1. INTRODUCTION
Unconventional oil plays with considerable resources are receiving increasing attention [1]-[5]. At the end of 2010, the total proven remaining oil reserves in the global conventional petroleum plays amounted to 1888xl08t, accounting for 40% of the global oil and gas reserves [6], The primary development of future resources will shift from conventional to unconventional. Currently, tight oil has achieved a breakthrough in the Bakken (Williston Basin, North America) [7]-[8] and the Eagle Ford formations [9], According to a 2012 prediction from the International Energy Agency (IEA), the remaining global recoverable resources of tight oil are 327x108 t, which is exploitable with the proper technology [10], Besides shale gas, tight oil is considered the most practical unconventional successor for conventional oil and gas [11]-[12].
Tight sandstone is a reservoir which porosity is less than 10% and air permeability less than lmD [13]. Tight sandstone reservoirs have undergone complex diagenetic evolution and structural changes [14]. The pore structure of tight sandstone reservoirs with small pore throat diameter, poor connectivity and strong heterogeneity is different from that of conventional sandstone reservoirs. [15]-[17] The pore system of tight reservoirs is mainly micron - nanometer pore throat system, and the nanometer orifice throat is dominant [18]. Quantitative diagenesis evaluation of sandstone reservoirs is still debated [19]. A tight sandstone reservoir is strongly heterogeneous and difficult to explore and develop [20]-[21]. The development of effective reservoirs has an important influence on tight oil and gas production. Therefore, the quantitative evaluation of the diagenesis in tight sandstone reservoirs is important for oil exploration [22].
The Yanchang Formation in the southern Ordos Basin has rich resources [23]-[24]. The reservoir in Chang 8th member is a typical tight sandstone reservoir, in which oil and gas are mainly concentrated in the micro-cracks and reservoir sweet spots [25], Researchers who studied these reservoir sweet spots in the past [26]-[27] believe that early sedimentation determines the type and intensity of diagenesis and that late diagenesis primarily drives reservoir densification [28].
The Binchang area in the southern Ordos Basin is a Sinopec exploration area; It is expected that by the end of 2011 the Chang 8th member reserves will be 95.72 million, and its forecast reserves will be 34.63 million t. Numerous wells have produced oil economically and good prospects have been identified in the study area. Current exploration and research activity in the Binchang area is low; therefore, an in-depth study of the diagenesis characteristics and evolution in the Chang 8th member is required to predict promptly the occurrence of effective and high-quality reservoirs.
2. THEORETICAL FRAME
The Yanchang Formation mainly developed as a delta sedimentary system within a continental basin; the basin can be divided into six first-order tectonic units [29]-[30] the Tianhuan syncline, the fault-fold belt at the western margin, the Yimeng uplift, the Yishan slope, the Jinxi fault-fold belt and the Weibei uplift (Figure 1). This lacustrine basin is elongated in the north-south direction, and it is shallower and steeper in the northern area. The main sediment source during the early period of deposition was to the southwest of the basin. In the middle and late periods of deposition, the main sediment sources were to the northeast [31]-[32]. Binchang is located at the structural junction of the Yishan slope and the Weibei uplift (Figure 1a). The study area is not structurally developed, but a small anticline has formed in the western study area [33]-[34].
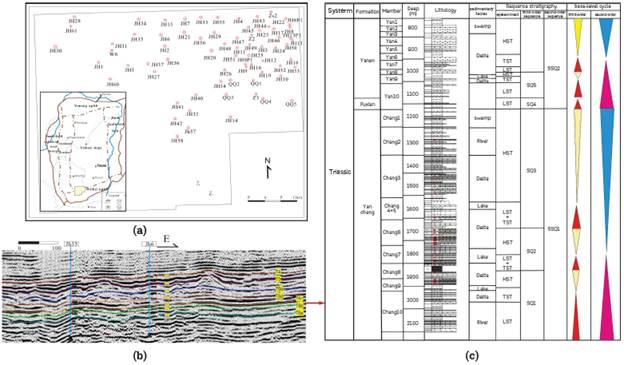
Figure 1 (a) Location map of the Binchang area in the Ordos Basin, (b) Seismic profiles in the studied area (c) The generalized stratigraphy of the Binchang region in the Ordos Basin.
The burial depth of the interior of the basin reached its maximum during the Yanshan movement in the Late Cretaceous (Figure 1b), when most of the Yanchang Formation was below 2100 m. Subsequently, oil and gas began extensive migration and accumulation [35]-[36]. In the late stage of migration and accumulation, the entire basin was uplifting to the southeast, and this tectonic tilt continues to date [37]-[38].
The Yanchang Formation, in the southern Ordos Basin, is divided into one first-order sequence (the Yanchang Formation), two second-order sequences (Chang 10 to Chang 7 and Chang6 to Chang1), and four third-order sequences (Chang10 and Chang 9, Chang 8 and Chang 7, Chang 6 to Chang 4+5, and Chang 3 to Chang 1) [38]-[39] (Figure 1c).
Previous studies indicated that sedimentary facies control the original physical properties of the reservoir at a microscopic scale of Yanchang Formation [40]. Chang 8th is the main oil-bearing layer in the Binchang area. According to the oil and gas show and test data (from Huabei Petroleum Bureau, Sinopec), the northeast study area is the main oil-producing area. The date of source rock correlation shows that the seventh member of the Yanchang Formation, just under the Chang 8th member, is the main source rock of Chang 8th reservoirs [41]. The Chang 8th reservoir of the Yanchang Formation is dominated by delta-front sedimentation, where a subaqueous distributary channel and distributary channel bay are developed. The underwater distributary channel (Figure 2) frames the reservoir and is the most favourable reservoir development zone.
3. EXPERIMENTAL DEVELOPMENT
In the study, numerous drillings for oil have taken place in this area There is plenty of exploration and production well data to support this paper, mainly derived from 43 wells.
400 rock composition data, 400 grading data, 2386 porosity and permeability data, 200 mercury injection capilary pressure testing data and well logging data sets for 137 wells were obtained from the research Institute of HuaBei Oilfield Company, Sinopec,
Samples of different lithology and sedimentary microfacies were selected from the Chang 8th member cores of 43 wells. For the Lithology analysis, diagenesis and pore characteristics, 200 polished thin sections and 400 blue or red epoxy resin-impregnated thin sections were grinded. Thin sections were coloured by Alizarin RedS and K-ferricyanide for calcium carbonate cementation identification Furthermore, 122 fluorescent thin section were used for carbonate mineral identification. Point counting was carried out on 45 thin sections to quantitatively analyze rock composition data, all types of pores and carbonate cements. 44 representative samples were observed by scanning electron microscope (SEM), which was equipped with an energy dispersive X-ray spectrometer. The cathodoluminescence experiment of 122 samples was performed, using an Olympus microscope equipped with a CL8200-MKS CL instrument. In addition, 3-D seismic data from the study area was interpreted, and well log and drilling data froml37 wells was statistically analyzed. Point counting, fluorescent thin section, and scanning electron microscope observations were carried out in the Key laboratory of SINOPEC Exploration Production Researcher Institute.
4. RESULTS
LITHOLOGY
The lithology analysis of the Chang 8th reservoir shows that the LithoLogic components Include 21%-62% quartz (average 36.15%) 15%-47% feldspars (average 30.83%), which are mostly in the K-feldspar (20.8%) and plagioclase (7.1%); and 16%-56% rock fragments (average 33.02%), which are mostly volcanic rock (17.03%) and metamorphlc debris (6.7%). According to the Folk classification, the Chang 8th sandstones are mostly feldspathic Lltharenites and lithic arkosic sandstones (Figure 3a), indicating that this region has a lower sandstone compositional maturity.
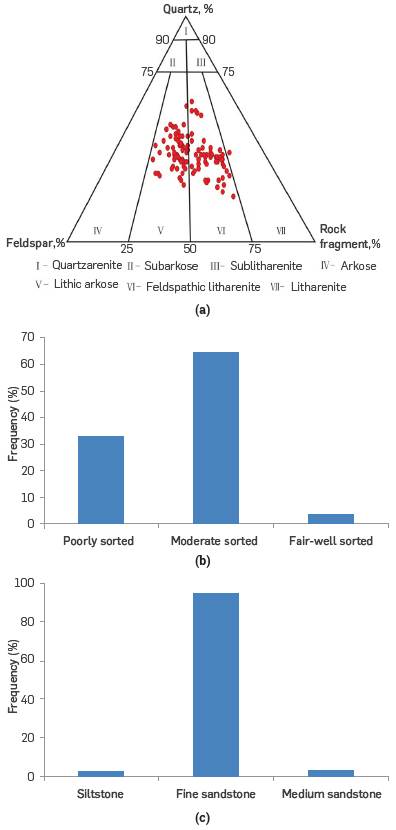
Figure 3 Detrital rock composition, lithology and sorting distribution of the Chang 8th member in the Binchang region, (a) Folk (1974) classification of the sandstone; (b)Sorting distribution; and (c) Lithology distribution.
The sorting degree is moderate (Figure 3b), where fine-grained sandstone is dominant (Figure 3c), and the high content of feldspar and dissoluble lithic material provides the material basis for the formation of dissolution pores.
RESERVOIR PROPERTIES
POROSITY AND PERMEABILITY
The porosity (measured from 406 thin sections) and the permeability (measured from 405 thin sections) data show that the porosity ranges from 1.2% to 19.8% (average is 6.4%) (Figure 4a) and the permeability ranges from 0.013 mD to 9.757 mD (average is 0.362 mD) (Figure 4b). The Chang 8th member is a low-porosity and low-permeability reservoir.
Based on the comprehensive analysis of the log, core and test data, the relationship between the porosity and the burial depth of the well (Figure 4c) and the relation between the permeability and the buried depth (Figure 4d) are established. Approximately between 0 m and 1000 m, the porosity rapidly decreases from 35% to 15% due to compaction, and the pore type is mainly intergranular. Between 1000 m and 1100 m, the porosity decreases slowly to approximately 10%, as the depth increases due to mechanical compaction, cementation and dissolution. The primary pore type is intergranular followed by a smaller number of secondary and intergranular pores. Between 1300 m and 1400 m, the porosity slightly increases with depth, which is related to the formation of secondary pores from the transformation of montmorillonite to illite or chlorite. The pore types are intergranular and secondary dissolution. Between 1400 m and 1500 m, the porosity gradually decreases with increasing depth due to the cementation of calcite. Between 1500 m and 1600 m, the porosity increases with depth due to the source rocks discharging organic acids that dissolve the cementation and clastic particles, forming the secondary pores; microscopically, the dissolved pores are filled by asphalt, indicating that the timing of the secondary pore development is concurrent with the oil accumulation and migration. Below 1600 m, as the burial depth increases, the cementation and pressure dissolution gradually increase, and the porosity gradually decreases. From the above analysis, secondary pores developed between 1300 m and 1400 m and between 1500 m and 1600 m. In addition, porosity and permeability have a good correlation (Figure 4e). Further, the better the physical properties, the better the oil content (Figure 4f).
PORE TYPES AND CHARACTERISTICS
Thin section and scanning electron microscopy observations (from 400 thin sections and 200 images) show the pores types in the Chang 8th reservoir: primary pores (Figure 5a), secondary solution pores (Figures. 5b,c,e), and micro-fissures (Figure 5c). The dissolution pores and the remaining inter-granular pores primarily create porosity. The primary pores are those remaining after compaction and cementation, and the primary pore edges are flat and triangular; these pores occupy 14% of the plane porosity. The dissolution pores include intra-granular dissolved pores and inter-granular dissolved pores. Inter-granular dissolved pores create the primary reservoir space in the Chang 8th reservoir, and the dissolution pore edges are irregular; intergranular dissolved pores occupy 75% of the plane porosity. Microcracks include shrinkage joints and mica cleavage joints, playing a minor role in controlling reservoir properties. The primary pores are generally well connected; however, because of continued compaction and cementation, the reservoir is becoming denser and smaller. The late widespread carbonate cementation further densified the Chang 8th member (Figure 5b). The secondary pores related to the dissolution of feldspar (Figure 5e) and other lithics (Figure 5d) and the micro-fractures (Figure 5c) considerably increase the quality of the reservoir [42]; furthermore, the inter-crystalline pores within clay minerals contribute to porosity (Figure 5f).
In Figure 5: (a) Thin-section micrograph showing a primary pore and enlarged intergranular pore; (b) thin-section micrograph showing a dissolution pore; (c) thin-section micrograph showing a micro-fracture; (d) thin-section micrograph showing partly dissolved cuttings; (e) thin-section micrograph showing a completely and partly dissolved feldspar; (f) SEM micrograph showing an intra-crystalline pore.
The statistical analysis results indicate that the average pore-throat radius of the Chang 8th reservoir is 0.032 urn; The smallest pore-throat is the limiting factor of the reservoir properties [43]-[45], The mercury injection capillary pressure data show that the pore-throat radii range from 0.18um to 0.89um with an average of 0.316um; the displacement pressures are high and range from 0.41MPa to 43.19MPa with an average of 5.86MPa; and the median pressures are high and range from 1.72MPa to171.35MPa with an average of 33.48MPa. Reservoir pore and pore-throat sizes are small and unfavourable for fluid migration and accumulation, and the percolation is poor.
DIAGENETIC CHARACTERISTICS
The Chang 8th tight member has undergone multi-stage superimposed modification, including compaction, authigenic quartz, carbonate cementation and clay mineral, leading to reservoir porosity decrease, but dissolution, oil invasion and chlorite film generation led to reservoir porosity increase.
COMPACTION
The Chang 8th reservoir in the Binchang area underwent a medium-intensity compaction, which is the most important factor of reservoir densification. Most of the particle boundaries are in-line contacts and concavo-convex contacts (Figure 6a). Under mechanical compaction, the mica deformed and squeezed between particles.
QUARTZ CEMENTATION
The syntaxial overgrowth and pore-filling cement are the main types of authigenic quartz developed in the study area (Figure 6c). Authigenic quartz is related to the formation of kaolinite and subsequent release of SiO2 by the k-feldspar, plagioclase, tuffaceous detritus and granite debris dissolution in the acidic environment [46]-[47] Authigenic quartz occupies pore space, which decreases the reservoir quality [48]. In the early diagenetic period, syntaxial overgrowth generally grew along the edge of quartz particles, and the authigenic quartz more commonly grew between detrital grains (Figure 6e). In addition, authigenic quartz is always accompanied by the formation of kaolinite, mixed-layer illite/smectite, and chlorite (Figures 6f, 6g). The influence of authigenic quartz on the pore evolution can be neglected, as there is only a small authigenic quartz content in the Chang 8th; therefore, the authigenic quartz is not quantitatively discussed in here.
CARBONATE CEMENTATION
In the Yanchang Formation reservoir of the southern Ordos Basin, which underwent multiple stages of structural subsidence and variations in its fluid properties, carbonate cementation is common in the Chang 8th member, carbonate cementation primarily occurred from the late settlement of the basin to the early stage of uplift. The carbonate cementation, which fills part of the inter-granular pores and intra-granular dissolution pores, has a significant destructive effect on the reservoir [49] (Figure 6b). The relationship between intergranular volume and cement volume shows that mechanical compaction has played a more important effect in destroying the reservoir porosity of the Chang 8th member than cementation (Figure 7).
THE EARLY STAGE OF CHLORITE FILM PRECIPITATION
The authigenic chlorite is observed as a thin-film chlorite structure and a thick pore-lining structure (Figure 6e); the content of chlorite in the Chang 8th reservoir ranges between 1.3% and 8%. The first stage of chlorite film formed in the early diagenetic stage A. The chlorite film can inhibit quartz overgrowth and pressure dissolution increase intergranular compaction strength, and occupy the growth space of carbonate cement [32],[50]-[52]. The chlorite film has a positive effect on reservoir properties. However, as the temperature continued to increase, the mudstone and shale continuously released iron and magnesium, initiating the second stage of chlorite precipitation (Figure 6e) in the pores [53]; consequently, the porosity decreased, and the pore throats narrowed, which had a negative effect on the reservoir properties.
KAOLINITE PRECIPITATION
The authigenic kaolinite in the study, whose content is approximately 2%, occurs mainly as booklets of vermicularly stacked pseudohexagonalcrystals. This kaolinite retains large inter-granular pores and is often associated with authigenic quartz (Figures 6f) The formation of authigenic kaolinite is primarily related to feldspar dissolution and othersilicate minerals [42]. If the system is open, the dissolution ions are removed, and the kaolinite remains in the pores: the intra-crystalline kaolinite pores provide crucial reservoir space in tight sandstone [54]-[57], The kaolinite precipitation occurred after the feldspar dissolution. Moreover, the kaolinite distribution is strongly heterogeneous and was determined by the heterogeneity of the feldspar dissolution and fluid flow.
OIL INVASION
Oil invasion plays a positive role in the evolution of the physical properties of reservoirs [58], mainly attributable to the promotion of acid dissolution, inhibition of late carbonate cementation, and weakening of a second stage of quartz growth; these effects strengthen with oil saturation [28],[48],[59]-[61].
From the inclusion testing data, four periods of oil and gas invasion are identified in the Yanchang Formation: The Late Jurassic (154 Ma to 149 Ma), the Early Cretaceous (143 Ma to 107 Ma), the middle Cretaceous (110 Ma to 30 Ma), and the early Tertiary to the late Tertiary (25 Ma to 23 Ma) (Figure 12). The analysis of 200 fluorescent thin sections shows that the migration channels and storage spaces of the early oil invasion are also the main areas for Later oil and gas intrusion. The early phase of invasion filled the pore throats with dark-brown heavy oil, the second phase of yellow oil filled the pores, and the third phase of yellow-green light oil filled the micro-cracks and granular dissolution pores. In the multiple periods of oil invasion, the reservoir properties continued to improve, and the reservoir heterogeneity also increased. A positive correlation is observed between the porosity of the reservoir and the oil saturation. Additionally, the logging results improve as the physical properties of the reservoir improve. In reservoirs with low oil saturations, the oil invasion cannot effectively inhibit the quartz overgrowth and carbonate cementation [62].
DISSOLUTION
Dissolution is an important diagenetic mechanism of increasing the physical properties of a reservoir. On the one hand, the selective dissolution of particles forms intra-granular pores; on the other hand, dissolution enlarges the inter-granular pores, and the reservoir space increases [63]. According to the chemical properties of a dissolution medium, dissolution can be divided into 2 categories: acid dissolution and alkaline dissolution [59]. Feldspar dissolution is evident in the study area and mainly occurred along the particle edges and cleavage (Figure 6e); later, the dissolution formed moulding porosity. Dissolution of the debris occurred after that of feldspar, and the minerals easily soluble in the debris were subjected to selective dissolution, which formed the intra-granular pores. Due to changes in water conditions, the early calcite cements were eroded and easily replaced by other minerals, partially clogging the pore space (Figure7h). Microscopically, the plagioclase-sourced laumontite (Figure 7i) occupies some of the pore space. As the groundwater gradually became acidic, the laumontite began to dissolve along the cleavage plane, and the physical properties of the reservoir improved. The dissolution of feldspar and debris is usually caused by the uplift of tectonic movements and the charging of organic acids.
5. RESULTS ANALYSIS
PARAGENETIC SEQUENCE OF THE DIAGENESIS
According to thin-section (400) and cathodoluminescence (122) observations, the space between particles is filled with clay (kaolinite and illite), and a small number of inter-granular pores are observed (Figure 6f). The observed chlorite film is closer to the particle surface than the calcite cement (Figure 6e), indicating that chlorite films formed earlier than the calcite cement [64]. The chlorite films disappear at the particle contacts, thus indicating that the chlorite film formed after the initial compaction. A small amount of calcite is observed on the syntaxial overgrowths (Figure 6b), indicating that the calcite formed after the syntaxial overgrowths. The diagenetic environment changed from alkaline to acid. The calcite also encompasses the residual kaolinite, suggesting that the calcite formed after the formation of argillaceous and siliceous cements. Authigenic quartz developed in the pores between the chlorite film (Figures 5b,6c), proving that the formation of the authigenic quartz occurred after the formation of the chlorite film.
Based on the abovementioned studies, the diagenetic sequence is as follows:
Mechanical compaction → Early stage of chlorite film precipitation → Authigenic quartz precipitation → Early stage of calcite precipitation → Illite and needle- and rosette-shaped chlorite precipitation → Feldspar dissolution → Kaolinite precipitation → Late calcite cementation
QUANTITATIVE EVALUATION
PRIMARY POROSITY
The original porosity was calculated by an empirical formula [65].
where S refers to the sorting coefficient and Φ0 refers to the original porosity. The original porosity of the Chang 8th reservoir is mainly between 30.1% and ~41.7%, with an average value of approximately 34.3%.
POROSITY REDUCTION DUE TO COMPACTION
According to the quantitative statistics of the thin sections, the porosity after compaction can be calculated by the following formula.
where Φ1 refers to the porosity after the compaction, △Vcem refers to cement content, Spre refers to the plane porosity of the primary intergranular pores, Scem refers to the plane porosity of cement, Φnow refers to the experimental porosity, and So refers to the total plane porosity.
where ΦL refers to the porosity decrease due to compaction and <t>c refers to the primary porosity.
The reservoir porosity substantially reduced due to compaction; it decreased an average 24.8% from the original porosity (Figure 8).
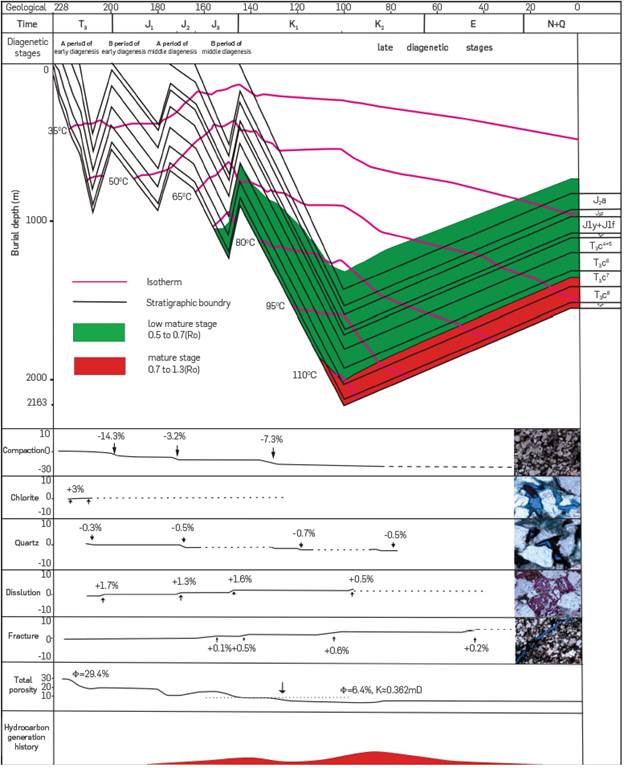
Figure 8 Thermal, burial, and diagenetic history and the porosity evolution of the Chang 8th sandstone reservoir in the Binchang area.
POROSITY REDUCTION DUE TO CARBONATE CEMENTATION
where Φ 2 refers to porosity after cementation and Φ c refers to the decrease in porosity due to carbonate cementation.
Carbonate cementation resulted in an average 8.2% decrease in the reservoir porosity.
POROSITY REDUCTION DUE TO DISSOLUTION
where Φ 3 refers to the increase in porosity due to dissolution, SIntra refers to the intra-granular dissolution from the plane porosity, and SInter refers to the inter-granular pore dissolution from the plane porosity.
The porosity increased by approximately 5.1 % due to dissolution (Figure 8).
INFLUENCE OF OIL INVASION ON RESERVOIR
The porosity of reservoirs is positively correlated with oil saturation. The higher the oil saturation, the greater the porosity and permeability (Figure 4F). Four stages of large-scale oil invasion were taken in the study area. Early oil invasion is conducive to the protection of pores, which become the best space for the later large-scale oil invasion. The second time intervals of oil invasion in 122 Ma -110 Ma are large and last for a long time. Some reservoirs inhibit the diagenesis at this stage, which has significant influence on the pore evolution.
According to hydrocarbon inclusions data the homogenization of inclusions is mostly distributed in 70 OC-100 OC and 100 OC-130 OC.
Based on the historical analysis of reservoir formation in the study area (Figure 8), there are two stages of oil accumulation in the reservoir, which are 155 Ma -150 Ma and 140 Ma -120 Ma. Through the relationship between reservoir evolution and hydrocarbon charging, it can be concluded that during the early and middle stages of oil accumulation in reservoir, the reservoir is not densified; however, regarding oil accumulation in the late stage, the reservoir is in the densification stage.
CONCLUSIONS
The Chang 8th sandstones are mostly feldspathic litharenites and lithic arkosic sandstones with low sandstone compositional maturity and relatively low textural maturity.
The Chang 8th reservoir properties are poor, with low porosity and permeability, small pore-throat radii, and high displacement and median pressures. Moreover, the physical properties are strongly heterogeneous, both laterally and vertically. The pore structures mainly consist of secondary dissolved pores and residual inter-granular pores.
Compaction, which reduced the reservoir porosity by 24.8%, and carbonate cementation, which reduced the reservoir porosity by 8.2%, are the main causes of densification in the Change 8 reservoir. Compaction has played a more important role than cementation in decreasing the reservoir quality. Dissolution, which increased the reservoir porosity by 5.1 %, is the most important factor for increasing the reservoir porosity. In addition, chlorite plays an active role in inhibiting secondary quartz growth and preserving primary pores.
Two stages of oil accumulation in the reservoir can inhibit the diagenesis, which has a great effect on preserving primary pores.