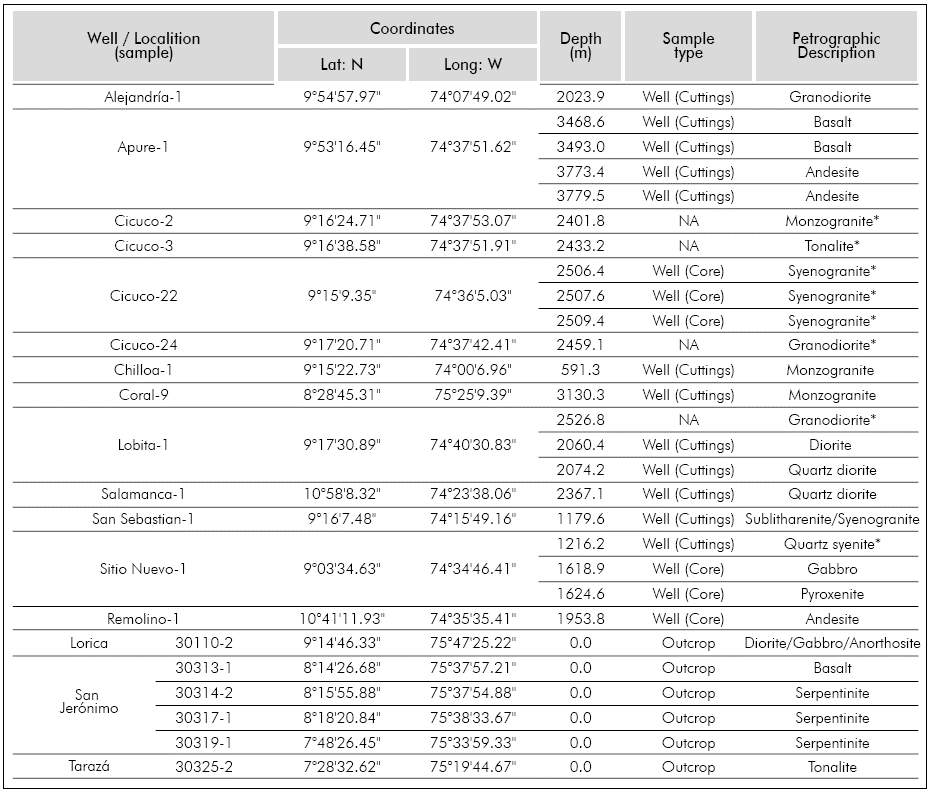
Major, minor and trace elements (MMT) geochemical characterization was performed in three well core samples (Cicuco-22, Remolino-1 and Sitio Nuevo-1) and in three outcrop samples (Table 1, Figure 1). ICP-OES (inductively coupled plasma optical emission spectrometry, equipment type: Perkin Elmer 2100 DV) and ICP-MS (inductively coupled plasma mass spectrometry, equipment type: Perkin Elmer ELAN 6000) were used as analytical techniques. In addition, U-Pb geochronological dating using the LA-ICP-MS technique was performed in zircons from four (4) LMV core samples from wells (Coral-9, Cicuco-22, Sitio Nuevo-1 wells) and one in SJeA (30325; Table IS). Samples from localities 30110, 30311, 30313, and 30314 of the SjeA were also included in the geochronological analyses, nevertheless zircons were absent and no dating was obtained. The details related to the zircon separation process and the corresponding isotopic analysis was summarized in Chang, Vervoort, McClelland, & Knaack, (2006). Finally, petrographic, geochemical and geochronological information was integrated to the qualitative interpretation of magnetic anomalies maps.
3. Results
Distribution of basement magnetic anomalies
Our qualitative interpretation of the magnetic anomalies reduced-to-pole (IMTRP) from Graterol (2006; Figure 2a) and Mantilla-Pimiento (2007; Figure 2b), shows a differentiation in the intensity of magnetic anomalies along the Romeral Fault. This limit separates a scenario of generally-high magnetic anomalies towards the SJFB and SnFB, contrasting with a wide spectrum in intensity of magnetic anomalies towards the LMV (Figure 2a). In SJFB and SnFB, the magnetometric values are estimated between 33950 and 34320 nT (according to Graterol's scale, 2006) including high magnetic anomalies (34020-34320 nT) distributed widely along the SnFB shoreline (i.e. Cartagena and Puerto Escondido), or with a larger extension but following the direction of the Romeral Fault (at -30 km to the W) in SJFB (i.e. Montería, Lorica, Coveñas and Tolu). The main SJFB magnetic anomaly is identified on the Romeral Fault at SJeA, where it has a clear elongated shape (-150 km long and < 25km wide), in a N10°E trend over the area connected to the SJsB (Figure 2a). Towards the LMV, the complete spectrum of magnetic anomalies is included, from low to high levels, with magnetic anomalies easily delineated and interpreted as medium- and high-intensity (33960 and 34320 nT), opposed to an environment of predominantly low magnetic anomalies (33345-33960 nT; Figure 2a). The main LMV magnetic anomaly is located in the MaHi, where the San Jorge-1, San Pedro-1 and Cicucos wells are located. The anomaly has a N60°W trend, -70 km long and 40-25 km wide, and separates the basin into two depocenters. To the NE, PlsB includes small anomalies of high magnetic intensity along the Romeral Fault (<25 km to the SE; Apure-1, Pivijay-1, Remolino-1 and Salamanca-1 wells) (Figure 2a); and to the SW, SJsB has some larger anomalies of high intensity facing SJeA, where Coral-9 and Ayapel-1 wells are located (Figure 2a).
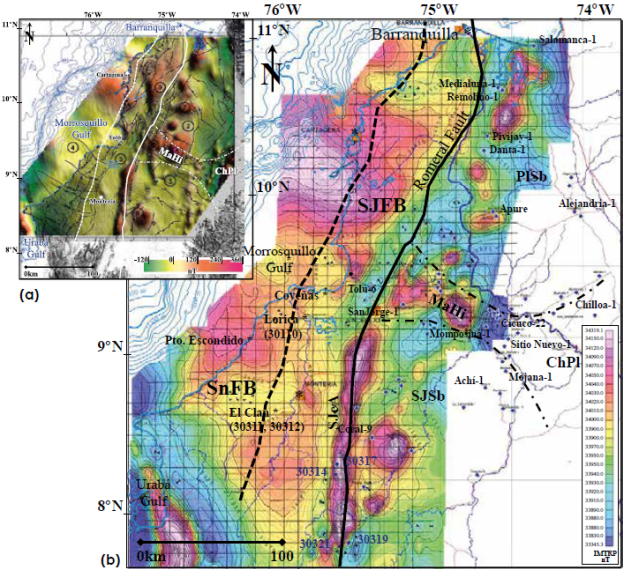
Figure 2 IMTRP magnetic anomalies map (magnetic reduced-to-pole) in the versions of a) Graterol (2006)/ and b) Mantilla-Pimiento (2007); with the location of wells and analyzed outcrops (Table 1). SnFB: Sinú Fold Belt; SJFB: San Jacinto Fold Belt; SJeA: San Jerónimo Anticline; SJA: San Jacinto Anticline; LA: Luruaco Anticline; LMV: Lower Magdalena Valley; SJsB: San Jorge sub-Basin; PIsB: Plato sub-Basin; MaHi: Magangué High; ChPl: Chimichagua Platform; SNSM: Sierra Nevada de Santa Marta; SLR: San Lucas Range; GP: Guajira Peninsula.
Petrography
The petrographic analysis of some LMV well samples allowed the identification of igneous rocks (plutonic and volcanic) in nine of the analyzed wells (Table 1, Figure 1). The felsic plutonic rocks were classified as granodiorite in PlsB (Alejandría-1 well) and monzogranite in the ChPl and SJsB (Chilloa-1 and Coral-9 wells, respectively) (Figures 2 and 3a). The monzogranite from the Chilloa-1 well has an inequigranular allotriomorphic texture with local perthitic textures (Figure 4a). The mafic plutonic rocks were classified as quartz diorites-diorites in PlsB (Medialuna-1 and Salamanca-1 wells) and as gabbro-pyroxenite in MaHi (Sitio Nuevo-1 well) (Figures 2, 4b and 4c). On the other hand, the volcanic rocks identified in LMV were classified as andesites (Remolino-1 and Apure-2 wells) (Figure 3b) and calc-alkaline basalts (Apure-1 well) in the PlsB (Figure 2). The andesites show a glomeroporfidic texture (Figure 4d); while the basalts have an amigdaloid texture formed by vacuoles filled with chlorite (towards the borders).
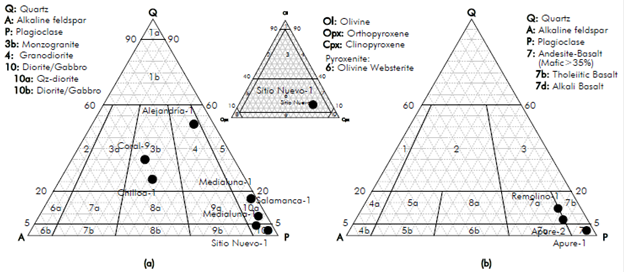
Figure 3 a) Streckeisen (1 976) classification diagrams for plutonio felsic, mafic and ultra mafic rocks from wells: Alejandría-1, Coral-9 and Chilloa-1, Salamanca -1, Medialuna -1, (Streckeisen/ 1979) and Sitio Nuevo-1. b) Streckeisen (1979) classification diagram of volcanic rocks from the Remolino-1, Apure-1 and Apure-2 wells.
An analysis was also performed on outcropping serpentinized samples in SJeA. The first ones correspond to Planeta Rica Peridotites (stations 30314 and 30317; Figure 2), which have a pseudomorphic texture (olivine and pyroxene-like texture) with minerals such as lizardite (91.3%), chrysolite (1.2%) and antigorite (1.1%) (Figure 4e). Estimated age from those peridotites is Upper Cretaceous according to Dueñas & Duque-Caro (1981). A second sample cluster correspond to the Uré basic and ultrabasic Complex (stations 30318 and 30319; Figure 2), which present a pseudomorphic texture and relicts (olivine crystals) with minerals such as lizardite (78.5%), antigorite (5.6%) and chrysolite (5.2%) (Figure 4f). Estimated age of these basic and ultrabasic complex is Lower Cretaceous according Zapata & Cossio (2001). Finally, in the Tarazá area (station 30325), the composition of tonalite from the Puquí Complex (Lower Triassic; Carmona & Pimentel, 2002) was classified as follows: plagioclase (41-36%), quartz (40-35%), biotite (10-6%), moscovite (6-4%), potassic feldspar (4.8-4.1%) and opaque minerals (4.4%) filling interstitial spaces, with inequigranular hipiodiomorphic texture, and myrmekite-like local texture.
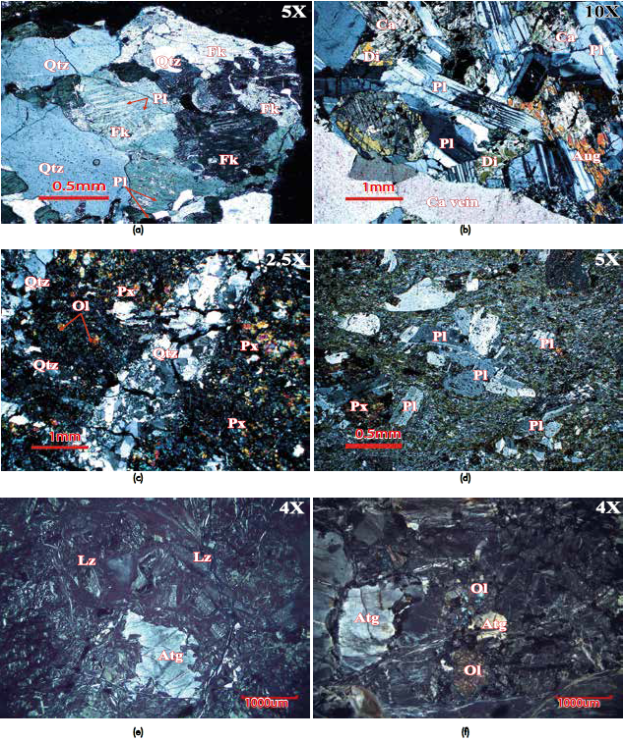
Figure 4 a) Monzogranite fragments in the Chilloa-1 well cuttings, with inequigranular allotriomorphic texture of anhedral quartz crystals (Qtz), potassic feldspar (FK) and plagioclase (PI); showing a local perthitic texture of PI lamellas within the FK and incipient development of seriate as a product of hydrothermal alteration, b) Gabbro from the Sitio Nuevo-1 well with plagioclase crystals (PI), diopside (Di) and augite (Aug), altered to calcite (Ca). c) Pyroxenite from the Sitio Nuevo-1 well with pyroxenes (Px) and olivine (Ol) and epigenetic quartz veinlets (Qtz). d) Andesite from the Remolino-1 well with glomeroporfidic texture with plagioclase phenocrysts (PI) with a Pl-Qtz-Px matrix, e) Serpentíníte with pseudomorphic texture with slight preservation of the texture features of Ol and Px which have been strongly altered to lizardite (Lz) and antigorite (Atg) from the original Planeta Rica Peridotite. f) Serpentíníte with pseudomorphic texture still preserving relict olivine crystals (Ol) altered to antigorite (Atg), coming from the original peridotite from the basic and ultrabasic Uré Complex.
Geochemistry
Annex 1 contains detailed results of chemical composition of core samples from the LMV, on the basis of different relationships between the main oxides, and between these and trace elements and Rare Earths study. The samples from Sitio Nuevo-1, Remolino-1 and Cicuco-22 wells are projected toward the field of sub-alkaline magmatic rocks (Figure 5a), and are geochemically classified as follows: Basaltic-Andesite (Sitio Nuevo-1 well) and Rhyolite (Cicuco-22 well) in MaHi, and Dacites in PlsB (Remolino-1 well) (Figure 5b). The results allow establishing a link between the igneous lithologies analyzed from the Remolino-1, Cicuco-22 and Sitio Nuevo-1 wells, and subduction settings (Figures 5c-f). The BaLa ratio in these igneous rocks (Remolino-1=110,21; Cicuco-22=80,90 and Sitio Nuevo-1=47,84), allows to suggest more specifically that these lithologies were formed in an arc settings (threshold values >20). The La/Yb vs. Sc/Ni ratios allow to propose that the magmatism generated by the PlsB igneous rocks (Remolino-1 well), took place in a thinned continental crust, while those found in MaHi (Cicuco-22 well), seem to be more related to a thicker continental crust (Andes type).
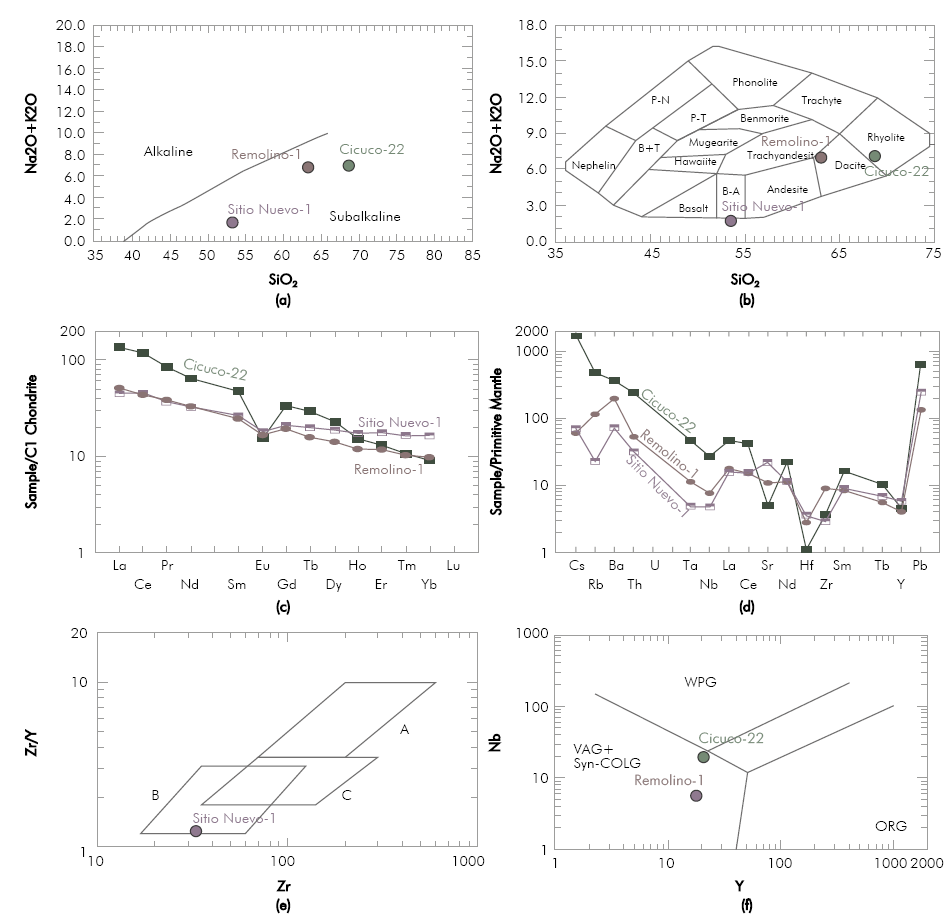
Figure 5 Geochemical diagrams for the classification of samples from the Sitio Nuevo-1, Cicuco-22 and Remolino-1 wells, a) Diagram for discrimination of magma series according to Irvine & Baragar (1971); b) TAS classification (Cox ef al., 1979); c) Rare Earths Pattern/ normalized to Chondrite CI; d) Trace elements/ normalized to the Primitive Mantle; e) Diagram for discrimination of the tectonic setting by Pearce & Norry (1 979). Extrusive analogous of the plutonic rock herein analyzed, f) Nb vs. Y diagram for the discrimination of the tectonic setting for magma emplacement (Pearce ef al., 1 984).
Regarding the samples collected in the SJeA, it is evident that the classification of igneous materials, according to their chemical composition, is clearly inconsistent with the rock lithology (Annex 2). Such is the case of the basalt (station 30313) and serpentinite (station 30314) from the Planeta Rica area, which reach SiO2 values of 71.60% and 63.99% in weight, which would project these rocks towards the field of acidic rocks. Even though contamination is not discarded, their high SiO2 content may also suggest the presence of quartz veinlets in the sample, or possible alterations caused by silicification. More specifically, the serpentinite sample (station 30314) from the Planeta Rica area shows a high MgO content (Annex 2), which is characteristic of peridotitic materials (ultramafic, primitive rocks). This rock shows serpentinization of its mafic minerals and might be related to materials characteristic of the lithospheric mantle upperparts (due to the presence of plagioclase), which may have experienced hydration processes (serpentinization) as a response to the fluids seepage leakage derived from dehydration (fluid release) of the subducted materials (located under the peridotitic materials).
On the other hand, the basalt analysis from Planeta Rica area (station 30313) and tonalite from Tarazá area (station 30325), allows to conclude that these materials are related to supra-subduction magmatic arcs (Annex 2), which have a different maturity degree, being basalt (station 30313) originated from a slightly more primitive magmatic arc, as compared to tonalite (station 30325).
Geochronology
In this study, four geochronological datings were performed (Table 2). In the MaHi, a gabbro from Sitio Nuevo-1 well (core) was dated; its analytical data and the U-Pb isotopic relationships determined in different growth areas of the zircons analyzed with the LA-ICP-MS technique (34 analyses performed), indicate the presence of a single group showing an age of 300 ±1.3 Myr (Figure 6a; Annex 3). Also in the MaHi, a syenogranite from the Cicuco-22 well (core) was dated; its analytical data and the U-Pb isotopic relationships (37 analyses performed) indicate the presence of six age groups, even though the largest group has an age range related to the syenogranite crystallization, of 84.6 ± 0.6 Myr (68%, 25 data points) (Figure 6b; Annex 3). In SJsB, monzogranite from the Coral-9 well (cuttings) was dated; its analytical data and the U-Pb isotopic relationships (38 analyses performed) indicate the presence of eight age groups: the most recent group shows an age range for monzogranite of 74.5 ± 1.9 Myr (21%), and the second group shows an age of 232 ±3.2 Myr (55%) (Figure 6c; Annex 3). This second age group is considered as inherited and derived from zircons coming from Triassic rocks. To the South, the tonalite from the Puqui Complex in the Cordillera Central foothills (station 30325) was dated; the U-Pb isotopic relationships (65 analyses performed) indicate the presence of at least six age groups: the most recent group (52%, 34 data points) shows an age range for tonalite of 231.8 ± 4 Myr, while the second group (42%, 27 data points) shows an age of 850 My (Figure 6d; Annex 3).
Table 2 Distribution of wells and outcrops by: structural domain, location, lithology, estimated intensity of magnetic anomaly (IMTRP by Graterol, 2006) and U-Pb age. (ID: Indeterminate; *: Petrographic description taken from Cerón ef a/., 2007; f: U/Pb dating taken from Montes ef a/., 2010; #: Ar-Ar dating taken from Lara ef a/., 2013).
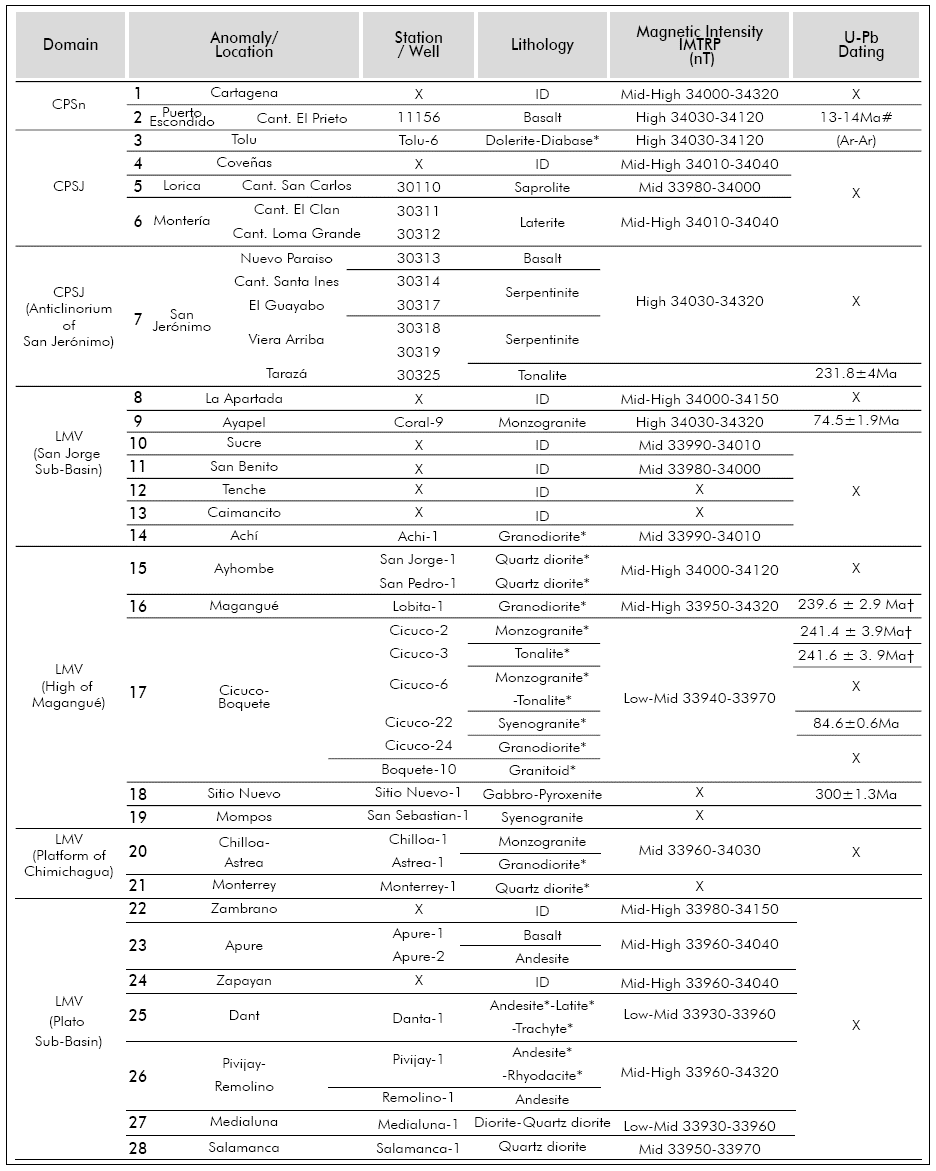
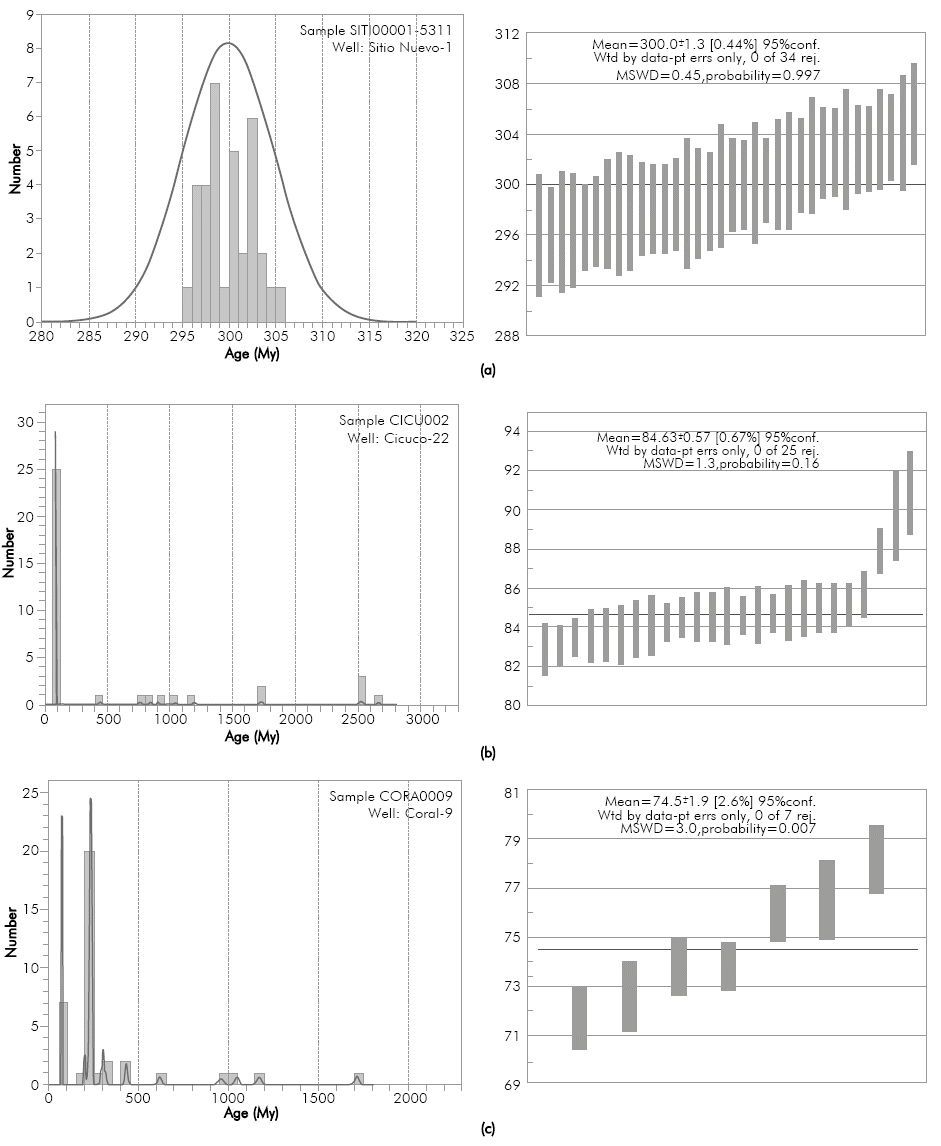
Figure 6 Age probability distribution for the zircons sampled in the Sitio Nuevo-1 a), Cicuco-22 b), Coral-9 c) wells and station 30325. At the right of each histogram, the possible ages of basements and a summary of every age of the zircons calculated using the 206Pb/238U ratio are illustrated, including the errors such as 2s uncertainties (see discussion in text).
4. Discussion
Correlation between Magnetic anomalies and basement lithologies
The combination of lithological composition and qualitative interpretation of magnetic anomalies (IMTRP), allowed generating a basement lithological occurrences map for the South Caribbean Colombian margin (Figure 7, Table 2). On this map, two high-intensity magnetic anomalies were identified in SnFB: (1) the undetermined anomaly to the North of Cartagena, which extends towards SJFB, and, (2) the anomaly to the South, corresponding to basalts emplaced in Middle Miocene rocks from the Puerto Escondido area (station 11156, Table 2, Figure 2a) (Lara et al, 2013) (Figure 7). The prolongation of these anomalies towards the offshore is limited by the IMTRP maps extension.
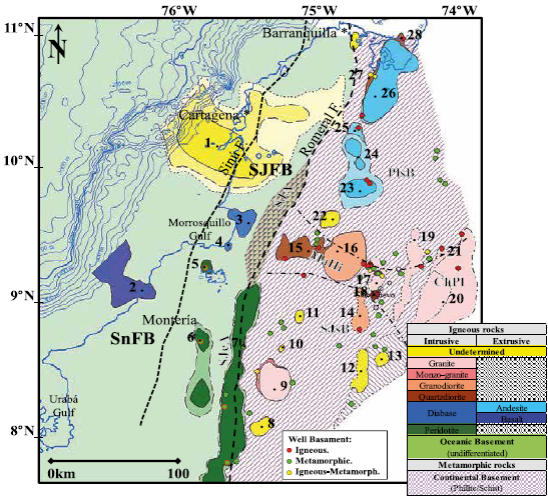
Figure 7 Map of basement lithological occurrences defined from the correlation of well composition data, outcrops and magnetic anomalies (listed in Table 2). SnFB: Sinú Fold Belt; SJFB: San Jacinto Fold Belt; SJeA: San Jerónimo Anticline; SJA: San Jacinto Anticline; SJsB: San Jorge sub-Basin; PlsB: Plato sub-Basin; MaHi: Magangué High; ChPl: Chimichagua Platform.
In the SJFB, five high intensity magnetic anomalies were identified, which mainly correspond to igneous bodies of mafic and ultramafic composition (Table 2). They are identified from North to South as follows: (3) high intensity anomaly from the Tolu-6 well diabase, which can be extended to the SE toward the (4) Coveñas area (Figure 7). To the SSW, the medium to high intensity anomalies correspond to the saprolitized peridotites from (5) Lorica (San Carlos quarry) and the lateritized peridotites from (6) Montería (El Clan and Loma Grande quarries). In both areas, peridotites outcrop in imbricate thrusts underlying the Upper Cretaceous Cansona Formation (Annex 4). To the East, the high-intensity anomaly of (7) San Jerónimo, corresponds to serpentinites (metamorphized peridotites), identified in the El Guayabo, Santa Inés Quarry and Viera Arriba area (Table 2). In addition, basalts were identified in Late Cretaceous rocks (Cansona Formation) and Paleocene (San Cayetano Formation), in the Nuevo Paraíso and Quebrada Nueva areas (station 30321; Table 1) (Figures 2 and 7).
To the SW of the LMV, 14 anomalies (medium to high intensity) are identified and distributed as follows: seven anomalies in SJsB, five anomalies in MaHi and two anomalies in ChPl, which correspond to felsic and intermediate intrusive rocks (syenogranite, monzogranite, granodiorite and quartz diorite), in addition to mafic intrusive rocks (gabbro-pyroxenite; Sitio Nuevo-1 well) (Table 2; Figure 7). On the contrary, to the NE of LMV, in PlsB, seven magnetic anomalies are identified (medium to high intensity) and correlated to extrusive felsic to mafic rocks (trachyte, andesite, rhyodacite and basalt) and some anomalies corresponding to intermediate-composition intrusive rocks (diorite-quartz diorite; Medialuna-1 and Salamanca-1 wells) (Table 2; Figure 7). On the map, the Romeral Fault seems to define the surface contact between the oceanic-like mafic rocks, outcroping to the NW, and the intrusive and extrusive, felsic to intermediate rocks, intruded in a continental basement to the SE (Duque-Caro, 1979; Flinch, 2003; Cerón et al, 2007; Mantilla-Pimiento, 2007) (Figure 7).
Tectonic evolution
According to the U-Pb datings (Table 2), there is a Permo-Triassic (300 - 235 Myr) magmatic pulse, recorded in granitoids (Lobita-1: 239.6 ± 2.9 Myr, Cicuco-2 and -3: 241.4 ± 3.9 Myr; Montes et al. 2010), and gabbros (Sitio Nuevo-1: 300 ± 1.3 Myr, Figure 6a) identified at the MaHi. The gabbros presence in a continental crust stands out since they are of metaluminic nature (type-I magma), which corresponds to a supra-subduction setting (Figure 5e). As emplacement mechanism it is proposed the occurrence of cortical fissures. Other locations where magmatic crystallization has been recorded from the Late Permian to the Early Triassic are: the SNSM (288.1 ± 4.5 Myr and 276.5 ± 5.1 Myr; Cardona et al, 2010), the North ofthe CC (248 ± 17 Myr; Ordoñez and Pimentel, 2001a, b), and the Puquí Complex (Tarazá, station 30325), which tonalite sample exhibits an age of 231.8 ± 4 Myr (Figure 6d; Annex 3). This first tectono-thermal event occur in the frame of the Pangaeas collision and Allaganian Mountains formation during the Permian (Woodward, 1957 in: Secor, Snoke, & Dallmeter, 1986); as well as the post-Alleganian event marking the rifting onset between Gondwana-Laurentia during the Early-Mid Triassic in the LMV basement.
A second, Late Cretaceous, magmatic pulse, is recorded in different LMV locations. In the MaHi, a syenogranite of peraluminic nature (metaluminic magma fractionation or cortical contamination), and characteristic of a thick continental crust, generated in a subduction setting (Figure 5f) yields an age of 84.6 ± 0.6 Myr (Cicuco-22 well; Figure 6b). In the SJsB, a monzogranite yields an age of 74.5 ± 1.9 Myr (Coral-9 well; Figure 6c), in addition to an inherited age from the first Triassic magmatic pulse (232 ± 3.2 Myr; Figure 6c). This evidence suggests the existence of a magmatic arc of Late Cretaceous age in the LMV basement, defined in this paper as Magangué Magmatic Arc. Its existence is based upon the igneous bodies occurrence along the Romeral Fault (Figure 7), among which Permian mafic (Sitio Nuevo-1) and Triassic felsic sources (Montes et al. 2010) are identified in the MaHi; as well as Late Cretaceous sources (Cicuco-22, Coral-9 wells) in the MaHi and SJsB (Table 2), in addition to the presence of intermediate intrusive-extrusive bodies in the PlsB (Table 2).
The ages (84-72 Myr) and geochemical characteristics of the magmatic bodies in the LMV are similar to those reported in a group of outcropping igneous rocks in the Cordillera Central (CC), as those from the Antioquia Batholith (E.g. Restrepo-Moreno et al., 2007a, b; Ibañez, Tassinari, & Jaramillo, 2007; Ibañez, Jaramillo, & Valencia, 2008; Ibañez, Vinasco, Cardona, Valencia, & Weber, 2010); as well as to other small intrusive bodies, including the Sonsón and Segovia batholiths, distributed in the North segment of CC (Ordóñez & Pimentel, 2001a, b; Restrepo-Moreno, Foster, & Kamenov, 2007a), b; Ordóñez et al, 2008; Villagómez et al., 2011). Therefore, this study proposes the continental basement continuity from the Tahami Terrane (sensu Restrepo & Toussant, 1988) up to the LMV (Figure 8), with the existence of at least two important magmatic arcs, developed within the same context of supra-subduction and as a response to the interaction between the Farallones or the ancient proto-Caribbean plates and the South American plate (E.g. Aspden et al, 1987; Ordoñez et al, 2008; Pindell & Kennan, 2009; Restrepo-Moreno et al, 2009; Villagómez et al., 2011).
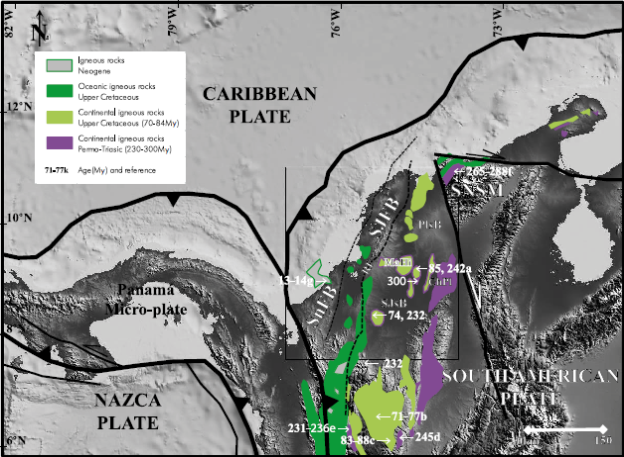
Figure 8 Distribution of the Magangue Magnetic Arc in the LMV to the North and continuity of intrusive bodies of the two magmatic pulses identified in the LMV (Permo-Triassic and Late Cretaceous) (modified from Ayala et al., 2012). Ages from Montes et al. (2010); Restrepo et al. (2007); Ibañez et al. (2007); Ibañez et al. (2008); Rodriguez-Jimenez (2010); Cardona et al., (2010); and Lara et al. (2013). SnFB: Sinú Fold Belt; SJFB: San Jacinto Fold Belt; PlsB: Plato sub-Basin; MaHi: Magangué High; Ch: Chimichagua Platform; SJsB: San Jorge sub-Basin; SNSM: Sierra Nevada de Santa Marta.
Even though the Magangué Magmatic Arc reflects a Late Cretaceous active margin, it does not necessarily imply its juxtaposition with an oceanic Magmatic Arc, represented by the Planeta Rica basalts (station 30313). At the moment of its formation, the oceanic basement must have been in a different location and subsequently must have been accretioned to the continental plate, during the Late Cretaceous/Paleogene as evidenced by the saprolitized and lateritized peridotite folded and thrusted below the Cansona Formation (Annex 4).
Additionally, establishing the petrogenetic and chronological relationship of these igneous bodies, of mafic and ultramafic composition, as reported in the Planeta Rica, Montería and Lorica areas, with other allochtonous fragments from the Caribbean Plate attached to the northern margin of South America is a difficult task. To the north of the LMV, in the SNSM, Cardona et al. (2010) have reported a magmatic event, associated to subduction (90 - 65 Myr), which generated the crystallization of an orthogneiss and intra-plate volcanic-arc granitoids formed by melting of metabasalt sources (50 - 68 Myr). In the Guajira Peninsula, Weber et al. (2009) associate the Cabo de la Vela Mafic Complex (and possibly the Etpana Formation) with back-arc settings, occurring over on oceanic crust over the Benioff Zone, which then experienced a transition toward an intraoceanic island arc. Furthermore, to the East of Colombia, in the Paraguaná Peninsula, Los Monjes Island, Curaçao Island, Gran Roque Island, mafic composition lithologies are displayed (Santamaría & Schubert, 1974; Toro et al, 2014). These rocks represent a tholeitic series, characteristic of oceanic ridges and, by extension, of sea floor in general. Further to the East, in the Aves Ridge and Lesser Antilles areas, there is basaltic and doleritic lithologies to which an intraoceanic-arc origin was attributed (Neill, Kerr, Hastie, Stanek, & Millar, 2011; Santamaría & Schubert, 1974; Bouysse et al, 1990). However, the uncertainty regarding the formation age of serpentinites and basalts from the Planeta Rica area, as well as genetic processes difference, make it impossible to establish a link between these rocks and what are considered relicts of the Great Caribbean Arc.
5. Conclusions
The described and delimited lithologies from the LMV basement, as well as new well data ages reported here, support a basement correlation to the lithologies and ages identified in the CC, being the LMV basement the northern extension of the Tahami Terrane from the CC.
The Magangué Magmatic Arc of the LMV has a Late Cretaceous age (84 - 70 Myr) and consist of felsic to intermediate igneous bodies (Coral-9 and Cicuco-22 wells), which intrude an older tectono-thermal event of Permo-Triassic age (300-235 Myr), also identified in the MaHi (Sitio Nuevo-1 well and wells dated by Montes et al., 2010) and in SJsB (inherited zircons in the Coral-9 well).
The existence of the Magangué Magmatic Arc and its close tectono-temporal relationship with an important group of igneous rocks outcropping in CC (i.e. Antioquia, Sonsón, Segovia, and other batholiths) supports the establishment of an Active Continental Margin for the Late Cretaceous, implying the onset of subduction of the Farallon or proto-Caribbean plates underlying the North of the South American Plate, with the intrusion of felsic to intermediate magmas (some peraluminic) in the LMV continental crust.
In the aforementioned tectonic setting, the differences between the Magangué Magmatic Arc and the Antioquia Batholith would be related to the Wadati-Benioffzone depth, and its corresponding implications: a progressive decrease in volatiles contribution toward the mantle wedge (as the subducted plate became deeper), and the subsequent reduction of the mantle wedge partial fusion percentage, among other aspects. The knowledge of the relationship between the two aforementioned magmatic arcs has an evident implication in the mineral deposits exploration and the evolution of Colombian sedimentary basins.