1. Introduction
The vinasse is a liquid waste generated by ethanol distilleries at a ratio of 8 to 15 liters of vinasse per liter of alcohol produced. This effluent has an acid pH (<4) and a high pollution potential due to its high chemical oxygen demand (COD) between 30,000-150,000 ppm (Nava, Recéndiz, Acosta, & González, 2008) and biochemical oxygen demand (BOD) between 40,000 and 50,000 ppm (Khandegar & Saroh, 2014). The vinasse is considered a recalcitrant effluent with a variable and complex composition; one of its constituents are the long polymeric molecules resulting of the exposure at high temperatures of residual sugars known as melanoidins. The melanoidins are responsible for the dark brown color of the vinasse and when they are poured into water bodies, they reduce the solar light penetration, decreasing the photosynthetic activity and the amount of dissolved oxygen (Chandra, Bharagava, & Rai, 2008).
Actually, the most common industrial treatment for this waste is the concentration (up to 55% in solids) using multiple effects evaporators and the subsequent fertigation into the sugar cane crops at dosages varying between 5 to 200 m3 ha1 per application (Chauhan & Dikshit, 2012)(Robles-González, Galindez-Mayer, Rinderknecht-Seijas, & Poggi-Varaldo, 2012)(Devesa-Rey et al., 2011). Nonetheless, this solutions do not solve the environmental problem of vinasses since their extensive use in soils causes a reduction of land's alkalinity and inhibits seed germination (Chandra et al., 2008). Recent investigations present different technologies for the vinasses treatment, including physicochemical methods (Liakos & Lazaridis, 2014) (Liang, Wang, Zhou, & Liu, 2009)(Arimi, Zhang, & GeiBen, 2015) that are associated with the use of high concentrations of reagents and large sludge production. Also, microbiological treatments have been tested (Wagh, 2015) with low efficiency and requiring long residence time (Cañizares et al., 2009). Up to date, there is no treatment that achieves full degradation of pollutants in this type of wastewater or allow the recirculation of treated water in the ethanol-sugar agro-industrial process.
Anodic oxidation or electro-oxidation is one of the most popular electrochemical procedures for elimination of dissolved organic pollutants and consists in the oxidation of organic matter in an electrolytic cell. When using boron doped diamond anodes, properties of this material enable the oxidation of organic compounds mediated by hydroxyl radicals. The mechanism consists in the H20 oxidation at the anode surface to produce a slightly adsorbed hydroxyl radical on the electrode (Eel), that reacts with the organic molecules (R) that reach the vicinity of the anode producing C02 and H20 as shown in Ec.2. (Comminellis & Guohua, 2010)
Since the invention of the BDD material, the anodic oxidation was born as a promising technique, because of its capacity of degrading pollutants in a non-selective manner to non-dangerous compounds. Despite being a technology with less than 25 years of existence, the records of patents about electrochemical technologies in wastewater treatment with BDD electrodes evidence an interesting dynamic of innovation with a growing interest by researchers in the industry and the academy. (Alvarez-Pugliese, Marriaga-Cabrales, & Machuca-Martínez, 2014)
In fact, investigations on the electro-oxidation of ethanol distillery wastewater at laboratory scale with synthetic wastewater (Cañizares et al., 2009) (Nava et al., 2008) and real wastewater (Alvarez-Pugliese, Moreno-Wiedman, Machuca-Martínez, & Marriaga-Cabrales, 2011) (Batista, Oliveira, Ferreira, Miwa, & Santos, 2011) have been published. In such studies the mineralization of organic compounds higher than 80% were obtained, achieving full mineralization of recalcitrant compounds such as melanoidins. Nonetheless, the investigations were conducted at a reduced scale of treatment and in diluted matrixes, that does not allow to establish specific energy consumption, degradation efficiency and operation time for the treatment of real distillery effluents at large scale.
Therefore, considering the exhibited capacity for the degradation of recalcitrant organic compounds through the electro-oxidation with BDD anodes, this study assesses different operating conditions at pilot scale for the first time for this type of effluent.
2. Experimental development
Vinasse
The raw vinasse was taken from the bottom of the stripping column of a local ethanol distillery, from sugarcane molasses "B" fermentation, and it was kept at 4°C. Commercial biocide BIOBAN was added at a concentration of 0.01% (v/v %) for the conservation of the samples.
Chemical Analysis
Color was measured by the absorbance at 420 nm in a Thermo Fisher Scientific GENESYS 10 Vis spectrophotometer. Additionally, absorbance spectrums between 200 and 800 mm were made in the Spectroquant Pharo 300 MERCK spectrophotometer to assess the qualitative degradation of pollutants. The dissolved organic carbon (DOC) was measured using the Shimadzu TOC-VCPH 5050 analyzer, the UV-Vis spectrums were measured in the SHIMADZU UV Spectrophotometer UV 1800. Measurements of pH and conductivity were made with a pH-meter Accumet Fischer Scientific AB15.
Laboratory scale Tests
Preliminary laboratory scale tests were made to establish the operation conditions in an acrylic cell with a volume of 12.5 cm3 fed by a reservoir tank of 200 cm3. The cell has only one compartment with two plate electrodes in parallel (Figure 1). The anode was a BDD supported on Si from Fraunhofer USA and the cathode was made of stainless steel (SS 304), both electrodes with an active area of 3.14 cm2.
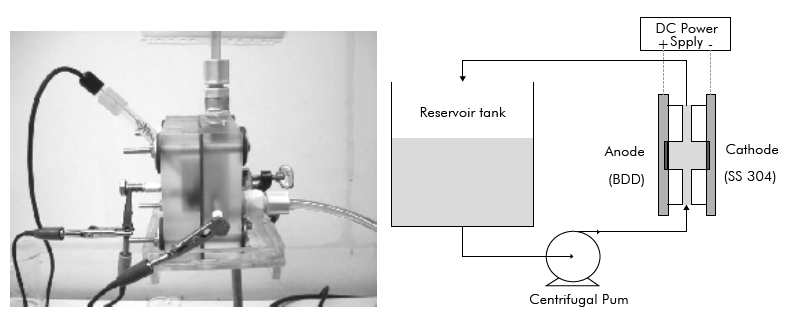
Figure 1 Photograph of the electrochemical cell and experimental setup for the laboratory scale tests.
The preliminary tests were executed at 30±2°C and a volume of 120 cm3 of pretreated vinasse. Additionally, before each test, the anode was polarized at 40 mA cm 2 for 5 minutes with a 0.1 M of H2SO4 solution to clean the electrode surface. The conductivity was adjusted to 12 mS cm-1 with anhydrous Na2SO4.
The experimental conditions at the laboratory scale were evaluated with a 23 factorial design. The initial pH, current density (j) and Reynolds Number (Re) were the studied factors. The two levels of the initial pH were 4 and 10, the acid pH was adjusted with a H2SO4 solution 5% (v/v %); the current density levels were 60 mA cm-2 and 100 mA cm 2; and the Re used were 2,700 and 14,600, that represent volumetric flow rates of 70 and 390 dm3 h1. A specific electric charge (Q) of 7.2 A h dm 3 was passed through the cell.
Pilot scale Tests
The assembly used in the pilot scale tests (Figure 2) is composed of a 50 dm3 vinasse storage tank, 50 dm3 recirculation tank, a variable speed centrifugal pump and a CONDIACELL reactor equipped with DIACHEM® electrodes provided by CONDIAS (Germany). The reactor has a stainless-steel casing and an active anodic and cathodic area of 1,500 cm2, the DIACHEM® anodes are both side coated with a BDD film supported on Nb and the cathodes are made of stainless steel.
For each test, a volume of 25 dm3 of pretreated vinasse was used and the initial conductivity was adjusted to 12 mS cm-1 using anhydrous NajSO,,. Before each test, an anodic polarization was conducted at 40 mA cm 2 for 5 minutes with 0.1 M H2SO4
The experiments were conducted at three different current densities (35 mA cm 2, 60 mA cm 2 and 100 mA cm 2) at a flow rate of 4,500 dm3 h1 that represents a Re of 39,700. The temperature was controlled at 30±2 °C. Two specific electric charges were studied 7.2 A h dm 3 and 25.2 Ah dm3.
Additionally, an essay was performed to identify the effect of electro-generated oxidants that can be produced during the electro-oxidation when using electrolytes containing chloride ion. For this purpose, NaCl was used as an electrolyte adjusting the conductivity to 12 mS cm1 and the test was conducted at a current density of 60 mA cm 2 and a Re of 39,700.
Energy Consumption
The specific energy consumption (W) of the electrolytic system was reported in kilowatts-hour per volume treated (kWh nr3). This consumption was calculated in accordance with the following equation:
Where I is the current (A), U is the average cell potential (V); t is the electrolysis time (h); V is the volume of the solution treated (m3).
3. Results and analysis of results
The electro-oxidation of wastewater is recommended for diluted or pretreated effluents (Comminellis & Guohua, 2010). When applying this technology to effluents with high organic concentrations increases the treatment costs and makes it economically unfeasible. For this reason, the raw vinasse was pretreated by the Electroflox-UV® process (Machuca Martinez, Marriaga Cabrales, & Cardona Palomino, 2011) consisting in an iron electro-dissolution and flocculation process at alkaline pH. This process remove up to 95% of the solids existing in the raw vinasse, and generates a sludge with high concentration of solids and a clarified treated vinasse supernatant.
The vinasse supernatant resulted was kept at 4°C and was used in all the research tests. The Table 1 shows the physicochemical characterization of both wastewater.
Laboratory scale Tests
The results obtained in the preliminary test are shown in Table 2. Additionally, these results were analyzed by a Pareto diagram (Figure 3).
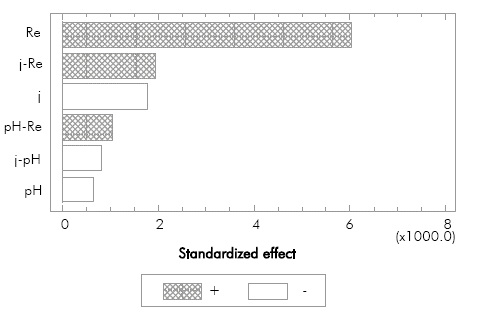
Figure 3 Pareto diagram of standardized effects in the factorial design for the laboratory scale essays.
According to Figure 3, changing the initial pH from 4 to 10 had little influence in the electro-oxidation process, since this variable only affected the color removal in an average of ±1.40%. This result is in concordance with different investigations (Palma-Goyes et al., 2010) (Alvarez-Pugliese etal., 2011). The variation of the Re shows a strong influence in color removal, confirming that under the conditions studied the process is controlled by the mass transfer of the organic substances that are transported from the bulk of the solution to the anode surface.
Additionally, the standardized effect for the j shows an inverse effect on color removal for the studied range, contrary to what it was expected. In average, the color removal at 60 mA cm 2 was 3.84% higher than at 100 mA cm-2. The previous result suggests that there is a limited capacity of pollutant removal at high current densities. In a research by Medel et al. (2012) the maximum removal rate of organic matter was observed at a current density lower than the highest one studied, equal to the behavior obtained in the present research. This behavior can be explained by the presence of excessive foam provoked by the large amount of gases produced during the treatment at 100 mA cm-2 (mainly H2, O2 and CO2), creating additional limitations to the mass transfer in the surroundings of the anode and disfavoring the electro-oxidation of the organic matter mediated by the hydroxyl radicals.
Pilot scale Tests
For the pilot scale test the initial pH was fixed to 10.4 as it is the pretreated vinasse initial pH. Also, the maximum allowed volumetric flow, corresponding to a Re of 39,700 was used, minimizing the mass transfer limitations.
The current densities studied in the laboratory scale tests evidenced limitations in the process at high current densities; therefore, an additional lower current density level (35 mA cm-2) was used. The highest current density level of 100 mA cm-2 was maintained to verify the arise of the foam limiting effect in the pilot scale setup.
At 60 mA cm 2 the highest rate of COD and color removal was obtained, as shown in Table 3, evidencing a positive effect when increasing the current density from 3 5 to 60 mA cm2, but proving the limitations of the process when operating at a current density of 100 mA cm-2. The foregoing can be explained by inefficiencies in the electro-oxidation process that occurred when operating at high current densities caused by a higher recombination rate of hydroxyl radicals in the lateral reaction of 02 evolution (water oxidation at the anode). Additionally, the generation of large amount of gases in the reactor can reduce the contact area between the electrodes and the electrolyte increasing the mass transfer limitations in the cell (González, Domínguez, Palo, Sánchez-Martín, & Cuerda-Correa, 2011).
In Figure 4, it can be observed that the color removal for the tests at 60 and 100 mA cm-2 occurs rapidly until 5 A h dm-3, where most of the compounds responsible for the color, such as melanoidins, are degraded. At higher specific electric charges, the behavior is asymptotic due to the lower concentration that increase the mass transfer limitations for the approach of these compounds to the anode vicinity where are oxidized via hydroxyl radical. This means that if the process focuses only in the color removal, applying a specific electric charge around 7.2 A h dm-3 is enough. Additional color removal of 5% causes an increase of 3.5X in the energy consumption. Furthermore, for the lowest current density (35 mA cm-2), the trend is lineal during the entire process, evidencing that the process is controlled by charge transfer.
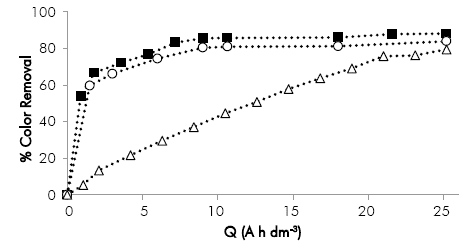
Figure 4 Effect of the current density on color removal as a function of the specific electric charge applied to the electro-oxidation process. Conditions: initial pH = 10.4, Re = 39,700. ¡ = (∆) 35 mA cm 2, (■) 60 Ma cm-2, (o) 100 mA cm-2.
The COD removal reaches values of up to 81.3% at 60 mA cm 2 applying a specific electric charge of 25.2 A h dm 3 with an energy consumption of 173.19 kWh m-3.
From an economic point of view if the purpose of the treatment is the removal of most (more than 80%) of the dissolved organic matter, the industrial application will not be feasible. Thus, the partial electro-oxidation (applying a specific electric charge under 7.2 A h dm-3) focused in the color removal of the effluent, will be the only applicable operational condition for the industrial scale up of this treatment, since color removal rates higher than 80% are obtained with energy consumptions lower than 50 kWh m-3.
A possible application of the partial electro-oxidized vinasse is the recirculation to the fermentation process, reducing the industry net water consumption. The electro-oxidation also decreases the concentration of melanoidinas in vinasse. Being a non-selective process, electro-oxidation can reduce the amount of fat acids inhibiting the activity of yeasts (Castro, 2009). Additionally, it is possible to take advantage of the presence of residual sugars (sucrose and inverted sugars) in partially electro-oxidized vinasse if it is used as dilution water.
The oxidation of the organic matter was also analyzed through UV-Vis spectrums, shown in Figure 5 for a specific electric charge applied of 7.2 A h dm 3. Spectrums show an important decrease of the absorbance within the visible range of 450-800 nm, suggesting the almost complete degradation of color-causing compounds. Nonetheless, in the UV spectrum between 200 and 400 nm high absorbance values were observed in the treated samples, indicating the presence of persisting aromatic intermediate compounds (Martínez-Huitle & Brillas, 2009).
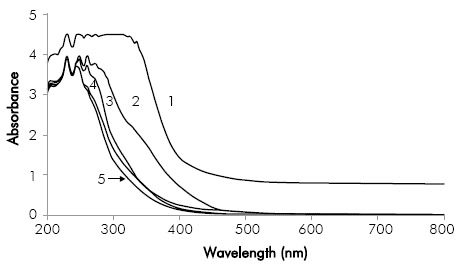
Figure 5 UV-Vis Spectrum before and after applying a specific electric charge of 7.2 A h dm-3 in the electro-oxidation process. Conditions: initial pH 10.4, Re 39,700. (1) Original Vinasse; (2) Pretreated Vinasse (3) ¡ = 35 mA cm-2; (4) ¡ = 100 mA cm-2; (5) ¡ = 60 mA cm-2
In Figure 6 the results obtained in the tests conducted with NaC1 and Na2O4, at 60 mA cm-2 are compared. The color removal is favored using NaC1 towards the end of the process. However, until 10 A h dm-3 removal was higher with Na2SO4. This phenomenon can be attributed to the pH of the solution that affect the oxidant species produced in the bulk solution during the electrolysis with NaC1. During the first stage of the process the pH is basic above 8 units and the predominant oxidant is the hypochlorite ion (OC1-), however the pH goes below 8 units after 10 A h dm 3 and the formation of the hypochlorous acid (HOC1) is favored, which has a higher oxidation potential and thus the discoloration of the samples increase (Panizza & Cerisola, 2009).
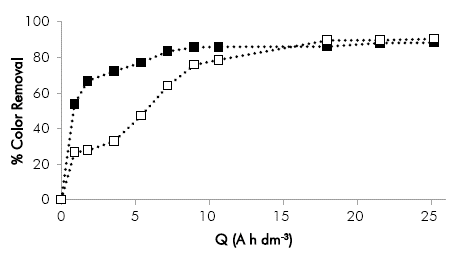
Figure 6 Effect of the supporting electrolyte on the color removal as a function of the specific electric charge in electro-oxidation process. Conditions: initial pH 10.4, Re 39,700. (■) 60 mA cm-2 with Na2SO4, (□) 60 mA cm-2 with NaC1.
Nonetheless, mineralization of organic pollutants is higher using a chloride-free electrolyte. This suggests that the electro-oxidation in presence of chlorinated compounds is not complete and persistent intermediate compounds are generated (Costa, Montilla, Morallón, & Olivi, 2010).
The UV-Vis spectrums of the treatments at 60mA/ cm-2 with Na2O4 and NaC1 are shown in Figure 7a. In the test conducted with NaC1 the peak at a wave length of 289 nm is attributed to the presence of different derivatives from chlorine ion (Pope, Hansen, Bayes, Friedl, & Sander, 2007). Additionally, it suggests the possible formation of toxic persistent chlorinated organic compounds absorbing in the wave lengths between 254 and 366 nm (Lekic & Korac, 2000) (Diaz, Ibáñez, Gómez, Urtiaga, & Ortiz, 2011).
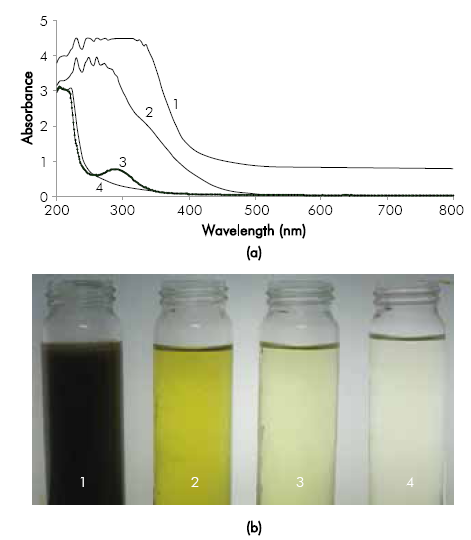
Figure 7 a) UV-Vis Spectrum before and after applying and specific electric charge of 25.2 A h dm-3 in the electro-oxidation process with different electrolytes. Conditions: initial pH 1 0.4, Re 39,700 and ¡ = 60 mA cm'2, b) Photograph of samples: (1) Original vinasse; (2) Vinasse Pretreated (3) Electro-oxidation with Na2SO4; (4) Electro-oxidation with NaC1.
5. Conclusions
The recalcitrant compounds that produce the dark coloration of the vinasse, were treated effectively by electro-oxidation with BDD anodes at a pilot scale achieving color removals higher than 80% with specific energy consumptions lower than 50 kWh m-3.
The electro-oxidation with BDD electrodes was diminished at high current densities due to the generation of large amounts of gases that difficult the mass transfer in the liquid phase, decreasing the oxidation of organic compounds in the anode. Therefore, the use of current densities equal and higher than 100 mA cm 2 should be avoided.
The initial pH did not change the process effectiveness; therefore, this variable does not require a pre-adjustment, reducing reagents dosage.
The higher COD removal rates were obtained using the Na2O4 electrolyte instead of NaCl. Avoiding the formation of toxic organic chlorinated intermediates, regularly present in chlorine ion mediated processes.