ENHANCED OIL RECOVERY
After the primary reservoir production, it is necessary to maintain reservoir pressure and drive additional oil out of the rock formation by injecting exogenous fluids, such as water. Water injection or water flooding is the most common oil recovery method [1]. The efficiency of this method is governed by the mobility ratio between the displacing fluid and the oil (displaced fluid), which is a combination of their viscosities and effective permeabilities. The higher the water-oil mobility ratio, the less effective is the displacement of oil by water [2] as a result of preferential paths. This leads to poor sweep efficiency, which is detrimental to the oil recovery process [3]. Water mobility can be several orders of magnitude higher than the oil one, especially for medium-to-heavy oils and oil-wet formations [4]-[6], which results in inefficient waterflooding processes,
ROLE OF POLYMERS IN ENHANCED OIL RECOVERY
Injecting polymeric solutions instead of water can reduce the mobility ratio. This mechanism is called mobility control, which leads to a more stable aqueous front, increasing the oil sweep efficiency. A relatively low polymer concentration (hundreds of parts per million, ppm) can significantly increase the injection solution viscosity if the polymer molar mass is high enough. The water phase viscosity increases, changing the aforementioned mobility ratio to more favorable values for a specific permeability condition [7][8], increasing oil sweep efficiency [3].
Polymer molecules experience retention when flowing through a porous medium [2]. This retention, caused by physical adsorption, mechanical entrapment in pore throats, and hydrodynamic retention, reduces the rock formation permeability. This phenomenon retains polymer from the flowing solution and reduces the polymer flooding performance. Conversely, the permeability reduction can increase the oil sweep efficiency by improving the chase water sweep efficiency after a polymer slug injection [9], [10].
Due to the polymer advantages, many operating oil companies have employed polymer flooding for enhanced oil recovery (EOR) since the 1960s [11], [12]. This technique has been applied successfully for EOR up to oils of 10000 mPa.s in reservoirs with high permeability [6], [9], [13], [14].
Partially hydrolyzed polyacrylamide is the most used commercial polymer for polymer flooding to enhanced oil recovery. The low price, high commercial availability, and easily customizable properties are the main competitive aspects [15]. However, unmodified polyacrylamide is sensitive to salt and has poor thermal stability [16].
Biopolymers are promising alternatives to synthetic polymers because they have great resistance against mechanical degradation, high temperatures, high salt content, wide pH ranges, and are eco-friendly [17], [18]. Additionally, they are extracted from renewable materials like plants or microorganisms. Despite their advantages, they can suffer biological degradation, and their cost is usually high, so their use needs to provide better oil recovery performance. As mentioned before, several aspects need to be considered to use a polymer in the field. First, the initial screening based on the reservoir conditions should show the polymer flooding as an applicable EOR technique [13], [19]. Then, laboratory-scale studies, such as rheology, filterability, degradation resistance, are necessary to better understand how a particular polymer performs at reservoir conditions. The next step consists of developing simulation models based on the laboratory data and upscaling them to include additional geological aspects of the reservoir and well injector/ producer geometry and location. Lastly, field pilot tests and full field-scale models provide information and risk analyses to the field operator [13], [19]. Ferreira and Moreno [19] summarized these stages as a workflow, and the content of the present paper corresponds to the first stage of the laboratory studies, which is the rheological evaluation.
Bulk rheology tests are fast and relatively low-cost and can provide information such as thickening capacity, pseudo-plasticity, resistance to salt, and temperature. The behavior of apparent bulk viscosity against polymer concentration offers the first estimate of the required polymer content to reach the target viscosity of the injected fluid for a particular reservoir.
The following sections show general aspects regarding laboratory studies.
CONDITIONS AFFECTING POLYMER PERFORMANCE
The polymers can be exposed to high salinity, temperature, and shear in the reservoir during the polymer flooding process. Polymer filterability, precipitation, flocculation, adsorption, and mechanical, thermal, biological, and chemical degradation are phenomena to consider when selecting a polymer [20]. All of these conditions affect the polymer rheological behavior in different ways.
After the initial screening, the polymer concentration range has to be selected. A suitable polymer solution for EOR must contain the minimum amount of polymer to improve mobility control. A reasonable maximum polymer content is in the order of 3000 ppm (parts per million) [21], and the world average is around 700 ppm [22]. Offshore applications present additional challenges related to platform space and logistics, pushing the maximum working polymer concentration downwards [23].
As the polymer content increases, the polymer chains start to interact [24], resulting in different concentration regimes. That affects the amount of polymer that has to be added to increase viscosity. In the dilute regime, hydrodynamic interactions start to appear [25], and when there are significant entanglements between different chains, the solution enters the semi-dilute regime. This broad transition can be characterized by the overlap concentration, c* [24], [26].
The overlap concentration allows one to estimate the mass of polymer to be added to the solution. Using polymeric solutions in concentrations below c* results in low thickening capacity per mass of polymer added and less pseudo-plasticity, which excludes the injectivity advantages of more viscous solutions. Additionally, below c*, a lower relative amount of polymer is adsorbed [27], [28], which is preferable.
Temperature dependence is also relevant when choosing a polymer. Polymeric solutions typically experience a viscosity decrease as temperature increases [29]. One possible model to explain the effect of temperature on the viscosity of fluids is the Eyring equation for viscous flow [30], modified from the Arrhenius equation. Many authors have applied the Eyring equation to study polymer melts [31]-[33] and polymer solutions [34]-[39].
In addition to temperature, salinity is also very relevant for polymers in EOR. Seawater is usually used at offshore platforms, and it mixes with reservoir water, which can have a high amount of total dissolved solids (TDS). Higher salinity leads to changes in electrostatic interactions in solution, can affect polymer hydration, and can lead to coil shrinkage, collapse, or crosslinking, depending on the ion. [40], [41] Therefore, salinity needs to be considered in a study.
BIOPOLYMERS
Biopolymers can be advantageous for EOR due to their good performance in terms of resistance to mechanical degradation, temperature, and salt content. Xanthan Gum, Schizophyllan, Scleroglucan, and Guar Gum were chosen as the test polymers. Previous works have studied Xanthan Gum [16], [25], [35], [42], [43], Guar Gum [43], Scleroglucan/Schizophyllan [16], [18], [42]-[45], focusing on EOR or pore medium flow behavior. This paper studied the bulk rheology of those biopolymers under different conditions. Xanthan Gum is produced by the bacterial species Xanthomonas Campestris. It has high molecular weight, 2 to 10.5 MDa [46]-[48] and excellent viscosifying properties [3], [15], [49]. Its backbone is composed of a (1→ 4)-β-D-glucopyranosyl units, the same as cellulose. It has a trisaccharide lateral group every two glucopyranosyl units, composed of, from outside-in, β -D-mannopyranosyl(1→ 4) β -D-glucuronopyranosyl-(1→ 2)-α-D-mannopyranosyl6-acetate-(1→ 3)-(backbone), and some of the farther mannopyranosyl groups have a 4,6-O-pyruvyl cyclid acetal group [47], [49]. Xanthan Gum in solution has a five-fold helix shape, often in the form of double-helixes or bundles of double-helixes, formed by hydrogen bonding between the chains and ionic interactions [35], [46], [49]. This secondary structure results in a stiff, rigid rod that can suffer transitions depending on the salt content and temperature [46], [47]. This polymer is the basis of the comparison of this paper due to its ubiquity. Scleroglucan is a (1→ 3) β -glucan polysaccharide produced by the fungi from the Sclerotium genus [50], [51]. Schizophyllan, on the other hand, is produced by Schizophyllum commune, and it has the same overall structure as Scleroglucan [52], [53], but with some measurable differences [53] such as their degree off substitution and branching [54]. The molar mass of Scleroglucan and Schizophyllan ranges from 2-13 MDa [48], [53], [55]-[58]. These polysaccharides are characterized by one main chain of (1→ 3)- β -D-glucopyranosyl groups, and, in every third unit, there is a (1→ 6)- β -D-glucopyranosyl branch [51]. In solution, Scleroglucan/Schizophyllan adopt a triple helix structure, forming a straight cylinder [59] due to cooperative interactions between chains. The cultivation, microorganism strain, synthesis, and extraction all influence the molar mass of these polymers [48], [49], [52]. It is recommended to add biocide or kill the bacteria before injection [15], although we did not observe any biological degradation for Scleroglucan during the time necessary to perform the experiments.
Guar Gum is extracted from the endosperm of the guar beans, Cyamopsis tetragonoloba [60], [61]. It is composed of a backbone of (1-4)- β -D-mannopyranosyl groups, and every other mannose residue, there is a (α1-6 β) linkage to a single α-D-galactopyranosyl group. It is part of a larger class of biopolymers called galactomannans, where the difference is the ratio of mannose and galactose, which varies among species. Its molar mass has been estimated to be between 0.5 to 2 MDa [62], [63]. These polymers in solution form random coils [64] despite their relatively rigid chains, although polymer-polymer interactions are reported [65], [66], such as superstructures and hyper entanglements [63], [67]. Previous studies of Guar Gum have revealed a relatively low thickening capability and viscosity retention compared to Xanthan Gum and Scleroglucan [43].
Regarding their properties for EOR, Scleroglucan/Schizophyllan have a much less marked interaction with carbonates [68]-[70] and suffer less influence by ionic strength or temperature than Xanthan Gum [15], [58], [71]. They are resistant to pH values from 2 to 12 [18], [48], but the triple rods can be melted at higher pH values [48], [53]. An extensive comparative study of several polymers, including Xanthan Gum, Scleroglucan, and Guar Gum, found that Scleroglucan has the most potential for North Sea use [43].
This work aims to provide a robust set of bulk rheology data for the solutions of four biopolymers: Schizophyllan, Scleroglucan, Xanthan Gum, and Guar Gum. This data set will encompass shear rates, polymer/salt concentrations, and temperatures analogous to reservoir conditions, focusing on, but not limited to, the Brazilian pre-salt fields. Model parameters will be obtained, allowing rheological properties under different conditions to be calculated with accuracy.
REAGENTS AND SYNTHETIC BRINE COMPOSITION
Table 1 lists the reagents used in this study, their supplier, and purity. The suppliers of Xanthan Gum, Guar Gum, and Scleroglucan did not provide any information about the purity or purification methods. More information on the production of Schizophyllan used in this work can be found in reference [72].
Table 1 Reagents used in the study, the supplier, and informed purity.
Reagent | Supplier | Supplied purity |
---|---|---|
Xanthan Gum (XG) | Sigma Aldrich | N/A |
Guar Gum (GG) | Sigma Aldrich | N/A |
Scleroglucan (SCLG) | Carbosynth | ≥90% |
Schizophyllan (SCP) | BIOINOVAR-UFRJ | N/A |
Sodium chloride | Sigma Aldrich | ≥99% |
Potassium chloride | Sigma Aldrich | ≥99% |
Calcium chloride dihydrate | Sigma Aldrich | ≥99% |
Magnesium chloride hexahydrate | Sigma Aldrich | 99-102% |
Strontium chloride hexahydrate | Sigma Aldrich | ≥99% |
Iron(II) chloride tetrahydrate | Sigma Aldrich | ≥99.0% |
Lith urn chloride | Sigma Aldrich | ≥99.99% |
Sod urn bromide | Sigma Aldrich | ≥99% |
Sod urn sulfate | Sigma Aldrich | ≥99.0% |
Sod urn propionate | Sigma Aldrich | ≥99-100.5% |
Sod urn acetate | Sigma Aldrich | ≥99.0% |
Sod urn formate | Sigma Aldrich | ≥99.0% |
Glutaraldehyde | Sigma Aldrich | 50% in water |
Table 2 lists the brine composition for the Synthetic Sea Water (SSW) and the mixture of 80% SSW and 20% Synthetic Formation Water (SFW), hereafter called merely 80:20. The brine 80:20 was used to simulate the mix between seawater and reservoir formation water during the injection process. The quantity of each salt was corrected by the water content of each hydrated salt.
Table 2 Brine composition in parts per million (ppm) of each salt.
Salt | SSW | 80:20 |
---|---|---|
Potassium chloride | 749.3 | 1,570.3 |
Calcium chloride dihydrate | 484.2 | 10,435.9 |
Magnesium chloride hexahydrate | 1,271.3 | 4,958.2 |
Strontium chloride hexahydrate | 5.2 | 1,852.6 |
Iron(II) chloride tetrahydrate | 2.0 | 9.4 |
Lith urn chloride | 1.2 | 74.3 |
Sod urn bromide | 82.4 | 327.1 |
Sod urn sulfate | 57.7 | 71.6 |
Sod urn chloride | 28,251.3 | 55,640.7 |
Sod urn propionate | 0.0 | 5.8 |
Sod urn acetate | 0.0 | 54.2 |
Sod urn formate | 0.0 | 24.8 |
TDS (%) | 3.01 | 6.91 |
POLYMER SOLUTION PREPARATION.
The Recommended Practice 63 by the American Petroleum Institute (API RP63) [73] states that, to prepare the polymer solutions, the polysaccharide powder should be sifted onto the brine under highspeed stirring and that this procedure should be adapted depending on the manufacturer's recommendation.
The providers of Scleroglucan (Carbosynth), Guar Gum (Sigma Aldrich), and Schizophyllan (BIOINOVAR) did not provide any specific instructions on how to perform solubilization. The procedure by Cargill [74], one of the main producers of Scleroglucan, specifies that either brine or water can be used, that high shear is required, and that the process is completed after a few minutes of stirring.
Those recommendations were suboptimal for the Scleroglucan and the Schizophyllan used in our work. The polymer solutions were prepared according to the protocol developed by Rueda and Moreno [39]. They found that the procedure to solubilize Xanthan Gum in API RP 63 did not provide a well-hydrated polymer solution, so the authors developed a new method. The key of the process was to use deionized water to prepare the polymer stock solution, with later dilution with brine. This polymer preparation procedure was adopted and applied to all polymers studied to minimize potential differences in the viscosities due to preparation. Several other solubilization methods were also tested, including using high temperature and high shear, but none were as efficient as the one presented herein Detailed descriptions are given as follows. First, a 4,000 ppm stock solution of polymer was prepared using Deionized Water (Dl) with a resistivity of 18.2 MQ.cm. This was performed by weighing an appropriate amount of water in a container with a threaded lid and weighing the powder polymer amount in a separate flask. Stirring was turned on to form a large vortex where the polymer powder was added slowly to maximize the polymer dispersion and hydration. For 1.0 g of polymer, the addition of powder took approximately 15 minutes. That was performed at room temperature (25°C). Guar Gum required 4,000 ppm of glutaraldehyde to be added to mitigate biodégradation.
After adding the polymer, the containers were closed. The solutions were stirred at room temperature at the maximum possible velocity of the magnetic stirrers (model 114-1 from Ethik Technology) for 24h, except Scleroglucan, which required 7 days. This period was determined by observing when the solution viscosity, measured with the rheometer, stopped changing. More details about the rheological analysis are provided in the next section.
The polymer concentrations used in this study were 2,300,1,300, 750, 450, 250,175, 50, 25,10 ppm, which were obtained by diluting the stock polymer solution with an appropriate brine. This logarithmic range encompasses the possible polymer concentrations that can be used in the field and also allows the parameters described in the next sections to be calculated. No extensive filtration tests were performed in this study, but they are essential for core-flooding, which is the next step of this study.
POLYMER SOLUTION ANALYSIS
A Thermo Scientific HAAKE MARS III rheometer was used to obtain the flow curves of each polymer solution. The measuring geometry was a coaxial cylinder Z41 TLB08031, with 41.35 mm of inner diameter, 43.25 mm of outer diameter, and 55 mm of Length. A modular temperature controller MARS TM-Li-C kept the temperature within 0.1°C of the target temperature. The temperature of 60°C was used to simulate reservoir conditions, but additional measurements were also performed at 25, 40, 50, and 70°C. The gap between the measurement cup and the spindle used was 3.000 mm, which was re-determined at each temperature. A solvent trap was used for measurements at 70°C to limit solvent Loss through evaporation.
Apparent viscosity measurements were acquired under controlled shear rates, from 0.001 s4 to 1,000 s_1. The integration time was 3 seconds and the minimum wait time for stabilization was 15 seconds. This procedure was strictly the same for all solutions and all temperatures.
DATA ANALYSIS
Some experimental data points were discarded because of torque sensitivity limitations of the rheometer, measuring geometry inertia, and Taylor vortices, according to the recommendations in Ewold et al. [75], From this corrected dataset, a few parameters were estimated.
The Power Law, also known as the Ostwald-de Waele model, was fitted to the flow curves using least-squares regression. This model is described by
Where η is the apparent viscosity (mPa’s), K is the consistency index (mPasn), n is the flow behavior index (unit-Less) and y is the shear rate (s-1). For pseudopLastic fluids n<1, for Newtonian fluids n=1 and thickening fluids have n>1. For more viscous solutions, K is higher, and vice-versa.
The overlap concentration c* was estimated by finding the intersection of two straight Lines in a Log-Log plot of viscosity by polymer concentration. Each Line corresponds to one concentration regime (dilute and semi-dilute).
One possible way to compare the viscosity behavior of different polymers under the dilute and semi-diluted regime is through the quality value Z, proposed by Akstinat [20], which has the form of
where c p is the polymer concentration (ppm).
Temperature effect on viscosity was obtained by the simplified Eyring equation, which is defined as [36]:
where A is a preexponential term (mPa⋅s), E a is the activation energy (kJ⋅mol -1 ), R is the gas constant (8.314 J⋅mol -1 ⋅K -1 ), and T is the temperature in Kelvin. Using this method, the viscosity sensitivity to temperature can be summarized with a single number. Equation 3 can be linearized by applying a natural logarithm to both sides of the equation and by considering 1/T as the independent variable.
A Linear regression informs both the pre-exponential term and the activation energy.
The Debye shielding Length can determine the degree of shielding caused by ions in solution, k1. For a 1:1 electrolyte at 25°C, this Length can be simplified to Equation 4 [41], where ["Electrolyte"] is the molal salt concentration (mol.kg -1 ), and k 1 is in nm.
3. RESULTS AND DISCUSSION
First, the general rheological behavior of the polymeric solutions is shown and discussed, using the flow curves at 60°C in SSW as representative of the general observed trends. Then, the overlap concentration values are shown and discussed. The activation energy determination follows this, and then the power-law fitting parameters of all the flow curves in all conditions are discussed.
GENERAL RHEOLOGICAL BEHAVIOR OF THE POLYMERIC SOLUTIONS.
Figure 1 contains the flow curves for every polymer studied, at every studied polymer concentration, at 60°C, in SSW. The curves in 80:20 brine are very similar, and so will not be shown.
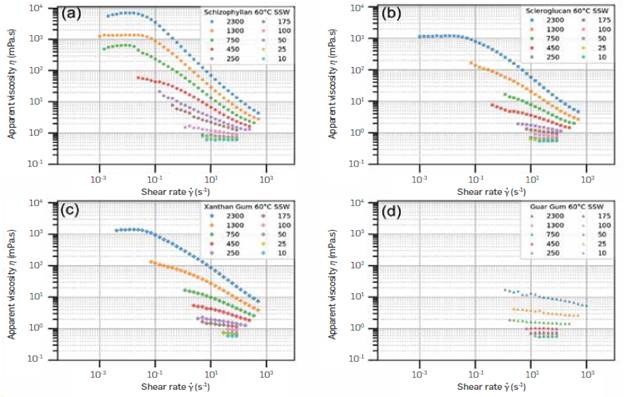
Figure 1 Flow curves of (a) Schizophyllan (SCP), (b) Scleroglucan (SCLG), (c) Xanthan Gum (XAN), and (d) Guar Gum (GG), at concentrations ranging from 10 to 2,300 ppm, at 60°C, in SSW.
Scleroglucan, Schizophyllan, and Xanthan Gum have very similar behaviors. Schizophyllan showed the highest viscosity of all biopolymers at the same concentration, especially at lower shear rates, suggesting its potential use as an EOR additive.
Guar Gum exhibited pseudoplastic behavior only above 750 ppm and was undistinguishable from a Newtonian fluid at lower concentrations. The same results were previously reported for galactomannans [65], [66], Guar Gum has been found to enhance oil recovery, although, as shown here, it requires higher concentrations than the other polymers [76], That may be due to its different conformation. Whereas Xanthan Gum and Scleroglucan form helixes and behave like rigid rods [46], [59], Guar Gum has a conformation more similar to a random coil [64], which occupies Less hydrodynamic volume and, therefore, has Less effect on the viscosity. That aspect will manifest itself again when discussing the overlap concentration.
Xanthan Gum is a polyelectrolyte and should, in principle, be affected by salinity changes. However, this was not observed here (see Figure 2) to a significant degree. That has already been reported in the Literature [35], [48], [77], On the other hand, Scleroglucan was also unaffected by salinity It is intrinsically nonionic, so it should be a better candidate for pre-salt reservoirs (composed of carbonates, with positive surface charges) than the negatively charged Xanthan Gum [78], The disadvantage is its very Long preparation time.
The pseudoplasticity of Xanthan Gum and Scleroglucan also allows for better injectivity control. Increases in pump speed will yield Lower viscosity fluids due to shear thinning. Guar Gum, on the other hand, will not provide this benefit. However, Guar gum is a possibility if a high viscosity Newtonian fluid is desired.
Another way of visualizing the differences between the polymers is by fixing the shear rate and comparing the viscosity differences. That has to be performed on a shear rate that presents useful rheological data in all conditions for a better comparison. Figure 2 shows the viscosity versus polymer concentration at 42 s-1 the reference shear rate value that is common to all concentrations of all polymers studied, in SSW and 80:20 brine, at 60°C. The dashed Lines indicate the calculated mean overlap concentration.
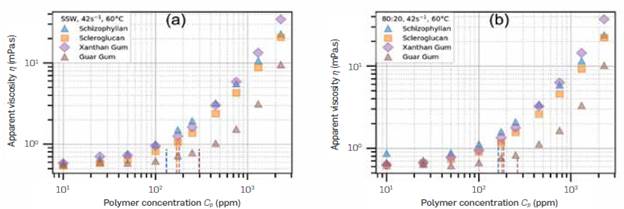
Figure 2 Viscosity at y =42s-1 for the polymers studied, in every concentration, at 60°C, in (a) SSW and (b) 80:20 brine.
In Figure 2, as the polymer content increases, the viscosity values increase. The polymer solutions separate into two groups, Guar Gum, with Lower viscosities, and Schizophyllan, Scleroglucan, and Xanthan Gum, with higher viscosities. The higher viscosities of Schizophyllan observed in Figure 1 are not necessarily observed in Figure 2 due to its higher flow behavior index n.
Instead of the viscosity itself, the quality value Z (defined in Equation 2) can be calculated. This parameter is shown in Figure 3 as a function of polymer concentration. The dashed Lines indicate the mean overlap concentration.
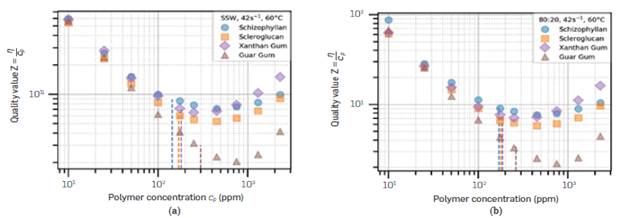
Figure 3 Quality Value Z (multiplied by 103) for the polymers studied, in (a) SSW and (b) 80:20 brine using viscosity at y =42 s-1
The quality value is high at low polymer concentrations due to the vanishing value of c p , but as the concentration increases, it reduces due to the poor viscosity scaling in the dilute regime. After c p >c* , the viscosity scaling is higher and Z starts to increase.
This transformation separates the four polymers, making Xanthan Gum slightly better than Scleroglucan and Schizophyllan purely from a thickening power perspective, at y=42 s-1.
These curves can be used to quickly judge the polymer cost-effectiveness at 60°C based on estimates of local costs, and the resulting vertical shifts of these curves (Z' = η/c p xcost ). For example, a 1,300 ppm solution of Guar Gum (Z≈2.5), compared to 1,300 ppm of Xanthan Gum (Z≈d0), would need to be a quarter of the price to compensate the higher thickening power of Xanthan Gum. However cost estimates are inaccurate at this moment since Schizophyllan was produced on a small scale by a partner laboratory, and its cost is high. Besides, this method does not consider the mechanical or thermal resistance, so this comparison should be coupled with additional rheological data.
The higher salinity of the 80:20 brine did not affect any polymer solution significantly, especially Xanthan Gum. However, it could be the case that SSW contains sufficient ions that every carboxylate group is effectively electrostatically shielded from any charged neighbor (i.e., the Debye shielding length is lower than the distance of the charges). Considering the approximate composition for the SSW brine as a 3% NaCl solution, which is equivalent to 30g NaCl/970g of H2O, or 0.53 molal, and placing this value in Equation 4, a k -1 = 0.41 nm is obtained, or about 2.7 sp3-sp3 C-C bond lengths. That means that every 0.41 nm around the carboxylate, the electrostatic potential falls by 1/e. An upper bound for the shielding length can be inferred from Rau et al. (1990), where it was shown that brines with more than 0.01 mol/dm3 NaCl did not affect viscosity. That is equivalent to approximately k -1 ≈ 3 nm. Therefore, the thickening and solubilizing capabilities of Xanthan Gum in these brines with higher salinities are due to the solvophilicity of the rest of the polymeric chain, with minimal contribution from electrostatics. Similar results were already observed for other brines [35],[79].
OVERLAP CONCENTRATION (C*)
Figure 4 shows an example of the c* determination using Scleroglucan at 60°C in 80:20 brine. Repeating this procedure for all polymers and all concentrations, the c* of every polymer under all conditions can be compared. The small vertical bars over the symbols represent the region considered for each linear fit, which is represented by dashed lines. The vertical line down to the abscissa represents the intersection of both fits.
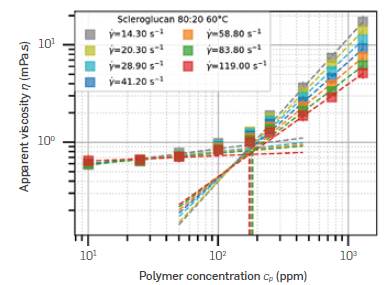
Figure 4 Overlap concentration (c*) determination for Scleroglucan in 80:20 brine at 60°C, in several shear rates.
Figure 5 presents a comparative chart of the overlap concentrations for all polymers in their respective reliable shear rates. Filled markers symbolize SSW, whereas empty markers represent 80:20 brine.
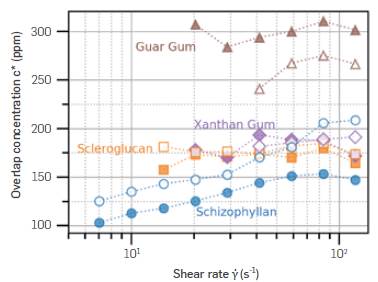
Figure 5 Overlap concentrations for every polymer studied, scanning shear rates in two different salinities.
From Figure 5, the overlap concentration of Xanthan Gum and Scleroglucan is almost constant in the tested shear rate and salinity ranges. Guar Gum and Schizophyllan both seem to be affected by the brine, although in opposite ways. A decrease in c* of the same polymer is a result of an increase in its volume, since c*≈N/R 3 F , where N is the number of segments and R F is the radius of the polymeric molecule [26]. Based on that relationship, it could be inferred that Guar Gum presents a larger hydrodynamic radius in 80:20 brine than in SSW. The opposite would be valid for Schizophyllan. However, small differences such as these could be inside the margin of error for these estimates due to the logarithmic scales of the plot and the experimental uncertainty associated with viscosity measurements. Additionally, the manual attribution of points to the dilute regime, the transition region, and the semi-dilute regime can lead to more variations. On the other hand, the difference of 100 ppm between Scleroglucan/Xanthan Gum and Guar Gum is sufficient to separate them with confidence.
The rising c* value for Schizophyllan and its lower value is different from the constant, higher c* value for Scleroglucan, despite their identical structures and ionic characteristic. The differences in c* values could likely be attributed to different molar masses of these polymers (as mentioned in the Introduction), differences in the amount of branching, and different expansions. [53], [54]
Table 3 shows a comparison of the overlap concentrations determined in this study and those found in the literature. There is high variability in the values. That is due to several factors, such as different solvents, temperatures, shear rates, measurement methods used, differences in biopolymer batches, and extraction methods. The c* concentrations found here are in the same concentration range as the Literature reported. As mentioned in the Introduction section, the overlap concentration is not a strictly defined value [24], [26], and its relevance in this study is as a comparison among polymers and their sizes, and as a guideline for the target polymer concentration to be used.
Table 3 Overlap concentration obtained in this study, compared to the literature
SSW | Mean c*/ppm | Literature c*/ppm | Solvent | Temperature | Reference |
---|---|---|---|---|---|
Xanthan Gum | 184 | ~2,000 | 20-220 kppm NaCl | 23-77°C | [35] |
280 | 5,800 ppm NaCl | 25°C | [80] | ||
126 | 5800 ppm NaCl | 25°C | [81] | ||
~2,000 | Distilled water and 2,900 ppm NaCl | 23°C | [82] | ||
650,1,000, 1,200 | 7,100 ppm KCl, 1,100 ppm NaCl, Distilled Water | n/a | [83] | ||
200 | 3,000 ppm NaCl | 25°C | [84] | ||
50-60 | SSW (36014 ppm TDS) | 25,60°C | [79] | ||
Guar Gum | 281 | 550 | Water +10% sucrose | n/a | [64] |
1,800-5,800 | Water +10% sucrose | 25°C | [85] | ||
1,300-4,500 | acetate buffer | n/a | [66] | ||
2,300 | 0.015 M NaCl | 25°C | [86] | ||
Scleroglucan | 174 | 150-200 | 20,000 ppm KCl | 30°C | [69] |
43-71 | SSW | 20, 60°C | [79] | ||
Schizophyllan | 148 | ~80 | O.OlNNaOH | 25 °C | [87] |
The 100 ppm c* difference between Guar Gum and the other polymers is probably due to the smaller molar mass and the random coil conformation of Guar Gum compared to the other polymers rigid rods that can be visualized by the ratio between the radii of both flowing molecules. That can be roughly estimated from the c*values [c*≈N/R
3
F
). Supposing the molar mass of Guar Gum as 2 MDa, of Scleroglucan as 4 MDa (upper range for Guar Gum and middle range for Scleroglucan), and assuming that both have similar quantities of sugar groups for the same Length, a ratio
of is found, which results in -
That is, the radius of the Guar Gum molecule is around two-thirds that of Scleroglucan. This is relevant when considering that these molecules will be forced through pore throats that can have similar sizes to their radii.
Regarding the applicability for EOR, the smaller the overlap concentration, the Lower is the minimum required quantity of polymer to achieve the viscosity target for EOR applications. Therefore, using Xanthan Gum as our basis, it can be seen that Schizophyllan would require Lower concentrations than Xanthan Gum to reach a target viscosity. Despite having the same structure as Schizophyllan Scleroglucan has a different rheological behavior, very close to Xanthan Gum. The similarity of Scleroglucan to Xanthan Gum has already been observed [16], [20], [43], On the other hand, Guar Gum has a much Lower viscosifying effect and higher c* values, making it, at first glance, a poor substitute for Xanthan Gum.
TEMPERATURE AND ACTIVATION ENERGY (Ea)
Temperature control is of great importance in rheology, as viscosity generally varies exponentially with temperature. The activation energy was quantified by a Linearization of the Eyring equation Equation 3. Figure 6 shows one example of Xanthan Gum in SSW at 25, 40, 50, 60, and 70 °C. The viscosity used was at y=42s_1, for it is the shear rate that most experiments have in common. The dashed Lines represent Linear fits.
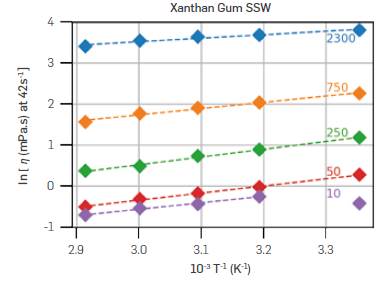
Figure 6 Linearized Eyring equation plot for Xanthan Gum in SSW and five polymer concentrations (in ppm, in labels close to the lines).
Repeating this process for all polymers in SSW and for Schizophyllan in both SSW and 80:20 brine, the activation energies and the pre-exponential term can be compared. It is important to note that, in some cases, measurements from high or Low temperatures had to be discarded to perform the Linear fits, as they deviated strongly from the trend. Figure 7 shows how the activation energy for viscous flow (E a ) and the pre-exponential parameter (A) depend on the polymer and its concentration. Full symbols represent SSW, and hollow symbols represent 80:20 brine. The horizontal black dashed Line in both graphs represents the calculated value for the brine. The red Line indicates E a =0.
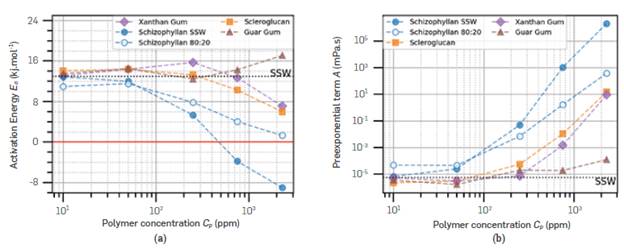
Figure 7 (a) Activation energy (E a ) in kJ/mole by polymer concentration (cp) in ppm (b) Pre-exponential parameter A, in mPa.s, by polymer concentration.
First, it is necessary to establish a Line of comparison to a known quantity. That was performed by calculating the activation energy of SSW, which was 12.9 kJ.mol-1. The value calculated from Table 2 by Kestin et al. (1981) is 15.54 kJ.mol-1 for a 300,00 ppm NaCL brine, 12% higher when compared to the value obtained here. Considering that these viscosities are above but close to the Lower measuring Limit of the used rheometer, the difference is understandable and caused by equipment precision.
All dilute polymer solutions have activation energies close to water, which is due to their minute concentration. As the concentration ncreases, a few divergences appear. Guar Gum becomes more affected by temperature than water, whereas Xanthan Gum Scleroglucan, and Schizophyllan were Less. The activation energy of Schizophyllan in SSW became negative at higher concentrations, a physically impossible value which means the viscosity would become more elevated. However, this was performed without considering the viscosity at 70 °C, deviating from the Linear trend. If the deviating data point is considered, the trends of 80:20 brine and SSW will overlap and approach E a =0 at higher polymer concentrations.
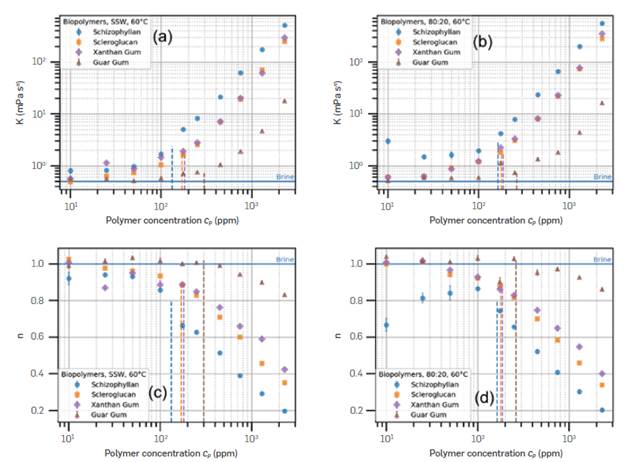
Figure 8 Comparison of the fitting parameters K (a, b) and n (c, d) for the biopolymers in SSW (a, c) and 80:20 (b, d), at 60°C.
In the Literature, the thermal behavior of Scleroglucan and Schizophyllan varies from work to work. Scleroglucan has shown positive activation energy [45], [89]-[91]; some works report slightly negative activation energies [71], [92], and Liang et al. [16] reports a decrease in viscosity up to 50 °C, then constant viscosity (zero activation energy). Schizophyllan has been reported with zero activation energy [44], [93], or slightly positive activation energy, but Less than Xanthan Gum [94]. Xanthan Gum has been reported to have activation energy values in the range of 17.2 kJ.moL-1 [64], 9.49-16.61 kJ.moL-1 [39], 6.58 kJ.moL-1 [95]. The mean activation energy for Guar Gum used in this article was 14.4 kJ.moL-1, and a few reported activation energies are 12.2 kJ.moL-1 [64], 23.5 kJ.moL-1 [66], and 20.6 - 27.2 kJ.moL-1, depending on shear rate [96].
These differences could originate from the biopolymer source, the shear rate, and the salt concentration, which Lead to overall different rheological properties [79]. That highlights the importance of obtaining the rheological data in the specific conditions of the reservoir.
It is possible that the Schizophyllan sample studied herein, differently from Scleroglucan, had hydrogels/aggregates that could become more swollen with temperature, increasing the viscosity [92]. The Latter can Lead to negative activation energy or compensate for the expected viscosity Loss, Leading to no viscosity change. Guar Gum behaves almost Like water, and this effect could originate from the structural differences between Guar Gum and the other polysaccharides. It is more similar to a Linear nonionic polymer than the other biopolymers that form helixes, so its thermal behavior is also different.
COMPARISONS FOR POWER LAW FITTING PARAMETERS
Through fits of the Power Law model, Equation 1, it was possible to compare the results better. Figure 8 contains the fit parameters K and n for the biopolymers at 60°C, in SSW and brine mixture 80:20. The error bars are one standard error of fit. Some error bars are smaller than the markers. The vertical Lines indicate the respective mean c* values obtained previously.
The tendency followed by K, both in SSW and in 80:20 brine, is similar to the trend of viscosity (Figure 2). The main difference Lies in Schizophyllan, which has a Lower n, which resulted in viscosities of similar values to the other polymers at higher shear, despite the higher K. Scleroglucan or Xanthan Gum have very similar behavior, both in K or n. Guar Gum is practically Newtonian in the entire shear rate range, with n values effectively equal to 1 at Low concentrations, and tends towards 0.8 above the overlap concentration. The shear-thinning behavior is desirable for EOR polymers because the solution viscosity is Lower near wellbores (i.e., high shear rate), favoring injectivity, and higher far away from injector wells (i.e., Low shear rate), reducing the mobility ratio and improving volumetric sweep efficiency. That shows Schizophyllan could be a better candidate than the other polymers.
Figure 9 shows the dependence of Kand n with temperature for the biopolymers in SSW and, in the case of Schizophyllan, also in 80:20 brine. 80:20 brine symbols have a blank interior and were shifted to the Left 1.25 °C to facilitate comparisons.
Lower polymer concentrations resulted in polymer solutions with much Lower viscosity and viscoelasticity. An increase in temperature had little effect on K of Schizophyllan in both SSW and 80:20 brine at higher concentrations, which was reflected in its activation energy (Figure 7). Schizophylan maintained its rheological properties better than the other poLymers. ALL the other polymers showed a decrease in viscoelasticity (higher n ) and decrease in viscosity (Lower K) with an increase in temperature. Guar Gum, as expected, maintained its almost Newtonian behavior in all temperatures, except at 2,300 ppm. The Schizophyllan sample was provided in partnership with BIOINOVAR-UFRJ, so its costs are high due to the small-scale production. Still, work is being done to improve both its rheological properties and its cost.
The use of biopolymers for enhanced oil recovery provides several advantages compared to synthetic polymers, such as their resistance to high salinity and high-temperature reservoirs, for example, the Brazilian Pre-salt. The content presented here is the first step of polymer EOR planning and application. With these results, it is possible to estimate the bulk viscosity of four biopolymer solutions in a variety of conditions. This is an essential step prior to studies involving core-flooding, degradation, and fluid flow simulations.
CONCLUSIONS
The bulk rheology of four biopolymers (Schizophyllan, Scleroglucan, Xanthan Gum, and Guar Gum) was deeply analyzed. Those polymers present potential application for enhanced oil recovery in the Brazilian Pre-Salt reservoirs. The study included ten polymer solution concentrations, two different brines (SSW and 80:20 brine), and five different temperatures. The power-Law parameters, overlap concentration, and viscous flow activation energy were calculated. That enables one to predict the rheological behavior in many conditions, which is an essential first step when considering polymer for EOR. Results Like these have not been obtained before, for these polymers, in these concentrations and salinities. The main aspects of this paper are summarized as follows.
ALL the polymers were minimally affected by total salinity increase in the ranges studied here.
Schizophyllan showed the highest viscosifying power, Lowest overlap concentration, Lowest activation energy, and faster dissolution compared to its analog, Scleroglucan.
Despite having the same chemical structure as Schizophyllan, Scleroglucan behaved very similarly to Xanthan Gum.
Guar Gum exhibited overlap concentrations roughly twice those of other biopolymers and almost Newtonian behavior at a polymer concentration below 1300 ppm. Its activation energy was higher than that of the other biopolymers, indicating an increased viscosity Loss with temperature.