1. INTRODUCTION
The Eocene Mirador Formation, along with the late Cretaceous upper Guadalupe and Paleocene Barco Formations, are the primary reservoir rocks in the Foothills and Llanos Basin of Colombia. The Mirador Formation is the main producing reservoir in the Cusiana, Cupiagua, and Volcanera oil Fields [1]-[3].
The lower Mirador Formation of the Cusiana oil field consists of fluvial, amalgamated, coarse-grained sandstones and mudstones deposited in incised valleys, and the upper Mirador Formation are sandstones and mudstones deposited in estuarine channels and bay-head deltas [4],[5],[1],[2]. In the Cupiagua Field, the lower Mirador Formation comprises channel and crevasse sandstones and swamp mudstones, and the upper Mirador, sandstones, and mudstones of bayhead deltas and bayfill environments, respectively [3].
The Mirador Formation was mapped as a continuous unit along the Foothills of the Eastern Cordillera and the Llanos Basin [4]-[6],. This unit was mapped up to the Caño Limón oil field , northeast of the Llanos Basin, where the Mirador Formation was called the "basal sands of the Carbonera Formation" and described as deposited in a deltaic environment [7],[8].
Bayona et al in [9], reintroduced the concept of "Basal sandstone unit of the Carbonera Formation, as they considered that the Mirador Formation should be restricted to the Foothills area, and the basal sandstones to equivalent units in the Llanos Basin. Other authors consider the diachronous onset of "Mirador-like units," as was proposed in the central Llanos by Mondragón [10], who named it as a new, C09 - member of the Carbonera Formation. The Mirador Formation continuation along the basin, lithology, depositional environment, and age, have been challenged [11]-[13].
Some marker beds in the Llanos basin have been identified as potential clues about the age of the reservoirs. These correspond to paleosols that usually develop when the substrate (any substrate) is exposed subaerially but could also include sediment material from volcanic ashfalls [14], that if possible to date could provide relevant data on the stratigraphic framework of the basin fill.
In the western SLLB, several studies have redefined the reservoir rocks in the Chichimene, Castilla, and Apiay oil fields. Sandstones of the Mirador, Barco, and Guadalupe formations are part of the primary reservoir rocks and contain over 5000 MMBLS proved in these fields [15]-[18]. However, few facies analyses have been carried out on the sandstones of the Mirador Formation [19],[20].
Essential questions such as the age of the Mirador Formation, its continuation and architecture of the reservoir to the east, distribution and thickness of the sandstone facies, and configuration of the ancient sedimentary environment are in the mind of exploration and production geologists in the oil industry to aid in the exploration and production work.
The primary purpose of this work is to present a detailed description of facies, facies successions, their distribution, and the depositional environment configuration of the Eocene rocks (Mirador Formation and lower part of C8 member of the Carbonera Formation) in the southern Llanos basin. We also present the U-Pb detrital zircon ages for two critical stratigraphic markers, two partially volcaniclastic paleosols, one at the base of the lower Mirador, and the other at the top of the unit.
Based on the foregoing, we present a regional correlation involving surface outcrop data from the Foothills and wells in the basin. In this correlation, we show the reservoir distribution to the east, its environment evolution and the stratigraphic framework for the Mirador, and C8 member of the Carbonera formations in this part of the basin.
2. THEORETICAL FRAME
GENERAL EVOLUTION OF THE FOOTFHILLS AND LLANOS BASIN
Nowadays, the Llanos Basin is a foreland basin, and its geologic evolution was actively controlled by the tectonics of the western margin of South America (Figure 1, inset). The diachronous, late Mesozoic-Cenozoic collision of a magmatic arc in the Caribbean Plate, with the South American plate, was instrumental in the Eastern Cordillera uplift and the Llanos Foreland basin formation in Colombia [21],[22]. See Mora [22] for a more detailed tectonic evolution of the Eastern Cordillera and Llanos Basin.
Recent works have shown tectonic deformation and uplift during the late Paleocene and earliest Eocene in the Central Cordillera, Magdalena Basin and western flank of the Eastern Cordillera [23]-[28]. In parallel, other works identified the reactivation of older structures on the eastern flank of the Eastern Cordillera [26], [29]. In SLLB, the Mirador Formation is in unconformable contact over Cretaceous rocks. The stratigraphic configuration indicates Paleocene tectonic activity in this part of the basin [29].
The tectonic activity of the Paleocene continued into the early Eocene [25],[29],[26]. In the latest early Eocene, a fluvial sedimentary system established in the Llanos basins, and deposition of the lower Mirador took place in this area. During the middle-late Eocene, tectonic deformation and loading advanced to the Eastern Cordillera [30],[26],[31],[32],[33] and the Santander Massif began to uplift, initially from the northwestern sector towards the southeastern area of the Massif [27],[28],[34]. During middle Eocene to late Eocene, transitional to marine environments established in the central part of the Llanos Basin and the Eastern Cordillera [35].
The Mirador Formation is diachronous in the central and northern Llanos Basin, as this unit is early to middle Eocene in the Llanos Foothills and Oligocene to the east in the foreland basin [13]. In the southern Llanos Basin, however, the Mirador Formation was dated as middle Eocene. The Mirador Formation in the Cusiana Field was interpreted as an incised valley fill after a period of relative sea-level fall in the late Paleocene [4],[1],[2].
Paleogeographic reconstructions of the Cenozoic in Colombia show lower-middle Eocene strata of the Mirador dominated by sandy fluvial facies in the Foothills and Llanos basin and middle-upper Eocene by mudstones and sandstone, along an NNE elongated fluvial system with periods of marine ingression [36]. This late Eocene depositional configuration continued in the Oligocene, with accumulation of mudstones of the C8 member of the Carbonera Formation covering most of the basin with the deposition of coeval sandstone deposits toward the easternmost part of the basin.
3. EXPERIMENTAL DEVELOPMENT
The description of facies and facies successions was made in three measured stratigraphic sections at outcrops in the creeks of the Acacias area, nine well cores of the Chichimene-Castilla oil fields, and eleven well cores along the eastern side of the Llanos Basin, from Chichimene to the Camoa oil field and eastward. In this paper, we show information from the Sagú Creek section and nine well-cores in the Llanos Basin (Figure 1).
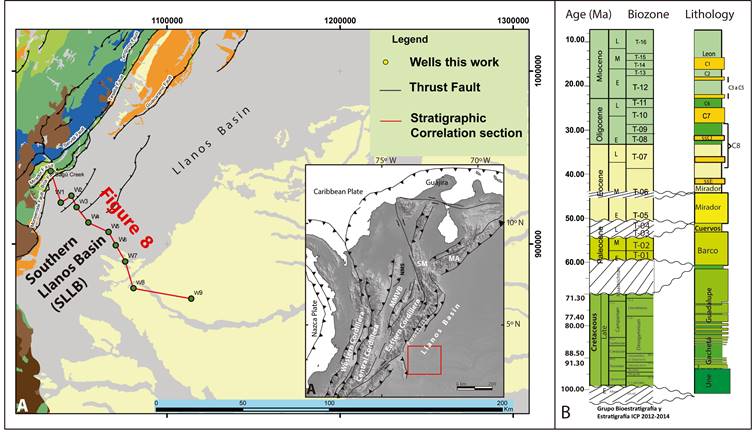
Figure 1 Location of the Southern Llanos Basin (SLLB). (a) Outcrop and well correlation between the Foothills of the Eastern Cordillera (Sagú Creek) and the foreland basin. Location of wells in correlation of Figure 8. (b). Chronostratigraphy of the Southern Llanos Basin. Main unconformities identified, the unconformity below the Paleocene rocks, the unconformity below the Eocene rocks, and the transgressive ravinement surface at the top of the lower Mirador Formation.
"A facies is a body of rock characterized by a particular combination of lithology, physical and biological structures that bestow an aspect ("facies") differing from the bodies of rock above, below and laterally adjacent" [37].
Grain size, primary sedimentary structures, type and degree of borrowing and interbedding were studied in cores and outcrops. These descriptions also included the composition of special features (framework grains, duricrusts, nodules, diagenetic material). The Bioturbation Index [38] was used to describe the degree of bioturbation in facies. Bioturbation is the disturbance of sedimentary deposits produced by animal and plant activity. Bioturbation includes burrowing, ingestion, and excretion of sediment grains. Ichnofossils and bioturbation were described following the guidelines and criteria of [39]. Paleosol identification and nomenclature are based in the work of [40], but also a useful classification nomenclature of paleosol is found in [41].
Sedimentary structures are some of the most valuable data for interpreting the depositional environment of the rocks. The structures are especially useful in determining energy, flow regime and biological activity, and are often the only way to define sedimentary processes by which sediment is deposited [42].
We described the facies following the guidelines of [43]. In this methodology, the first part of the name of the facies consists of a two-letter symbol; the primary texture is in uppercase, the surrogate in lowercase. The second part is in lower case and corresponds to the physical sedimentary structure. Sedimentary structures include ichnofossil or other attributes that are important in defining the facies. (see Figure 12 in [43]).
The genetically related facies occurs in conformable contact, shows no difference in lithology and only minor differences in sedimentary structures. For example, sandy conglomerates are present as a differentiable lithology, but they could have different sedimentary structures in the same bed set. These facies were combined into facies associations, as they are genetically related to all others, and likely, they record almost the same sub-environment of deposition [44]. For example, the sandy conglomerate facies related to the channels and bars in a braided system can be massive, plane laminated, or cross-bedded, but these gravels where deposited in the same fluvial environment. i.e., on a longitudinal bar, sidebar, or high stage vs. waning flood in a fluvial cycle and, therefore, are genetically related.
In this paper, we describe all related facies first, and then we ordered them into the natural facies succession to analyze them in such context. Facies successions are conformable facies taken in the vertical context. As such, facies show a progressive change from one to another, in one or more parameters of the facies; i. e., thickness, abundance of sand vs. mud, grain size, type of sedimentary structures [44], [37]. In a vertical facies succession, if the facies at the base is gradational with the next one and this to the next until the top, these are the result of one related set of depositional conditions. According to Walker [44], "Experience has shown that, in a broad sense, there are only a limited number of progressive facies successions. Historically, the comparison of many different examples of one type of succession (ancient and modern) has led to generalizations that are the base of facies models."
Biozones identified in the correlated sections were classified at the Biostratigprahy-Laboratory at Colombian Petroleum Institute (ICP), following the palynology zonation established by Jaramillo [45].
The palynology analysis provided information regarding depositional environments. For example, it was possible to distinguish non-marine and marine organic matter. The first one is dominated by continental particles and freshwater algae, whereas marine organic matter contains marine algae, like dinoflagellates and acritarchs. However, we also used the salinity index, which is SI=M/T, where M is the number of marine palynomorphs, and T is the total count (marine plus terrestrial palynomorphs), as was used by Santos [35]. A correlation shows the distribution of facies through the basins, and the salinity index (SI), was used to support depositional environment interpretations.
The Gamma Ray log helped in the identification of sharp vs. gradational trends, changes in stacking patterns, and maximum flooding surfaces. It also provided context for the interpretation. Pattern matching analysis was useful for the correlation.
We present new U-Pb ages obtained from three samples of the two paleosols described hereunder, the intensively-weathered and the compound paleosols. Zircon grains were separated by standard heavy liquid techniques, selected randomly, and analyzed by laser ablation-multi collector-inductively coupled plasma-mass spectrometry at the Isotopic Radiogenic Laboratory at Washington State University (IRL). Gehrels [46], explains the laboratory method used for the analysis UPb of the samples at the WSU-IRL.
4. RESULTS
Fl: SANDY CONGLOMERATE LITHOFACIES (sG m, sG imb, sG x, sG h)
The sandy conglomérate Lithofacies include clast supported pebble conglomerate with a matrix of fine, medium to coarse sand (Figure 2a, 2b). The sandy conglomerate lithofacies shows massive or crude horizontal stratification (sG m, sG h), clasts imbrication (sG imb), and cross-stratification (sG x). Pebbles are rounded to subrounded and composed mainly of quartz and chert (Figure 2b black&gray pebbles). The composition of the sand fraction in the Llanos basin is predominantly quartz.
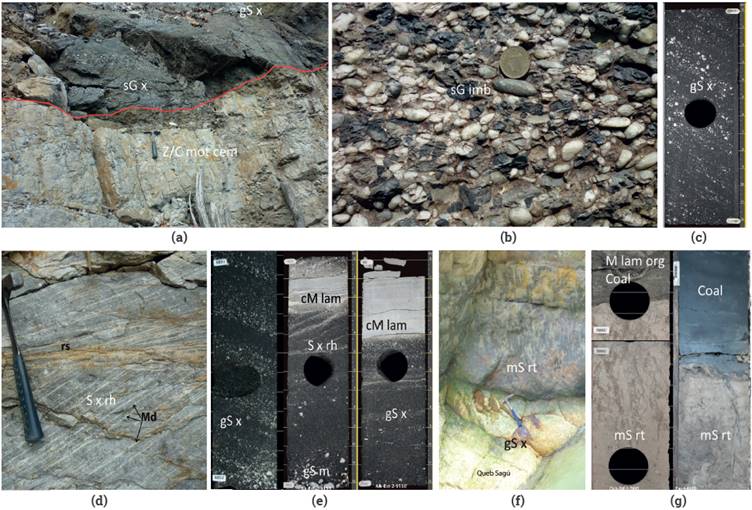
Figure 2 Facies of lower Eocene in the Foothills outcrops and middle Eocene in the Llanos Basin cores, (a): Conglomerate facies (sG x), over intensely-weathered paleosol of the Paleocene (Z/C mot cem); this paleosol defines the unconformity at the base of the Eocene (red line), (b): Detail of conglomeratic facies in outcrop (sG imb). (c): Conglomeratic sandstones with plane cross stratification (gS x) in well core, (d): Cross stratified sandstone with mud drapes (md) and reactivation surface (rs). (e): well core, stratified sandstone (S x rh) with mud drapes (Md), and clayey, laminated mudstone (cM lam) between sets of coarse cross stratified sandstone (gS x; Sx rh). (f) and (g): Outcrop and core image of rooted and mottled muddy sandstone (mS rt), compound paleosol above conglomeratic sandstone (gS x); in cores,this facies could reach 4m and is capped by coal or carbonaceous mudstone (M lam org), above the rooted sandstone. ((a),(b),(d) and (f): Sagú creek, (c), (e) and (g) (left): Acacias-Chichimene. (g): Camoa field.
The contact at the base of the sandy conglomerate lithofacies is sharp and irregular over Paleocene-lower Eocene rocks of the Cuervos Formation. These Paleocene rocks are pedogenized, cemented, and mottled. The contact represents an unconformity (Figure 2a). Sandy conglomerate lithofacies are usually transitional or sharp with sandstone lithofacies. The set thickness of sandy conglomerate lithofacies is from 0.3 to 1.5 m, and bed sets up to 11 m occur by amalgamation.
This sediment is likely to be transported by unidirectional currents. Gravel clasts were transported as bedload by traction, rolling, or under flat-bed conditions in high stage flow; at the same time, the sand is carried in suspension. Gravel clasts were first deposited during the falling stage of the fluvial flooding event and infiltrated later by sand as energy decreases. Cross strata deposited at the margins of gravel bars during the falling phase, but this also occurs when flooded channels are filled in the falling stage of the flood [47], [48].
F2: SANDSTONE LITHOFACIES (gS x, S x rh, S x)
Sandstone lithofacies consists of medium to very coarse-grained sandstone, and medium to coarse-grained sandstone. The framework is composed of subangularto subrounded quartz grains. Sedimentary structures are planar or trough cross-bedded (S x), which could be gravelly sandstone (gS x) with quartz granule to pebble clast, concentrated toward the base of the foresets (Figure 2 c,e. Figures 4 and 5). Rhythmic cross-bedded sandstone (Xx rh), was identified when a mud drape over each foreset is present and allow to see lateral foreset thickness variation and reactivation surfaces (rs) (Figure 2d).
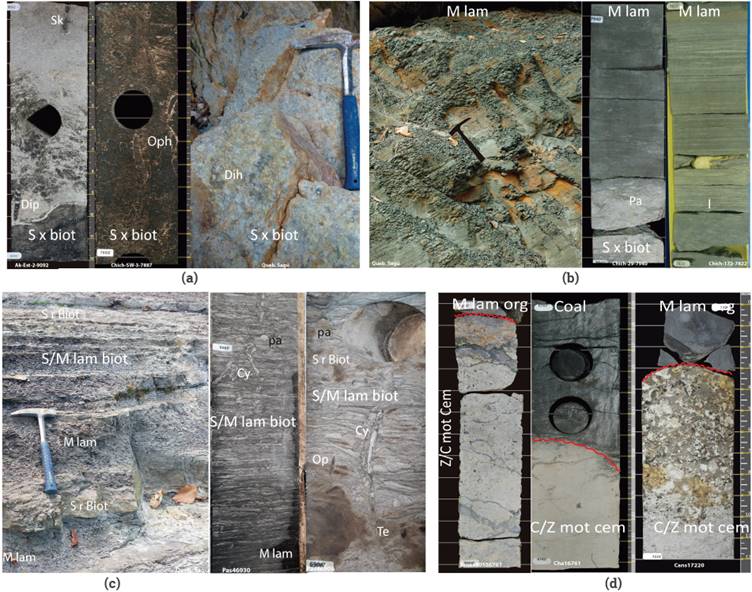
Figure 3 Facies of middle-upper Eocene in SLLB and Foothills, (a): Bioturbated fine to medium cross stratified sandstones (S x biot) by Ophiomorpha (Op), Skolithos (Sk), Diplocraterium (Dip) and Diplocraterium Habichi (Dih). (b): gray to greenish gray, laminated mudstone (M lam) with thin lenses of silt or very fine sandstone (1). (c): Laminated and bioturbated heterolithic fine sandstone/mustone (S/M lam biot) with Cylindrichnus (Cy), Paleophycus (Pa), Teichichnus (Te), Ophiomorpha (Op), (d): Below: Paleocene mottled and intensely-weathered paleosol (C/Z mot cem); above: coal and dark gray carbonaceous mudstone (M lam org). Red line marks the Paleocene/middle Eocene unconformity. Outcrop images (a), (b) and (c) from the Sagú area (hammer is 30 cm long). Cores (a), (b) from the western sector of the SLLB. Cores (c) and (d) from the eastern sector of SLLB.
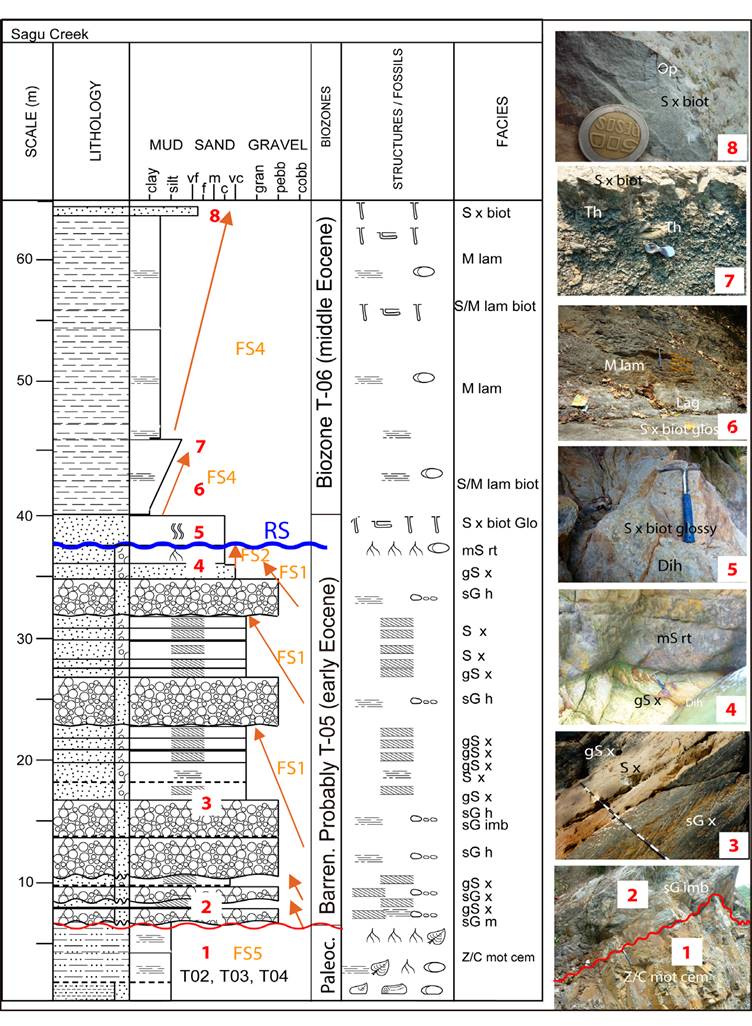
Figure 4 Lower - middle Eocene facies successions in the Sagú creek, Foothills. From base to top, the (FS5) intensely-weathered paleosol (Z/C mot cem) below the unconformity at the base of the Eocene (red line). Above the unconformity, five amalgamated braided conglomerate and sandstones (FS1). The coastal plain compound paleosol horizon (FS2) caps the FS1. The wave ravinement surface (RS) eroded the paleosol and Diplocraterion Habichi (Dih) marks the beginning of marine transgression. Above the ravinement surface, there are two shallow marine facies successions (FS4), (see text for explanation of facies and facies successions).
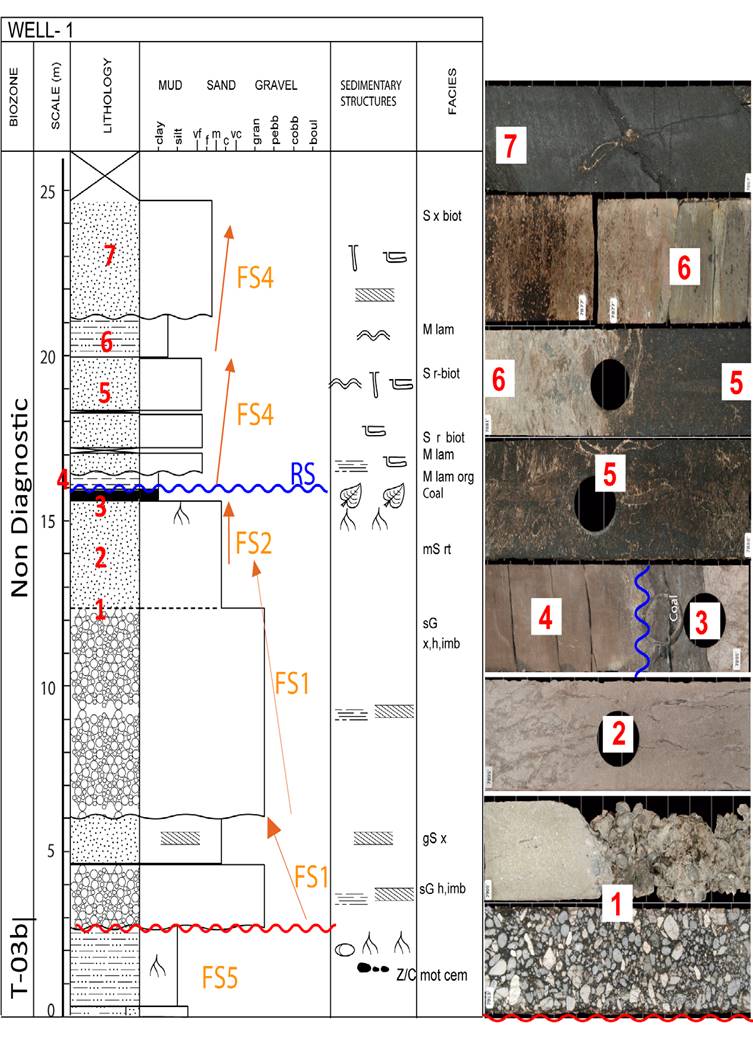
Figure 5 Middle Eocene fluvial and marine facies successions in Well 1, western side of the SLLB. At the bottom the extensively-weathered paleosol (Z/c mot cem), below the unconformity. Two cycles of braided conglomerate and sandstone (FS1). The coastal plain compound paleosol horizon (FS2), capped by a thin layer of coal. The wave ravinement surface (RS) erodes the coal layer. After the ravinement surface, there are two shallow marine facies successions of mudstone and sandstone (FS4). The ravinement surface separates the fluvial from the marine facies.
Sandstone lithofacies sometimes is bioturbated by roots (Figure 2g). The contacts toward the base and top can be gradational or sharp. Set thickness vary from 40 cm up to 1.5m and bed sets up to 11 m in the Llanos Foothills, with rhythmic cross-bedded sandstones predominating in the Acacias and Chichimene area.
The crossbedding results from transport of bedload, by migrating two or three-dimensional dune forms. The crossbedding occurs in unidirectional or bidirectional currents under the lower flow regime [49]. Sandy cross-beds are generated in several environments, and their interpretation depends on the associated facies or other attributes of the facies or the stratigraphie context. It could be formed in fluvial, tidal, marine, or deltaic environments. Rhythmic structures and surfaces mean rapid and cyclic changes from high to low energy, and are probably of tidal origin [50].
When gravel is mixed with sand (gS x), this facies is transported as mixed sand/gravel bed load by traction and rolling processes, under a lower flow regime. These facies are carried underwater, on dunes that migrate in continuous superposition events, often over migrating gravel bars [47].
F3: ROOTED MUDDY SANDSTONE (mS rt)
Muddy sandstone lithofacies consists of medium to coarse-grained, yellowish to cream, massive argillaceous sandstone. The muddy sandstones have root traces, vertical structures of pedogenesis, mottling, and bioturbation (mS rt). (Figure 2g). The texture is poorly selected medium to coarse sand, and the matrix is mud or silt; local undifferentiated vertical burrows can be present. These facies are up to 4 m thick in the Foothills and western side of the SLLB, but to the east, they diminish to less than 1 m thick.
The sandstone lithofacies was subaerially exposed and colonized by vegetation for a long time. Eventually, weathering masked the primary sedimentary structures [40]. Bioturbation also destroyed these primary structures and mixed the sandy and muddy particles (Bioturbation Index BI = 2 to 6). Probably the vegetation that rooted in these sandstones (evidenced by the root traces) was retained as a thin layer of coal, as evolving sedimentary environmental conditions changed later to a rise in the water table (Figure 2g).
F4: BIOTURBATED SANDSTONE LITHOFACIES (S x biot, S r biot).
Bioturbated sandstone lithofacies comprise fine to medium-grained, bioturbated cross-bedded sandstone (S x biot). Bioturbated wave ripple cross lamination (S r biot) overlay the sets of bioturbated sandstone. Borrowing was mainly by ophiomorpha, teichichnus, skolithos, diplocraterion, and cylindrichnus (Figures 3a, 3c right). Bioturbation is variable with the Bioturbation Index: BI = 2 (Figure 3b center core) sparse bioturbation in some beds, to BI = 5 (Figure 3a right, outcrop) intense bioturbation. However, the low diversity of trace fossils predominates. Set thickness from 0.5 to 1 m in the eastern side of the basin; bed sets from 3 to 4 m are present mainly in the western sector of the Llanos basin and seems to increase towards the northeastern sector of the Foothills.
Bioturbated sandstone lithofacies (S x biot, S r biot) have a strong marine influence. The salinity index [35] in the Sagú Creek and Well-1 ranges from 0.075 to 0.19 and 0.16 respectively, and to the east, this SI is between 0.016 to 0.025.
The properties of salinity index, texture, sorting, thickness, and trace fossils, found in the bioturbated sandstones lithofacies suggest a shallow-marine depositional environment [51]. These bioturbated sandstones lithofacies were deposited in low-energy upper shoreface environments [52].
F5: LIGHT-COLORED MUDSTONE (cM lam)
Light-colored mudstone lithofacies consists of white, mid-gray to greenish-gray clayey mudstone (cM lam). The texture of this facies varies between silty shale and muddy siltstone. The predominant physical structures are massive to horizontal plane parallel lamination. Other structures identified are lenticular and wavy lamination, as well as ripples. These lithofacies may include laminae of fine to very fine-grained sandstone. Commonly, the contact is irregular by erosion at the top and sharp at the base of the mudstone as this lithofacies is always between facies F1 and F2. In most cases, its thickness is usually less than 50 cm. (Figure 2e).
These light-colored mudstone lithofacies were deposited by suspension under still water, eventually with the migration of ripples at low flow rates probably associated with shallow lakes or ponds in flood plain settings. High energy during the deposition of sandy or gravelly sediment erodes the F5 facies; furthermore, its preservation is low.
F6: SILT/CLAY MOTTLED, CEMENTED (Z/C mot cem)
The cemented, mottled claystone facies consists of white to greenish-gray to cream, silty cemented claystone (Z/C mot cem). Sometimes the color in these facies is mottled reddish-brown. This hard-mottled mudstone corresponds to an intensely-weathered paleosol, which includes ferricretes or silcretes. Root traces, nodules, and crusts are present (Figures 2a, 3d). The paleosol is 1 to 6 m thick; stratigraphically, it corresponds to the top of the Cuervos Formation in the Foothills and the western SLLB. However, to the east SLLB, this paleosol is developed on Cretaceous and older units. The upper contact of this paleosol represents the subaerial unconformity at the base of the Mirador Formation.
We interpret subaerial exposure of the preexistent substrate, weathering of this material, pedogenization, evolution, and development of the intensely-weathered paleosol, previous to the deposition of the facies above, which should be fluvial. This process could take up to several million years [40]. This type of paleosol forms during a period of landscape degradation and an episode of landscape stability, and is likely an interfluve paleosol that marks a sequence boundary. The genesis of this kind of paleosol is related to allogenic controls such as relative sea-level changes, global or regional climate changes, and regional tectonics. Furthermore, this paleosol marks an interfluvial sequence boundary [53],[40],[41].
F7: CARBONACEOUS MUDSTONE (M org, M lam org)
Dark gray to black carbonaceous mudstone contains pyrite nodules locally and is massive (M org) to planar laminated (M lam org). Carbonaceous mudstone lithofacies appears over rooted muddy sandstone and presents plant debris and coal laminae. (Figures 2g, 3d). Carbonaceous mudstone lithofacies are mostly 0.01 to 0.2 m thick in the western side of the Llanos Basin, and up to 6 m thick in some wells of the eastern sector of the Llanos Basin. The carbonaceous mudstone is bioturbated with planolites (Pl), and Glossyfungites (Gl) when situated below sandstones. Carbonaceous mudstone (M org, M lam org) salinity index in Well-7 ranges from 0.043 to 0.059, indicating brakish conditions of marshes, lagoons, or swamps.
The mudstone is a vertical accretion deposit under ponded water with a high contribution of vegetal carbonaceous material. The ichnofossils contained in this mudstone are of low diversity and density. These could indicate restricted settings, such as coastal plains, swamps, marshes, or coastal lagoon environments (brackish waters) [54]. In these environments, poorly drained areas, and even in the floodplain, could conduct to reducing conditions to preserve the organic matter [55].
F8: DARK-COLORED MUDSTONE (M lam)
This facies consists of dark gray to greenish-gray laminated mudstone (M lam) with isolated lenses or laminae of silt and very fine-grained sandstone. The dark-colored mudstones are locally bioturbated by marine organisms, with bioturbation index 2 (0-10%). Dark-colored mudstone is the most abundant in the Eocene - early Oligocene rocks of the Llanos Basin and it composes most of the C8 member of the Carbonera Fm (Figure 3b). Its thickness varies from a few cms to tens of meters in the SLLB.
This lithofacies was deposited in a subaqueous environment by vertical settling of mud below fair-weather wave base. When very thin laminae of silt or very fine-grained sandstones are interlayered, it means that there were interruptions by slightly more energetic events such as storms that reach these depths [51],[52].
Dark-colored mudstone (M lam) has a marine origin; in the Sagú Creek and well-1, the salinity index is from 0.11 to 0.81 and 0.220.41, respectively. To the east in well-3, this SI is between 0.48 to 0.65; in well-5, the SI value is between 0.0143 to 0.112; in well-7 SI is between 0.015 and 0.43, and in the easternmost wells, the values of SI range between 0.074 and 0.48. These values indicate a marine depositional environment,
F9: BIOTURBATED HETEROLITHIC LITHOFACIES (S/M lam biot)
Heterolithic lithofacies consists of sandstone interlayered with gray laminated mudstone (S/M lam biot). These facies evidence bioturbation. The sandstone is fine to very fine, very well sorted, and has wave ripple lamination (Figure 3c). The heterolithic facies shows horizontal and vertical burrows of Ophiomorpha, Skolithos, Teichichnus, Diplocraterion, Macaronichnus, and Cilindrichnus. Its bioturbation index is variable, with BI = 2: sparse bioturbation (Figure 3c left outcrop), to BI = 4: high bioturbation (Figure 3c right core). Bioturbation processes mixed both lithologies and make it difficult to see the primary physical structures. Heterolithic lithofacies are overlain by bioturbated cross-bedded sandstone. The thickness of heterolithic lithofacies ranges between 0.5 and 5 meters.
The wave lamination is evidence of weak oscillating currents at the limit of the fair-weather wave base. Bioturbation indicates low sedimentation rates, low energy in the environment, and reworking of the sediment. The heterolithic lithofacies are associated with shallow-marine environments, in the lower shoreface [51].
F10: Coal
Dark gray to black coal lithofacies appears shiny or dull in cores, with local pyrite nodules. Coal seams are recorded over rooted muddy sandstone, or between laminated organic mudstone (Figures 2g, 3d). Coal seams are thicker when located at the base or within organic laminated mudstones (Figure 6, FS3 at the bottom).
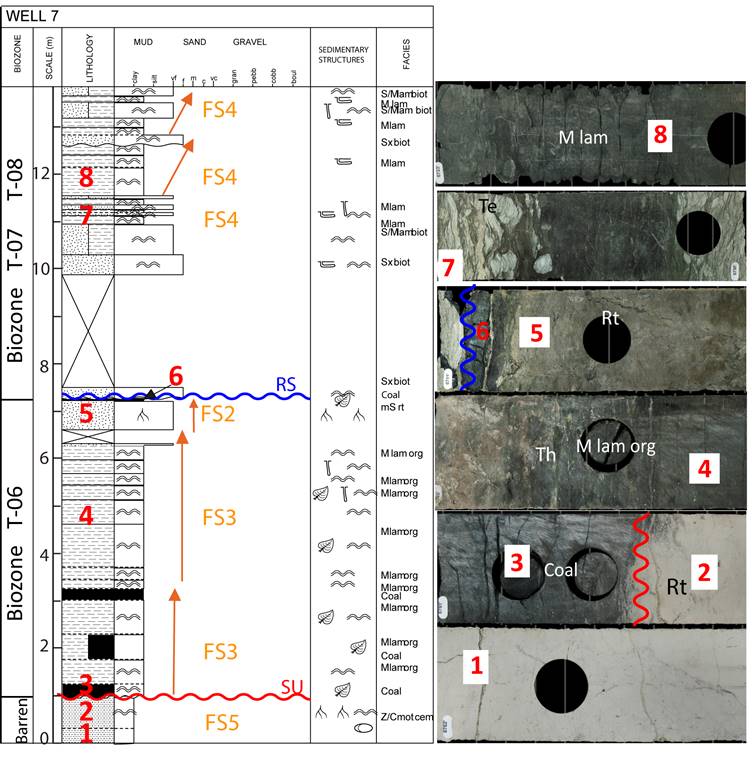
Figure 6 Facies successions in well 7. At bottom, the extensively-weathered paleosol (FS5), and the unconformity above. Overlying the unconformity, two facies successions of coal and carbonaceous mudstone (FS3) overlayed by a coastal plain compound paleosol (FS2). The ravinement surface separates this coastal plain deposit from the marine deposits above. There are several high frequency, upward coarsening marine facies successions (FS4) overlying the ravinement surface. Teichichnus (Te) Thallasinoides (Th) and Root traces (Rt).
Salinity index for coal SI = 0 to SI = 0,0059, indicate brakish conditions where marine waters flood freshwater environments. These conditions are the same as the facies of dark-colored mudstone (M lam), and these facies are associated.
The coal rocks form in swamps or mires, either in coastal plains or in flood plains. They register drowning of the coastal or flood plain due to relative sea-level rise and correspondent high-water table. These swamps develop vegetation ecosystems that supply high quantities of organic matter. Once the vegetal materials die and accumulate on the bottom water, it depletes dissolved oxygen and promotes reducing conditions that preserve organic matter to produce coal.
FACIES SUCCESSION 1 (FS1): FLUVIAL CHANNEL AND BAR SANDSTONES
This facies succession one is composed of previously described facies, which are F1: sandy conglomerate, F2: sandstone, and F5: light-colored mudstone.
FS1 starts with sandy pebble conglomerate lithofacies. These facies are followed, in sharp or gradational contact, by cross-bedded pebbly coarse sandstone or cross-bedded medium to coarse sandstone (Figure 4, 5). Thin beds of white to greenish-gray silty mudstone (cM lam) sometimes interrupt the sandstone and even the conglomerate sets. In some of the wells, this facies succession is covered at the top by a thick set of a plane or tangential cross-bedded sandstone, with mud drapes and rhythmic foreset thickness (S x rh in Figure 2d).
The FS1 is typical of the lower Mirador Formation in the Foothills of the Llanos basin (Figures 4, 5). The FS1 amalgamates, with the individual successions being upward finning. Although these successions pile up, in GR well-logs the pattern is box-like (Figure 8), typical of a braided fluvial system.
In the Foothills and WSLLB, the FS1 facies successions thicken upwards and become sandier upward (Figures 4, 5); they also preserve physical structures like plane-cross to tangential cross-stratification with mud drapes above foresets. This stacking pattern and fabrics could be a sign of tidal influence but also an increase in accommodation space (Figure 7a, b). This facies succession is interpreted as a braided channel belt composed of channels and bar sandstones several kms wide [56], [57] (Figures 7a, d).
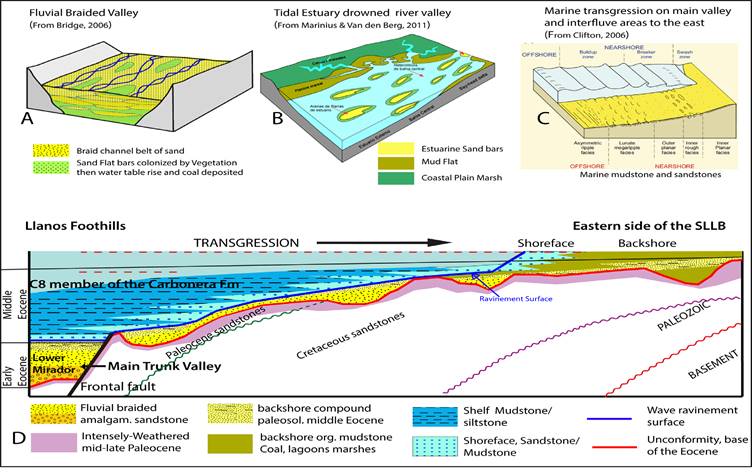
Figure 7 Cartoons are illustrating the change in depositional environment from the lower Mirador Formation to the C8 member of the Carbonera Formation in SLLB. (a): Ideal cartoon of a high energy braided river valley, this could be the kind of environment where the basal Eocene facies deposited. (b): Tidal estuary is a river valley drowned by the sea on transgression; this is the case in the Cusiana and Cupiagua upper Mirador member. (c): Example of a low energy shallow marine environment of the C8 member. (d). Ideal sketch of a section in the studied area at the end of the middle Eocene. Lower Mirador braided facies rapidly were drowned by the sea; without estuarine facies developed, maybe it was a braid plain in an intrabasinal piedmont with paleohighs to the east. The transgression fossilizes a low relief that was previously occupied a fluvial braided-channel. To the east, some of the fluvial landscape is filled by coastal plain facies.
FACIES SUCCESSION 2 (FS2): COASTAL PLAIN COMPOUND PALEOSOL
The facies succession 2 is composed of facies previously described, which are F3: rooted sandstone, F7: carbonaceous mudstone and F10: Coal.
This compound paleosol overlies the FS1, and consists of rooted muddy sandstone (mS rt) which thickness ranges between 4 m to 40 cm thick (Figures 2f, 2g, 4, 5, 6) and is covered in most wells by a thin layer of coal or carbonaceous mudstone (M lam org) of 5 to 15 cm thick. The compound paleosol succession (FS2) could suggest a change from well-drained conditions under a subaerial vegetation cover to poor-drained conditions of marsh and swamps, which halt vegetation growth, which is incorporated as coal or coaly mudstone.
FACIES SUCCESSION 3 (FS3): COASTAL PLAIN SWAMP- MARSH DEPOSITS
The facies succession 3 is composed of facies previously described: F7: carbonaceous mudstone deposited on brakish conditions and F10 coal.
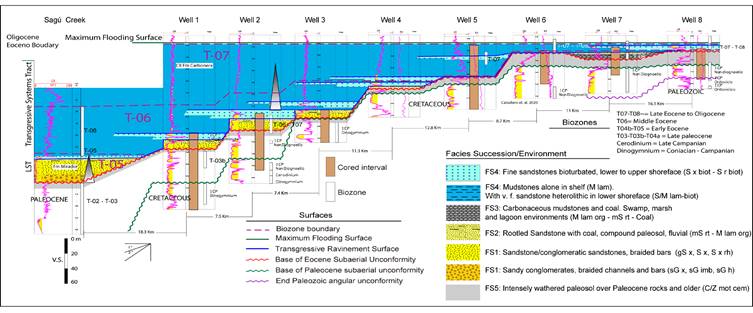
Figure 8 Correlation of outcrop section in the Foothills and wells in the SLLB. It shows the facies successions and stratigraphic surfaces detailed in this study along the area. At the bottom of the studied interval, the correlation shows the intensely-weathered paleosol of late Paleocene overlayed unconformable by the fluvial braided facies succession FS1. The FS1 is, in turn, overlaid by the compound paleosol dated as middle Eocene (Lutetian) and a layer of coal or carbonaceous mudstone (FS1-FS2-FS3) of the lower Mirador Formation. These rocks were deposited in the earliest-middle Eocene under low accommodation conditions. The wave ravinement surface erodes the deposits below and marks the initial transgression. The marine facies successions deposit above the ravinement surface. The deposits of carbonaceous mudstone and coal to the east in well 7 are correlatable with the marine facies to the west.
These backshore marsh, lagoon, or swamp deposits (FS3) were identified mainly in the eastern part of the Llanos basin, where it is Middle Eocene in age (Figure 6). The carbonaceous mudstone requires a low energy environment, with a nearby source of organic matter (thickness up to 6 m). These conditions exist in a backshore, or coastal plain setting where vegetation grows abundantly. It could be a marsh or shallow swamp or lagoon environment [54].
Poorly drained conditions, even in the floodplain, could conduct to reducing conditions that preserve organic matter [55]. This facies succession in the SLLB indicates a rise in relative sea level, which floods interfluvial areas (Figure 7b, d) toward the eastern side of the southern Llanos Basin and allowed swamps, marshes to thrive and finally fill up with coal and dark gray to black mudstone.
FACIES SUCCESSION 4 (FS4): LOW ENERGY SHALLOW MARINE
The facies succession FS4 consists of previously described facies: F8: laminated mudstone, F9: heterolithic lithofacies, and F4: bioturbated sandstone.
The FS4 at the bottom contains laminated mudstones (M lam) that gradually increases in very fine sand laminae and become heterolithic and bioturbated. The above facies are overlain by wave rippled fine to very fine well-sorted bioturbated sandstone. The above successions are capped by a bioturbated cross-bedded medium sandstone (Figures 4 and 5). Each cycle of FS4 on the western side of the southern Llanos basin could reach 28 m thick; in the eastern part of the Llanos Basin, it is no more than 14 m thick.
The FS4 configures intercalations of laminated mudstone, and bioturbated wave rippled sandstones in erosive contact (Figure 3c). Facies succession 4 is repeated upwards in well-cores (Figure 6). The upward trend of FS4 corresponds to an upward coarsening succession deposited on a shallow water low-energy shelf and its upper shoreface [52].
The facies succession FS4 lay above the transgressive ravinement surface, i.e. the Wave-Ravinement Surface (Figure 7c, d). This surface of marine erosion is a scoured surface cut by waves during the shoreline transgression. It is onlapped by the shallow marine, lower shoreface to shelf facies of the Facies Succession Four (FS4)
FACIES SUCCESSION FS5: INTENSELY-WEATHERED PALEOSOL
The facies succession 5 corresponds to the facies F6 ,which is composed of white to greenish-gray or cream, mottled, and cemented silty claystone (Z/C mot cem). The paleosol is 1 to 6 m thick and more mature towards the eastern sector of the basin. Stratigraphically, it corresponds to the top of the Cuervos Formation, but this paleosol has been developed above any other previous lithology like the Cretaceous (wells 3 to 8 in Figure 8) or Paleozoic rocks towards the easternmost southern Llanos basin (not shown but seen in wells). This paleosol was formed during a period of landscape degradation and stability and is likely an interfluve paleosol that marks the sequence boundary.
The FS5 formed under extreme climatic conditions, and it probably corresponds to the Paleocene Eocene boundary [58]. It developed over different lithological units around the southern sector of the Llanos Basin.
AGE AND PROVENANCE OF THE PALEOSOLS
We report new detrital zircon U-Pb ages for two samples of intensely-weathered paleosol from the Sagú creek and Well-5, and one from the compound paleosol in Well-2 (Figure 9). Detrital ages achieved for the first paleosol in the Sagu creek and Well-5 gave late Paleocene ages. Detrital zircon ages for the compound paleosol in Well-2 gave middle Eocene ages.
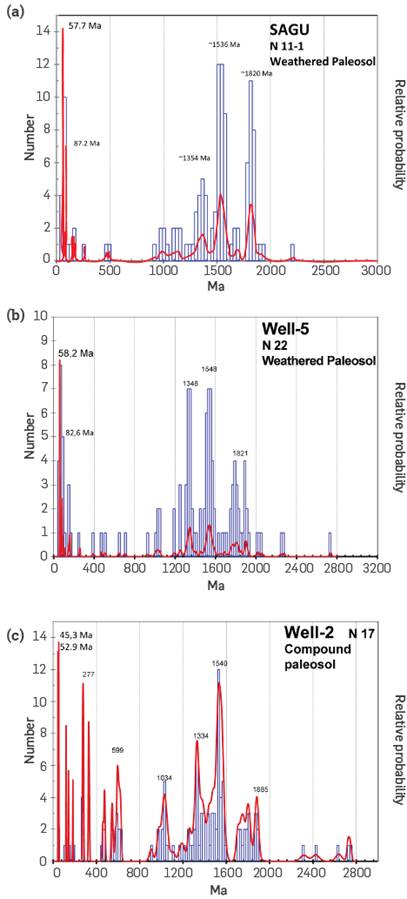
Figure 9 Detrital zircon age distribution for two paleosols in the SLLB. (a) and (b): intensely-weathered paleosol in the Sagu creek that shows younger peaks of 57.7 and 58.2 Ma indicating a late Paleocene age for this paleosol. (c): the sandstones with root casts of the compound paleosol sandstone - compound paleosol in well-2 shows two detrital ages, which indicates this paleosol is middle Eocene. See text for provenance analysis.
Eight detrital zircon U-Pb ages for the intensely-weathered paleosol in the Sagu creek ranged between 60.1±1.2 to 57.1±1.1 Ma, and the youngest peak was 57.7 Ma. These ages suggest that the paleosol is late Paleocene. Another sample from well-5 showed ten ages that range between 61.2±1.2 to 57.7±1.4 Ma and the youngest peak 58.2 Ma (Figure 9a, 9b), indicating that this paleosol is also late Paleocene (Thanetian).
For the compound paleosol, we analyzed several samples obtained from wells, but gathered no more than one grain of Cenozoic age. In at least five of the wells, dated samples showed one grain and a range of ages for the rooted muddy sandstones between 44 to 47 Ma. One sample of the well-2 showed two grains with ages 45.3±0.9 Ma and 47.8±0.7 Ma that indicates this paleosol is middle Eocene (Lutetian) in the SLLB (Figure 9c).
We propose the age of the thin braided carpet of sandstones of the Lower Mirador Formation located in between the two paleosols. These fluvial braided facies deposited during the earliest-middle Eocene (Lutetian). That age corroborates the zonation obtained with palynology for this unit in the SLLB (Figure 8).
In the detrital zircon age distribution for the paleosols, besides the f- Cenozoic age group <200 Ma, the Sagu paleosol shows several other peaks between 900-1200,1300-1600, and >1700 Ma. The well-5 paleosol has the same Cenozoic age group <200 Ma and age peaks 1300-1600 and >1700 Ma with Grenvillean peak absent.
In the Paleocene paleosols, the Cenozoic peak ages are commonly interpreted as originating in the Central Cordillera, where a magmatic arc was active at the time. In contrast, Grenvillean peaks or older originate in the Guyana Craton, but, also, these could be recycled grains from the early Cretaceous sandstone rocks eroded during the late Cretaceous - Paleocene orogenic phase, from above the Craton.
The paleosol in well-2 has Cenozoic age group <200 Ma, a peak at 250-300 Ma, another one between 450-600 Ma, the same 900-1200, 1300-1600 Ma, and >1700 Ma. The peaks older than Grenvillean age in the middle Eocene paleosol are likely to originate from the erosion of Cretaceous rocks in the Craton or the Craton itself. However, the two other peaks of Paleozoic and Mesozoic age, probably their provenance is the basement or they are recycled from Mesozoic rocks in the Eastern Cordillera. See Horton [59] for a provenance analysis and potential zircon source areas for the NW of south America.
5. RESULTS ANALYSIS
The facies successions described above are distributed in an orderly manner in outcrops of the Foothills and subsurface wells (Figure 8).
Lower Mirador fluvial braided facies overlay unconformably an intensely-weathered paleosol of middle to late Paleocene. Coincidently, these fluvial conglomerates and sandstones are also overlaid by another paleosol, a compound paleosol of the latest middle Eocene. The latter paleosol indicates cessation of the system and stabilization of the fluvial fill that was colonized by vegetation. The vegetation was later preserved as a coal layer or coaly mudstone, due to drowning. This deposit indicates the start of a marine flooding in this part of the basin, but it is poorly preserved as in the Sagu Creek where it was eroded (Figure 2f, 4).
In well-7, continental facies are muddy, coaly, deposited in a swamp, marsh, and lagoon environment and capped by a compound paleosol. These facies are equivalent to the beginning of the flooding to the east during middle Eocene. The continental facies successions previously mentioned are overlain by the low energy shallow marine facies succession four FS4. The contact between these two corresponds to an erosion surface, the wave ravinement surface (Figures 4, 5, 6, and 8). In wells 5, 6, and 8, there are no continental facies deposited. In these wells, the ravinement surface reworks the base of the Eocene unconformity (Figures 7d, 8). This means the deposits in well-7 were protected from the wave action such as in an embayment, whereas other like in wells 5, 6, and 8 were exposed to the wave action [60].
BASIN CONFIGURATION
The distribution of continental facies indicates there was a relief in the SLLB, just at the onset of deposition of the Mirador Formation. According to the continental facies thickness and the position of the intensely-weathered and compound paleosol, the relief may be less than 50 m based on thickness of the deposit. The hills are composed mainly of Cretaceous and Paleozoic sedimentary rocks (Figure 7d), or basement crystalline rocks to the eastern sector. In the east area of the SLLB, the basement highs like El Melón and El Viento developed an intricate topography. Similar relief was identified previously by seismic images in a 3D seismic Cube in the SLLB [18],[61].
ONSET AGE OF TRANSGRESSION
According to palynological zones in the correlation (Figure 8), and U-Pb detrital zircon ages, by early Eocene, deposition of fluvial facies was taking place only in the fluvial valley located along the Foothills (trunk valley). The marine transgression began at the end of early Eocene in the trunk valley, after the deposition of the compound paleosol, and the sedimentary system had to retrograde.
During middle Eocene, several tributary braided channels valleys to the main foothill trunk were filled by fluvial facies as the relative sea level rises and advances to the east making accommodation space available. Each tributary deposit to the east is younger than the previous one, and each one was halted as the marine transgression developed rapidly in a west-east direction. To the east, the middle Eocene flooding generated a coastal plain with embayments where carbonaceous mudstones and coal deposited until the end of middle Eocene, then by the late Eocene the marine shoreface established above them.
COMPARISON BETWEEN EOCENE FACIES OF THE SLLB AND THE CUSIANA FIELD
In both areas, fluvial channel and bar sandstones are reported for the lower Mirador; however, in Cusiana and Cupiagua, they are deposited in incised valleys and contain floodplain mudstones and crevasse splay sandstones, which is a sign of a low energy meandric style [2],[12],[3]. In the SLLB, these facies are amalgamated channel and bar sandstones and conglomerates without floodplain facies that indicates they were deposited in a piedmont to the intrabasinal highs to the east in high energy fluvial braided channel valley belts between hills less than 50 m high. Thickness is prominent in the north; as we have seen in well-1 and the Sagú creek, the thickness of the lower Mirador Formation is 22 and 33 m, respectively. In the Cusiana Field, this unit is 50 to 70 m thick.
Overlying the lower Mirador Formation, the shallow marine mudstones and bioturbated sandstones are less than 10 m thick in SLLB and the southern Foothills. These lithologies are more correlatable to the C8 member of the Carbonera Formation. This evidence indicates that the upper Mirador is absent in the SLLB. In Cusiana and Cupiagua, the upper Mirador is mainly sandstones and mudstones deposited on marginal marine environments, mostly estuaries, accounting for almost half of the thickness of all the Mirador Formation there (i. e. nearly 60-70 m) [2],[12],[3]. In the SLLB and Foothills, the fluvial facies successions of the lower Mirador member are separated from the marine facies successions by a ravinement surface. The wave ravinement surface is located above the middle Eocene (45.3 Ma) compound paleosol developed over the top of the continental fluvial facies successions. In the Cusiana field, a similar boundary was identified previously; this is an unconformity of at least ten million years duration. The unconformity represents a dominant control in the compartmentalization of the Mirador Formation due to its erosive character and facies changes throughout.
This sea-level rise probably was driven by tectonics during the middle-late Eocene. At this age, tectonic deformation, and loading advanced to the Eastern Cordillera [30],[26],[31],[32],[33]. The tectonic load generated by this mechanism led to the flexural subsidence and relative sea-level rise that allowed the marine flooding of the basin.
REGIONAL SEDIMENTARY ENVIRONMENTS CONFIGURATION
The configuration of the sedimentary environment for the Mirador Fm in the SLLB and Foothills corresponds to a proximal fluvial system that consists of a several km wide and only some tens meter thick braid belt, crossing a low relief less than 50 m high, like a braided piedmont. This system connects to a trunk valley where an estuarine system established northward, including the Medina depocenter and also the Nunchia, Zamaricote, and Cubara Synclines. In this regional configuration the deposits are not continuous but located along channel belts in a general northwestern direction between hills less than 50 m high. The thicker deposits are in the Foothills and Chichimene area whereas these pinch out out to the east. The marine deposits would be perpendicular tho the above fluvial deposits.
CONCLUSIONS
Previous studies and new data presented in this paper indicate that at the end of Paleocene, this area was exposed as the result of a relative sea-level fall driven by tectonics [29], [18], [62]. In the Llanos Basin, this relative sea-level fall reduced the accommodation space and established a fluvial relief with a fluvial valley parallel to the Eastern Cordillera Foothills toward the north. In the SLLB, Several piedmont tributary braided valleys connected to the central trunk valley.
The trunk valley was filled with amalgamated gravel and sand during early Eocene in the Foothills and middle Eocene in the tributary braid plain to the east. As relative sea level was rising and advancing in that direction, these tributaries were flooded and occupied by backshore mire, swamp, and lagoon systems configuring a swath of environments from coastal plain to marine from east to west. Another part of the higher interfluve area, the hills, continued in a subaerial exposition of the substrate, and development of paleosol.
By late Eocene, the transgression reached the eastern sector and covered all the previous landscape with shallow marine sandstones and mudstones of shoreface to shelf environment. Finally, at the limit between Eocene and Oligocene, the entire basin was covered and flooded with shallow marine shales (Figure 8).
Additional observations emerge from this facies analysis. The unconformity at the base of the Eocene is a diachronic surface as at the Foothills it puts into contact pedogenized rocks of the Paleocene with sandstones of the middle Eocene and, finally, in the eastern side of SLLB, it puts pedogenized Cretaceous rocks in contact with middle or late Eocene rocks.
The ravinement surface is a diachronic surface, it is younger to the east, it reworks lower Eocene deposits in the Foothills while in the western SLLB it reworks middle Eocene deposits. The magnitude of the erosion produced by this surface is yet to be determined.
We consider that this surface does not represent an unconformity as was proposed in the Cusiana area. We have dated the paleosols at the bottom of the lower Mirador as late Paleocene (Thanetian), and the paleosol at the top as middle Eocene (Lutetian), and then the lower Mirador in SLLB is earliest-middle Eocene (Lutetian). In the Cusiana area, the proposed unconformity duration is 10 Ma during the middle Eocene; this unconformity is not present in the SLLB, because above the ravinemente surface, the marine deposits are dated as middle to late Eocene.
The marine facies fossilize a previous landscape created in late Paleocene time in the SLLB. The late Eocene -Oligocene boundary, between biozone T-07 to T-08, coincides with a Maximum Flooding Surface (Figure 8).
The Mirador Formation reservoir rocks are not continuous but distributed along channel belts composed of channels and braided bars in an NW direction between hills less than 50 m high. The marine shoreface reservoirs are distributed as swaths of sandstones in a perpendicular direction to the fluvial belts. This reservoir has excellent seal rocks above.
Future exploration in the Southern Llanos Basin will require the acquisition and interpretation of 3D seismic as a routine tool if it is proved economically viable to map the fluvial braided belts and the buried hills in the SLLB as a valid play.