1. INTRODUCTION
Implementing oil recovery technologies is essential to increase the recovery factor and reserves in Colombian oil fields. To sustain oil production and expand the company's reserves, Ecopetrol S.A is evaluating water injection processes and chemical enhanced oil recovery (cEOR) with Colloidal Dispersion Gels (CDG), polymers, and surfactants, which were studied at the laboratory level, numerical simulation, and validated on a pilot scale [1]-[4]. However, the current hydrocarbon industry market's challenges demand the search for new products to improve the water injection processes performance at a lower cost and with less capital investment for their large-scale implementation.
To mobilize the trapped oil in the reservoir, a favorable change is required in the fluid displacement effective mobility ratio and/or the significant reduction of the interfacial tension between the residual oil and the displacing fluid. Hence, the injection of individual and/or combined polymers and surfactants have been potentially efficient recovery methods [5]-[7]. Different injection strategies could be considered to obtain the best performance of chemical flooding adapted to reservoir conditions. Indeed, depending on the rock's mineralogy and the respective adsorption levels of polymer and surfactant, the polymer front is often injected behind the surfactant front. As such, the polymer of the SP slug plays its role in controlling the mobility and improve the sweep to maximize the contact of the surfactant with the oil-bearing zones. In contrast, the post flush polymer drives the oil bank created by the surfactant. Nevertheless, due to different operating conditions, conventional surfactant and polymer blends tend to separate in a flowing stream through a porous medium, possibly affecting the SP slug efficiency. Further problems can be attributed to the incompatibility between the surfactants and the polymers in the formation, which results in a decrease in product performance because of problems associated with the aggregation, adsorption, and diffusion in porous media.
The injection of polymeric surfactants could be a unique chemical approach to Enhanced Oil Recovery (EOR) as favorable microscopic and macroscopic displacement efficiencies are provided simultaneously. In this regard, the reduction of the interfacial energy induced by the presence of surfactant, as well as the non-Newtonian behavior of the aqueous formulation injected, are available avoiding, at the same time, compatibility or tuning problems.
The amphiphilic polymer's potential for EOR has been recognized, mainly due to their ability to form a transitional association, causing viscosity increase under reservoir salinity, temperature, and flow conditions. Surprisingly, little attention has been paid to emulsification phenomena and such polymers surface properties, which are also crucial parameters in EOR processes.
The structure and composition of polymeric surfactants are essential to determine the surface activity and the rheological behavior in aqueous solutions. Furthermore, the physical properties can be significantly affected by temperature, salts content, and flow velocity.
Choosing a suitable system based on the above considerations is not trivial. For instance, various polymeric surfactants show very low or even nil surface activity. Some authors explain this lack of Interfacial activity by associating it with a very slow equilibrium that prevents the possibility of macromolecules migrating to interfaces. Nevertheless, it has been observed that polymeric surfactants can significantly reduce interfacial tension [8].
Aqueous solutions of certain polymeric surfactants show a considerable increase in viscosity with increasing salinity [9] and/ or temperature up to a point where a maximum is reached. The location of these maximum points is expected to be a function of concentration and molecular structure. The existence of these high-performance points is highly positive, particularly when compared to commercial hydrolyzed polyacrylamides (HPAM), which are products that undergo degradation by mechanical stress, thermal or chemical reactions and, therefore, loss of performance in the EOR process.
This study focuses on determining the experimental/operational feasibility of using polymeric surfactants in EOR processes through a conceptual design for a Colombian field. Rheological and phase behavior studies in brine/crude/polymeric-surfactant systems showed that the appropriate product choice is critical in reaching favorable rheological and interfacial tension conditions to displace residual oil in the porous medium. Optimal operating conditions and performance parameters were obtained through numerical reservoir simulation and process engineering of technology application.
2. THEORETICAL FRAMEWORK
The waterflooding performance decreases in applications where the porous medium has high heterogeneity and high crude oil viscosity. It is estimated that after the first two extraction stages (conventional oil recovery), only about one third of the OOIP is produced, with about two-thirds of the OOIP remaining to be recovered [10], [11]. The third stage, known as Enhanced Oil Recovery (EOR), is perhaps the most demanding in terms of recovery; this is because the oil is trapped by capillary forces or diverted somehow during extraction [12], thus hindering their recovery. The chemical EOR processes (cEOR) have gained wide acceptance due to high efficiency in oil displacement, both macro (crude oil total displacement) and microscopically (oil displacement trapped in the pores), as added to its technical and economic feasibility at a reasonable capital cost [12]. The cEOR process is characterized by the use of foams [13], nanotechnology [14], surfactants (S) [15], polymers (P) [16], alkalis (A) [17] or the combination of these technologies, such as AS [18], AP [19], ASP [20] or SP [9].
The use of surfactants seeks to reduce the interfacial tension and modify rock wettability [21], [22]. Meanwhile, polymers increase their viscosity and reduce the aqueous phase mobility, improving sweep efficiency and recovery factor [23]. Partially hydrolyzed polyacrylamide (HPAM) is the most widely used polymer in the EOR industry due to its cost/benefit ratio. It is used as reference in the development and evaluation of new formulas [24]-[27].
Polymeric surfactants have molecular structures that promote surfactant and viscosifying properties that favor oil mobility and the oil recovery factor given the increase in the capillary number of the system [28]-[31]. On the other hand, without the mechanical or thermal-chemical stability problems presented by HPAM type polymers [32], [33], or well-known high adsorption phenomena and tuning loss in conventional surfactant formulas [34], [35], mostly, when the injection process was not optimized.
3. STATE OF THE TECHNIQUE
The cEOR methods became widely known in the 1980s due to the high oil price and technological progress [12], and since then, significant technical contributions have been developed. Its proper application depends on the properties of both, reservoir fluid and reservoir rock [10]. Regarding the use of polymeric surfactants, a brief description of the most recent advances is included.
Rai et al. [30] studied the significance of eighteen dimensionless groups that control oil recovery in surfactant polymer injection processes. The variables identified with the highest impact on oil recovery include the initial oil saturation, the ratio of the endpoint relative permeability of phase l at the high and low capillary number k rl o,H / k rl o.L , and reservoir heterogeneity groups assuming adequate mass of chemicals are injected at favorable salinity gradient.
Xu et al. [36] studied the microscopic mechanism of oil displacement by polymeric surfactant direct injection and by flooding with micro-emulsion (polymeric surfactant + oil) followed by a polymeric surfactant. They found that polymeric surfactant injection can increase the aqueous phase viscosity, reduce the water-oil mobility ratio, and expand the swept area. Furthermore, the micro-emulsion can sweep areas with dead pores that cannot be swept with water or polymeric surfactant only.
Co et al. [37] proved than ultralow IFT values are not strictly necessary to improve oil recovery, Which seems to be also supported by a very recent study dealing with a polymeric surfactant rather than an SP mixture. In this case, the surface-active modified HPAM solution with a measured IFT of 10-1 mN/m gets 5% more oil recovery than a conventional HPAM in a core-flood experiment, even with lower viscosity.
Wu et al. [38] reported the synthesis PFSA (fluorine-containing polymeric surfactant), polymeric surfactant with potential use in EOR. In terms of displacement and oil sweep efficiency and, according to its physicochemical properties (critical micellar concentration or CMC), in 0.1 g/L aqueous solution with 100 nm micellar diameter can reduce the interfacial tension down to around 0.027 mN/m, even at high NaCl concentrations and high temperature.
In recent years, the Instituto Colombiano de Petróleo (ICP) has been studying ethylene oxides (EO) and propylene (PO) polymeric surfactants for application in cEOR projects. Results show both the interfacial tension reduction and the increase in viscosity in representative Colombian reservoir conditions.
4. EXPERIMENTAL DEVELOPMENT
Commercial Block Copolymer Surfactants (BCPS) EO-PO-EO with low molecular weight (945-6500 g/mol) were evaluated in this study. The organization of monomer blocks in the molecules being assessed is shown in Figure 1.
For the fluid-fluid and fluid-rock feasibility tests, 25° API ntermediate crude with 60 cP viscosity at 22°C was selected (dead oil). Synthetic brines between 0.2 and 3.2 wt% of total dissolved solids (TDS) using 6.2:1 NaCl to CaCl2 ratio according to the representative composition of field case production brines used for the different tests in this study. Commercial demulsifying and clarifying additives were also used to validate the emulsions behavior under representative operational conditions of the field case.
METHODS AND EQUIPMENT
The experimental evaluation was carried out using new in-house protocols developed at the ICP, some of them based on recommended practices to determine the feasibility of using polymers and/or surfactants in EOR processes [39]-[41].
The first step of the followed protocol includes a basic characterization and evaluation of different properties. Polymeric surfactant viscosity as a function of the shear rate and performance for different BCPS types at different concentrations and salinities are among the main properties determined. This step aims to screen products with better performance properties in the field case conditions, as shown in Figure 2.
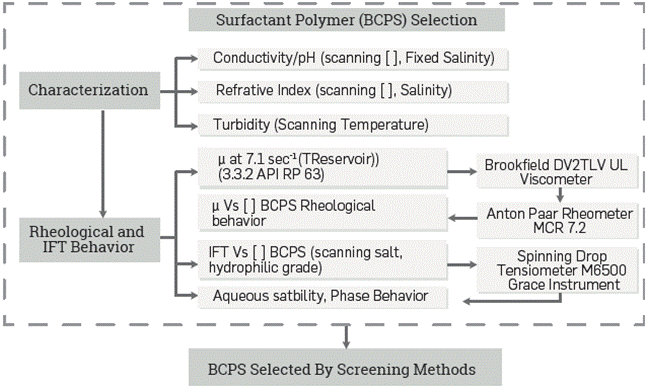
Figure 2 Laboratory equipment and Test conditions used for basic characterization and rheological/IFT behavior of surfactant polymers.
In a second stage, emulsion stability tests were carried out to confirm the fluid compatibility with the selected BCPS in different residual concentrations at reservoir and surface treatment scenarios, following in-house protocols. Experimental designs based on variables such as residual BCPS concentration (20 - 40 wt% of injection concentration), water/oil ratio WOR (30/70, 50/50, 70/30) at the wellhead stage, and surface treatment scenarios were performed. Lower BCPS residual concentrations (5 -10 wt% of the initial concentration injected) were projected to simulate the dilution process of production fluids before entering to the treatment facilities, keeping the crude/water mix ratio and the dehydration process temperature (65 °C).
Mechanical (MD) and Thermal-Chemical (TCD) degradation were assessed according to in-house protocols (Figure 3). In the MD test, the solution is placed in a stainless steel cylinder and pressurized with nitrogen. Then, it is forced to flow through a 1/8" capillary by opening a valve in between. TCD degradation test is a viscosity and IFT sequential monitoring when the BCPS solution is exposed for a Long term at reservoir conditions (temperature and salinity),
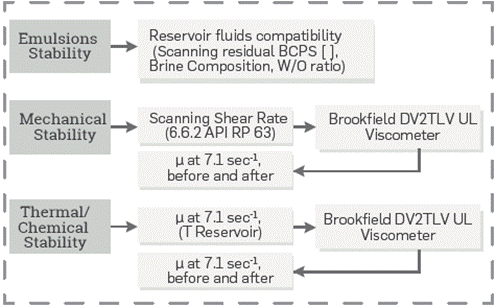
Figure 3 Laboratory equipment and Test conditions used for mechanical/thermal/chemical stability of surfactant polymers and emulsions behavior.
Finally, rock-fluid interaction tests were performed. According to the field case studied, adsorption isotherms were measured by placing one gram of sand in a container, adding 10 ml_ of BCPS solution in 0.8wt% TDS brines. After 24 hours at 60°C, the sample is centrifuged, and the supernatant is extracted to determine the equilibrium concentration for which the nephelometric method by reaction with tannic acid is used [42]. Contact angles were measured at room temperature in a goniometer and optical tensiometer (OCA 25 PMC 750), using dead oil and BCPS (0.2 wt%) prepared in a brine solution (0.8 wt%).
Displacement tests were run in sand packs (Ottawa 80-110 mesh and Kaolinite, 98:2 ratio). The sand packs were contained in PVC pipes of 1" diameter and 6.5" length. Two packages of similar dimensions were used in each test, aligned in parallel, one with high permeability, made up of Ottawa 80-100 mesh reference sand. The second one with Less permeability, using a 98:2 mix ratio of 80-100 mesh Ottawa sand and kaolin The aim is to create a permeability contrast, to evaluate the performance of polymeric surfactants in terms of flow distribution in an heterogeneous porous medium
Three displacement efficiency tests were carried out to compare both BCPS and HPAM at 0.2 wt% in 0.8 wt% TDS synthetic brines performance against a waterflooding baseline, and with each other The purpose was to conduct a waterflooding step in both tests roughly until water breakthrough before injecting the chemicals. Figure 4 shows a flow chart of the injection sequence in the three scenarios.
The BCPS solution was injected at a constant flow rate (0.067 ml_/ min) in sand packing brine saturated SW=1 to measure the dynamic adsorption on rock following standard procedures [40], [42], [43] For the evaluation of flow parameters of BCPS solutions (0.2 wt%) such as reduced mobility curves (resistance factor/RF) and residual resistance factors (RRF), various pore volumes of BCPS solutions were injected at different flow rates (0.067; 0.167; 0.25 mL/mi) and concentrations (500; 1,000; 2,000 ppm) in a Berea sandstone saturated with 0.8 wt % TDS brine following standard procedures [40],[42],[43].
The estimated parameters are the input data into the numerical simulation that contributed to predicting the BCPS slug performance in a conceptual reservoir model.
NUMERICAL SIMULATION AND CONCEPTUAL DESIGN OF THE PROCESS
The numerical simulation supports the design of the recovery processes. In the case of the injection of polymeric surfactants, it helps define the optimum pore volume of chemicals to be injected the flow rate and slug concentration, and the process operating conditions that allow estimating the injection facilities capacity and the incremental production expected.
The numericalsimulation was performed using a conceptual model built-in commercial software (CMG STARS®). The propagation of petrophysical properties in the model was based on a geostatistical analysis representing the reservoir heterogeneities under evaluation. Six parameters commonly represent the typical behavior of a polymeric surfactant injection process: dynamic adsorption retention, or entrapment of the BCPS molecules, inaccessible pore volume (IPV), rheological behavior/mobility control (RF), and interfacial tension (IFT) depending on its concentration, permeability reduction (RRF), and changes in oil saturation (Sor). Each of them was included in the numerical modeling
Different scenarios were simulated, varying operational parameters to determine the best BCPS solution injection strategy. The injection process design is based on a simple operation scheme as a polymer hydration unit such as that used for HPAM type polymers is not required. Due to its characteristics, the BCPS could be dosed directly in the water injection pipeline to the well, using a high precision dosing pump with high flow and pressure operating variables. Then it should be homogenized with a static mixer in the pipeline before entering the reservoir.
5. RESULTS ANALYSIS
BASIC PROPERTIES CHARACTERIZATION
The turbidity of aqueous surfactant solutions represents the micelles contained quantity and size. The latter is directly correlated with the solubilizing capacity, rheological behavior, adsorption tendency, etc. According to the results, the BCPS-containing brines turbidity depends on the salinity, the surfactant's concentration and the hydrophilicity.
The solutions with the highest turbidity are the saltiest and most concentrated in the least hydrophilic products. The BCPS's studied easily dissolves in fresh or saltwater and are stable (they do not separate) at room temperature. The most hydrophilic product solutions are transparent in all concentrations, salinity, and temperature in the ranges assessed. The aqueous BCPS solutions pH, conductivity, and refractive index were also determined. Figure 5 and Figure 6 show an example of the pH and turbidity trends with some of the products evaluated. In the figures shown as iso contour plots. Color shaded areas represent boundaries that are interpolated by the plotting software.
RHEOLOGICAL BEHAVIOR AND INTERFACIAL TENSION
The BCPS solutions viscosity studied increases up to 3 cP (7.3 s-1) when the molecule has Low EO content and medium molecular weight of PO. Based on the salinity and temperature conditions of the reservoir under study, it is observed that viscosity increases exponentially with the product concentration. It also exhibits a shear-thinning behavior following the power Law at Low shear rates (0.1 s-1-1 s-1). For high shear rates, the polymeric surfactant shows a Newtonian behavior, similar to that of water. The reofluidizing feature at Low flow velocities is desirable in injected fluids for improved recovery, as it would improve volumetric sweep efficiency by homogenizing the advancement front of the chemical formulation deep in the reservoir across strata and permeability contrasts zones.
Figure 7 and Figure 8 show viscosity as a function of the shear rate, at two different concentrations of a chosen BCPS, varying the aqueous phase salinity. As observed, BCPS solutions viscosity ncreases with salinity maintaining the shear rate, depending slightly on product concentration. This could represent a technical-economic advantage since, contrary to what occurs with conventional HPAM-type polymers, it would not be necessary to inject high concentrations or soften the injection water to maintain the rheological behavior target.
The oil crude/brine system interfacial tension is considered the most important parameter in EOR processes. It allows to minimize the capillary forces that keep the residual oil trapped in the reservoir Under the evaluated conditions, a trend towards decreasing interfacial tension was observed as both the percentage of EO, and the BCPS molecular weight evaluated increased (see Figure 9), Furthermore, according to the HLD principle, the optimal salinity values correspond to each relative hydrophilicity of surfactants, which vary if the temperature or the oil phase's composition is changed.
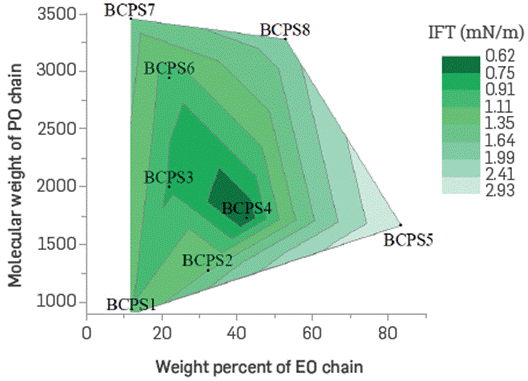
Figure 9 IFT of crude oil/BCPS solution systems containing different molecular weight and EO% in the molecules chain.
Under field case conditions (25°API oil, 60°C, 0.8 wt% TDS), some formulations Lead to interfacial tension values as Low as 0.2 mN/m, representing a reduction of up to two orders of magnitude with respect to the crude/brine system. Despite reducing the BCPS concentration even below 0.2 wt%, the interfacial tension remains Low. It was found that a formulation with the optimal BCPS would be very robustas regards changes in salinity and concentration, which s not common with conventional surfactant formulas (Figure 10), A BCPS4 aqueous solution was chosen for further assessment.
STABILITY OF THE FORMULATION (THERMAL / CHEMICAL / MECHANICAL)
Even high molecular weight polymer solutions are sensitive to high shear rates. HPAM solutions typically do not withstand shear rates above 50,000 s-1, losing more than 50% of their original viscosity. The BCPS's evaluated are much smaller molecules. They self-associate in structures that produce the desired rheological behavior, which could be disrupted by high shear. However, it is formed spontaneously a fraction of a second later. Figure 11 shows an example of shear degradation of polymeric surfactant BCPS4 aqueous solution. The results show that this system does not change the rheological behavior, nor degrade, at shear rates up to 214,000 s-1 On the contrary, the mechanical degradation of high molecular weight (VHM) HPAM is higher than 50% at similar shear rates (Figure 12).
Conventional sulfonated surfactants tend to undergo hydrolysis, precipitation, and HPAM-type polymers chains break under harsh thermal/chemical conditions as when they are exposed to high temperatures and high mono and divalent ions concentrations in brines for an extended time [32],[44]-[47], Nonionic compounds (EO/PO) block copolymers, such as those evaluated in this study, prove to be stable at high temperatures according to thermogravimetric analysis and IFT behaviors. Furthermore, given their nonionic characteristic and their covalent atomic bond, they are not affected by polyvalent ions in aqueous solutions. Additionally, hydrolysis is practically non-existent [38],[48].
The thermal-chemical stability of BCPS4 shows viscosity as a function of the shear rate, and the IFT of crude oil / polymeric surfactant system exposed at 60°C remaining stable over 120 days period. However, the scope of this type of evaluation normally seeks to evaluate stability for periods of 1 to 1.5 years (Figure 13 and Figure 14).
EMULSIONS' STABILITY EVALUATIONS
POTENTIAL IMPACT AT RESERVOIR CONDITIONS
The potential effect of the BCPS4 residual concentrations (400 and 800 ppm) on W/O synthetics emulsions at reservoir conditions was evaluated through changes in BS&W (Table 1) and emulsion viscosity (Figure 15) after gravity separation for 24 hours. For the first stage, demulsifiers were not used to break the emulsion. In this case, both residual concentrations evaluated cause emulsion viscosities below the reference system. This trend is maintained in the different water / crude oil ratios (WOR) evaluated.
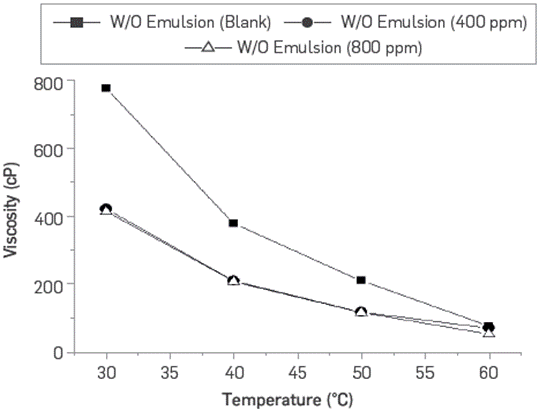
Figure 15 W/O emulsion viscosity vs. temperature comparing two different residual concentrations of BCPS4. Initial WOR 50:50.
Table 1 Effect of BCPS4 composition on BS&W
Emulsion Sample | BS&W (%) | |
---|---|---|
0 hours | 24 hours | |
[Blank] | 20 | 6 |
[400 ppm] | 20 | 0 |
[800 ppm] | 20 | 0 |
The viscosity curves confirm that the residual content of BCPS4 does not affect the W/O emulsion viscosity, nor its stability at reservoir conditions in the absence of emulsion breakers, which is directly associated with the reduction in the BS&W content with respect to the reference system emulsion after 24 hours. In fact, even scanning WOR condition; a greater separation of free water was also observed after 24 hours, confirmed by the BS&W measurements, which in all cases were also below in emulsion with a residual content of BCPS4 as compared to the reference emulsion system.
IMPACT ON OIL DEHYDRATION AND WATER CLARIFICATION PROCESSES
The Oil Dehydration process was carried out at surface treatment conditions (70:30 W:0 mix ratio and 65°C for dehydration process temperature), with 70 ppm of demulsifier added to dehydrate the crude oil according to field conditions. However, considering the Laboratory test scale down, it was necessary to obtain the optimum dose, defined as 380 ppm. It was observed that demulsifiers used in the field case have good performance in the dehydration process, even with BCPS4 residual contents evaluated (100 ppm, 200 ppm) as BS&W values obtained were lower than 2% .
Given the hydrophilic nature of the polymeric surfactant BCPS4, it is expected that the O/W emulsions to be produced will be stabilized. Jar tests were performed to evaluate the water clarification process, adding 6 ppm dosage of clarifier according to the reference system at field conditions. It was evidenced that the O/W emulsions with BCPS4 residual content present higher values of F&O and turbidity compared to the reference even after 24 hours. This confirms that water clarification could be affected as a higher creaming effect in the dehydration process is caused by BCPS4 residual content, Leading to clarifier inefficiency. These results are shown in Figure 16 and Figure 17. This issue should be easily controlled by tuning the demulsifier formulation or choosing a suitable clarifier for the O/W emulsion affected by BCPS residual content, but additional studies are required.
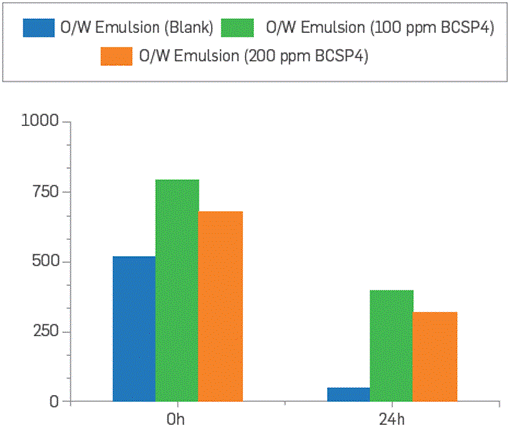
Figure 16 F&O of O/W emulsion comparing two different residual concentrations of BCPS4. Initial WOR 50:50.
STATIC ADSORPTION EVALUATIONS
Figure 18 shows the static adsorption values calculated according to the equilibrium concentration for two BCPS with different molecular weights and relative hydrophilicity.
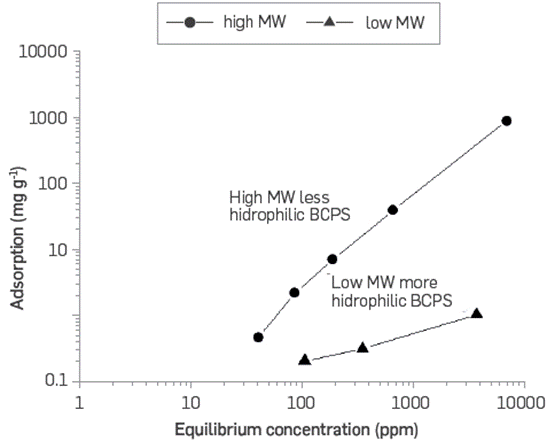
Figure 18 Static adsorption as a function of equilibrium concentration for two different polymeric surfactants at 60°C.
The results suggest that the higher the molecular weight and the Lower hydrophilicity, the greater the static rock adsorption. The difference increases with the corresponding equilibrium concentration; when it is 100 ppm, the adsorption difference is one magnitude order. When it is 1,000 ppm, the difference is two magnitude orders and up to 3 orders when it is 10,000 ppm of the polymeric surfactant.
CONTACT ANGLE EVALUATIONS
Figure 19 shows a contour map with the hydrophilicity degree (abscissa) and the molecular weight (MW) of BCPS's (2,000 ppm) prepared in 0.8 wt% TDS brine (ordinate). The map is parameterized in the iso-curve values on the surface, and the contact angle is measured in the aqueous phase at 22 °C. An oil drop was placed in the center of a glass coverslip, then this set was flipped and placed on top of a squared section glass cell (smaller than the coverslip), filled up to the top with each BCPS solution. Measurement of the contact angle was performed as a function of time until no change was observed, plotting the equilibrium contact angle.
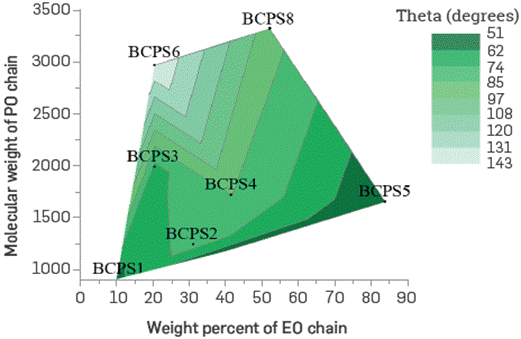
Figure 19 Contact angles as a function of the MW and hydrophilicity degree of polymeric surfactants prepared in 0.8 wt% TDS brine at 22°C (Surface glass).
As can be observed, the contact angle increases as the BCPS molecular weight increases and as the molecule is Less hydrophilic; the Latter is theoretically expected as the product tends to be Located and adsorbed on the oil side, thus favoring the contact of the oil drop with the substrate.
FLOW EVALUATIONS THROUGH POROUS MEDIA.
Figure 20 shows the dynamic adsorption calculated from the injection 5 PV of 1,000 ppm BCPS4 formulation in 0.8 wt% TDS brine and tracer. The sand pack used had 2,680 mD permeability and 0.366 porosity. The procedure was carried out at room temperature and without confining pressure.
It was observed that BCPS4 breakthrough occurred after the njected tracer. This result was expected due to its being adsorbed on sand grains. The calculated adsorption value is 205 ug of BCPS4 per substrate gram. As in static tests, dynamic adsorption could vary depending on the molecular weight and hydrophilicity of the BCPS type. The adsorption behavior will also depend on variations of salinity, temperature, and mineralogy of the porous media. It is worth mentioning that desorption is significant according to the measure in water chase effluents, which would improve recovery factors despite their adsorption.
The resistance factor represents a more realistic quantification of the performance using a non-Newtonian fluid behavior in EOR processes. Figure 21 shows the resistance factor (RF) curves as a function of the BCPS4 flow rates for three different concentrations.
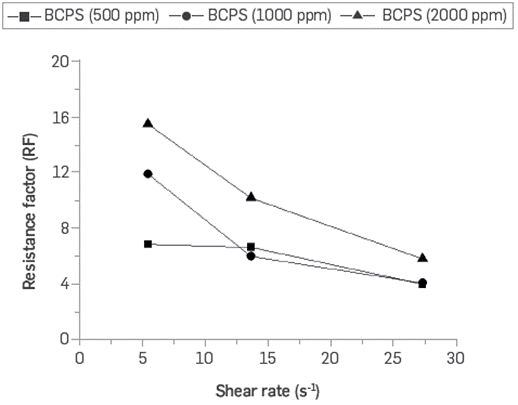
Figure 21 Resistance factor as a function of shear rate for three different concentrations of BCPS4 (0.8 wt% TDS brine, Sw = 1, T= 60°C).
It may be observed that the flow resistance factor can be increased up to 16 times for the solution with 2,000 ppm of BCPS at a shear rate corresponding to 1 ft/day velocity, which is indicated to reduce the mobility ratio, aimed at the surfactant effect of the BCPS4 formulation.
It is worth mentioning that the shear rate undergone by flowing phases inside the reservoir is a function of the distance from the injection wellbore but microscopically it is also a function of the distance from a grain of sand to the neighbor. Shear-thinning fluids typically have a flat velocity profile in most of this section around the center of this microscopic distance, and then very low shear rates (velocity differences).
The flow behavior in bulk (measured by rheometer) and inside the porous media does not necessarily correlate. For example, HPAM solutions are typically shear thinning in bulk, but they thicken in porous media. Spontaneous self-association structures (micelles) of small MW polymeric surfactant forming and breaking in a matter of fractions of seconds bridging with adsorbed molecules on the surface of sand grains, and microscopic low-shear in the center of the pores and throats could explain exceptionally high resistance factors / apparent viscosity of flow behavior inside the porous media BCPS aqueous solutions.
The RF's vs. shear rate in the porous medium shows a decreasing exponential trend. This shear-thinning characteristic in the porous media results in a more homogeneous displacement front, which contributes to an increase in the volumetric sweep efficiency. BCPS's find a larger applicability window because in a low permeability reservoir, the HPAM solution has a high resistance to flow through the porous medium given the elongation effects, thus producing a rapid injectivity loss and a significant process slowdown.
Figure 22 shows the OOIP recovery percentage as a function of the injected pore volumes in the three coreflooding tests. As observed, in the early stage of waterflooding, the oil recovery increases similarly n the 3 cases evaluated. However, the continuous waterflooding process reaches a maximum of 46% of OOIP recovered. In comparison, the displacements with 0.3 VP of HPAM polymer or BCPS4 solutions (0.1 wt%) achieves 62% of total oil recovery.
Figure 23 and Figure 24 show the variation in oil saturation in the two sand packs as a function of the injected pore volumes. The lowest oil recovery in the water injection case corresponds only to a higher oil saturation at the end of the process.
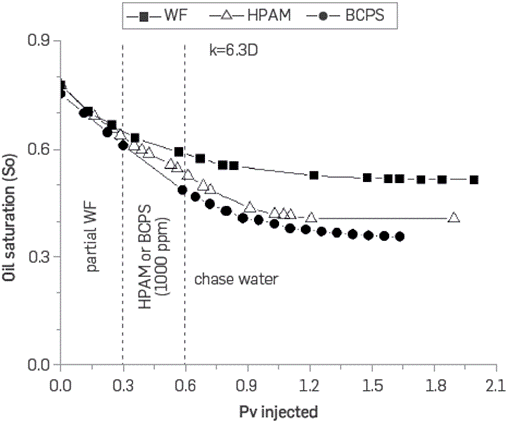
Figure 23 Oil saturation vs. pore volumes injected for the three core floodings in sand pack permeabilities of 6.3 D.
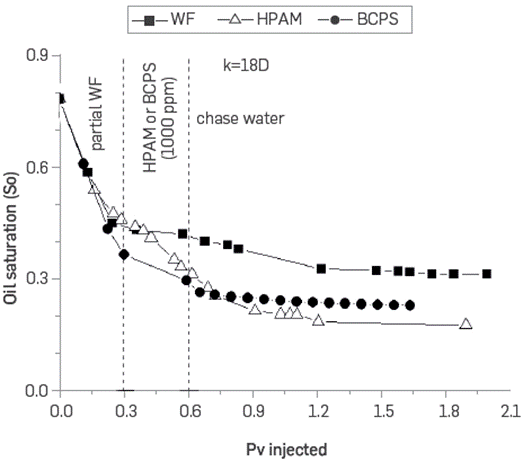
Figure 24 Oil saturation vs. pore volumes injected for the three core floodings in sand pack permeabilities of 18 D.
An interesting difference is observed with the final oil saturation which is lower in the less permeable packaging when using the BCPS4 compared to the HPAM polymer. The result is contrary in the case of the most permeable sand pack. It seems that the IFT reduction has greater impact in terms of the recovery obtained in the medium whose capillary action is more important (lower permeability) and not so much in the most permeable system. It was observed that the BCPS has the advantage of being injected in Low permeability porous media while conventional polymers have a higher flow resistance.
Figure 25 shows the differential pressure as a function of the pore volumes injected for the three cases studied. During the first 0.3 VP of water injected, the differential pressure behaves similarly in all the scenarios evaluated. Nevertheless, significant differences are observed in the HPAM and BCPS injection scenarios. At the same flow rate, the differential pressure increases in the case that the HPAM solution is injected, which is in agreement with the higher RF and RRF of polymer injection processes in porous media as compared to waterflooding or polymeric surfactants. On the contrary, the injection of the BCPS4, the smallest differential pressure values were observed. This can be attributed to the reduction of capillary forces within the porous medium due to the decrease of the IFT obtained by the polymeric surfactant, the decrease in oil saturation in low permeability zones without the resistance or retention effects in the throat pores, and the very low bulk viscosity behavior observed for this solution in the rheometer at the corresponding flow velocities/shear rates,
NUMERICAL SIMULATION AND CONCEPTUAL DESIGN OF THE BCPS4 FLOODING
The experimental evaluation of the polymeric surfactant BCPS4 generated key information required as input parameters for the numerical simulation of this recovery process. Table 2 presents the viscosities in three different concentrations at 10 s-1 which is a representative shear rate at the average flow rate in the reservoir and the rock-fluid interaction parameters of the BCPS4. Table 3 shows the IFT values as a function of BCPS concentration
Table 2 Polymeric surfactant data set for numerical simulation.
Parameter | BCPS4 |
---|---|
IPV (%) | 10 |
Maximum Adsorption (μg/g) | 600 |
RRF | 3 |
μ (350 ppm) cP 10 s-1 | 1.2 |
μ (1,000 ppm) cP 10 s-1 | 1.2 |
μ (2,000 ppm) cP 10 s-1 | 1.2 |
Table 3 Interfacial tension behavior as a function of BCPS4 concentration.
Concentration, ppm | IFT (mN/m) |
---|---|
0 | 25 |
20 | 0.70 |
350 | 0.60 |
1,000 | 0.51 |
2,000 | 0.20 |
The impact of critical parameters on the injection of polymeric surfactant was estimated by evaluating various scenarios considering a sensitivity analysis, as shown in Table 4.
Table 4 Sensitivity analysis variables evaluated for BCPS4 injection.
Parameter | Condition |
---|---|
Chemical concentration (ppm) | 350; 2,000 |
Max. Adsorption (μg/g) | 100; 600 |
Injection rate (BPD) | 1,000; 2,000; 2,500 |
Slug Volume injected (PV) | 0.15; 0.3 |
Injection scenarios | BCPS4 BCPS4 + HPAM |
Figure 26 shows the simulation sector model used, which has a confining ring to represent the reservoir effect on the production pattern. Before BCPS4 injection, the simulation model underwent a primary production stage and a water injection stage. For all scenarios, after the injection of the chemical slug, water was injected until completing 15 years of prediction. The approach used enabled us to identify BCPS4 injection performance with different injection schedules and operating conditions.
It is concluded that the polymeric surfactant adsorption control allows the product to penetrate more through the porous medium, managing to contact more crude oil even in low permeability areas. The reservoir volume affected by the IFT and the viscous displacement is more significant with the use of BCPS4 and the oil recovery.
An improvement in the accumulated oil production was observed at higher injection rates. This behavior is observed for both water injection and BCPS4 solution flooding. On the other hand, the lower the BCPS4 concentration, the lower the amount of oil produced as the performance of the polymeric surfactant is strongly affected by dilution, which occurs when the chemical slug contacts the formation water.
The best simulation scenario was achieved by injecting a total of 0.15 pore volumes of BCPS4 (0.2 wt%) at 2,500 barrels per day, followed by 0.15 pore volumes of HPAM polymer (0.2 wt%) at 1,500 barrels per day. It was not possible to inject the polymer at the same rate as the BCPS4 because, at flow rates above 1,500 bpd, the formation fracture pressure was reached. Furthermore, the BCPS4 injection did not undergo injectivity problems even at 2,500 bpd. Additionally, BCPS4 does not degrade at high injection rates, which does occur with HPAM synthetic polymer, limiting its injection rate (Figure 11). Figure 27 shows the cumulative oil production forecast for 0.3 pore volume injection of HPAM/BCPS4, compared to a water injection scenario (baseline). Oil production increase relative to waterflooding was 238,500 barrels for the BCPS4 followed by HPAM (0.15 PV each) and 168,000 barrels for the BCPS4-only scenario, corresponding to incremental recovery factors of 8.2% and 5.8%, respectively.
Otherwise, Figure 28 shows an acceleration of production due to the HPAM slug injection after the BCPS4 slug. The noticeable decrease in the production rate in this scenario is caused by the injection loss at the beginning of the HPAM slug injection
This scenario maximized oil production by combining two effects simultaneously. First, the injection of BCPS4 slug allows the low interfacial tension and simultaneous mobility control to release part of the oil that is immobile to the water due to capillary action. Second, the polymer injection allows mobile oil to be displaced by a more viscous fluid than the water and the polymeric surfactant.
Nonetheless, according to the results obtained, it can be concluded that BCPS injection in the early stages of secondary recovery could increase the final recovery factor even without the need to use HPAM polymer. This is mainly because the decrease in interfacial tension, due to the injection of BCPS, is enough so that part of the immobile crude oil can now be pushed and produced by injecting a displacing fluid such as water or polymer solution.
Based on preliminary assessments for field implementation, the injection of polymeric surfactants will not require special injection facilities. Its injection can be performed by dosing the chemical in the water flow lines, combined with static mixers thus simplifying the operation and reducing implementation costs. However, this is beyond the scope of this paper and will be addressed in a future publication.
CONCLUSIONS
The addition of small amounts of a properly selected polymeric surfactant (BCPS) in injection brine can decrease the interfacial tension of the water-crude oil system and promotes a favorable change in wettability. IFT remains low over broad BCPS concentration and salinity ranges, making these formulations highly robust. At low BCPS concentration, the increase in viscosity is limited, but still, the rheological behavior promotes a mobility ratio improvement in the porous media. Additionally, polymeric surfactants are mechanically and thermo-chemically stable molecules, conserving their ability to favorably modify the brine rheological behavior and reduce the TIF in the water/crude system simultaneously.
BCPS adsorption is moderate and was set at 300 μg/g in the simulation. However, the desorption observed in the front of water post-flow for the dynamic test is significant and favors the recovery process. Also, from the RF calculations, a reofluidizing behavior characteristic is observed within the porous medium that controls the displacement front's mobility, contributing to improve the volumetric sweep efficiency. The displacement efficiency test shows that 0.3 VP of BCPS4 injection achieves a total recovery of up to 62% of the original oil in porous media at laboratory scale.
The BCPS4 content does not affect the W/O emulsion stability. It, undoubtedly promotes a reduction of the emulsified crude oil viscosity, possibly due to the action of residual BCPS as a demulsifier. In fact, when the oil fraction is higher in the production WOR, the emulsified water separation is favored showing a better performance of the emulsion breaker W/O. Nevertheless, the water clarification may be affected as it is residual from BCPS4, promoting higher levels of fats & oils and turbidity in the aqueous phase and higher stability in O/W emulsions. Therefore, work is required in the fluid treatment additives formulation.
Numerical simulation shows that incremental oil production of up to 238,500 BO, corresponding to an increase of 8.2% recovery factor, can be achieved by injecting a total of 0.15 pore volumes of BCPS4 (0.2 wt%) at 2,500 barrels per day, followed by 0.15 pore volumes of HPAM polymer (0.2 wt%) at 1,500 barrels per day. Given its characteristics and the proposed injection design, the product can be dosed directly in the water injection line to the well without requiring high capital expenditures.