Servicios Personalizados
Revista
Articulo
Indicadores
-
Citado por SciELO
-
Accesos
Links relacionados
-
Citado por Google
-
Similares en SciELO
-
Similares en Google
Compartir
Universitas Scientiarum
versión impresa ISSN 0122-7483
Univ. Sci. v.15 n.3 Bogotá sep./dic. 2010
Effect of nutrients and fermentation conditions on the production of biosurfactants using rhizobacteria isolated from fique plants
Efecto de los nutrientes y las condiciones de fermentación en la producción de biosurfactantes con rizobacterias aisladas de fique
Efeito dos nutrientes e as condições de fermentação na produção de biossurfactantes com rizobactérias isoladas da piteira
2 Centro de Investigaciones de Estudios Avanzados del Instituto Politécnico Nacional. México D.F. México.
3 Laboratorio de Control Biológico. Centro de Biotecnología y Bioindustria. Corporación Colombiana de Investigaciones Agropecuarias. Mosquera. Colombia.
Received: 10-09-2010; Accepted: 27-11-2010
Abstract
Objective. To isolate biosurfactant-producing microorganisms from the rhizosphere of fique and to select the best genus to evaluate the effect of nutritional and fermentation conditions on the production of rhamnolipids. Materials and methods. Rhizospheric soil was sampled in three areas of Cauca. The best genus was selected for the experimental designs (Plackett Burman and 22 factorial) and to find the production conditions for the growth kinetics at an Erlenmeyer flask scale. Results. Isolates from the rhizosphere of fique plants were from groups (or genera) of Bacillus, Pseudomonas and Actinomycetes, being Pseudomonas the more responsive in preliminary testing for emulsification. From the results of the experimental designs and the kinetics of production, we found that rhamnose synthesis associated with rhamnolipids (3.2 g/l) and emulsification (68% EC24) was significantly favored (p <0.0001) by cultivating an inoculum of 10% v/v of Pseudomonas fluorescens in a medium composed of: soybean oil 2% (v/v), K2HPO4 0.2% (w/v), yeast extract 0.4 g/l, NH4NO3 3.7 g/l, 1 ml trace elements (CoCl3 20 mg/l, H3BO330 mg/l, ZnSO410 mg/l, Cu2SO41 mg/l, Na2MoO43 mg/l, FeSO 410 mg/l MnSO42,6 mg/l) and pH 7.2. Conclusion. Of all the microbial genera isolated from the rhizosphere of fique, Pseudomonas fluorescens had the greatest potential in the production of biosurfactants of the rhamnolipids family.
Key words: Pseudomonas fluorescens, biosurfactant, rhamnose, emulsification index, soybean oil.
Resumen
Objetivo. Aislar microorganismos de la rizosfera de fique capaces de producir biosurfactantes y seleccionar el mejor género para evaluar el efecto de las condiciones nutricionales y de fermentación en la producción de ramnolípidos. Materiales y métodos. Se realizaron muestreos de suelos rizosféricos en tres zonas del Cauca. El mejor género fue seleccionado para realizar los diseños experimentales (Plackett Burman y factorial 22) y establecer las condiciones de producción para las cinéticas de crecimiento a escala de Erlemeyer. Resultados. Se aislaron bacterias del género Bacillus, Pseudomonas y del grupo Actinomycetes, siendo Pseudomonas el grupo con mayor respuesta en las pruebas preliminares de emulsificación. A partir de los resultados obtenidos en los diseños experimentales y cinéticas de producción, se estableció que la síntesis de ramnosa asociada con ramnolípidos (3,2 g/l) y la emulsificación (68% EC24) se favorecieron significativamente (p<0.0001) al cultivar un inoculo de 5% v/v de Pseudomonas fluorescens en un medio compuesto por: aceite de soya 2% (v/v), K2HPO4 0,2% (p/v), extracto de levadura 0,4 g/l, NH4NO33,7 g/l, 1 ml de elementos traza (CoCl320 mg/l, H3BO330 mg/l, ZnSO410 mg/l, Cu2SO4 1 mg/l, Na2MoO43 mg/l, FeSO410 mg/l MnSO42,6 mg/l) y pH 7.2. Conclusión. Se aislaron 3 géneros microbianos a partir de rizosfera de fique, siendo Pseudomonas fluorescens la bacteria con mayor potencial en la producción de biosurfactantes de la familia de los ramnolípidos.
Palabras clave: Pseudomonas fluorescens, biosurfactante, ramnosa, índice de emulsificación, aceite de soya.
Resumo
Objetivo. Isolar microorganismos da rizosfera da piteira capazes de produzir biossurfactantes, selecionar o melhor gênero para avaliar o efeito das condições nutricionais e de fermentação na produção de rhamnolipídeos. Materiais e métodos. Foram realizadas amostragem de solos rizosféricos em três áreas do Cauca. O melhor gênero foi selecionado para realizar desenhos experimentais (Plackett Burman e fatorial 22) e definir as condições de produção para as cinéticas em escala de erlenmeyer. Resultados. Foram isoladas bactérias do gênero Bacillus, Pseudomonas e grupo de Actinomycetes. As Pseudomonas foram o grupo com maior resposta nos testes preliminares de emulsificação. A partir dos resultados obtidos nos desenhos experimentais e cinéticas de produção foi estabelecido que a síntese de ramnose associados com rhamnolipídeos (3,2 g/l) e a emulsificação (68% EC24) foram favorecidos significativamente (p<0,0001) ao cultivar um inoculo de 5% v/ v de Pseudomonas fluorescens em um meio composto por: óleo de soja 2% (v/v), K2HPO40,2% (p/v), extrato de levedura 0,4 g/l, NH4NO3 3,7 g/l, 1 ml de oligoelementos (CoCl320 mg/l, H3BO330 mg/l, ZnSO410 mg/l, Cu2SO41 mg/l, Na2MoO43 mg/l, FeSO410 mg/l MnSO4 2,6 mg/l) e pH 7,2. Conclusão. Três gêneros microbianos foram isolados da rizosfera da piteira, sendo Pseudomonas fluorescens a bactéria com maior potencial na produção de biossurfactantes da família dos rhamnolipídeos.
Palavras-Chave: Pseudomonas fluorescens, biossurfactante, ramnose, o índice de emulsificação e óleo de soja.
Introducción
Biosurfactants are metabolites, generally secondary, that constitute a group of diverse compounds synthesized by a wide variety of microorganisms (bacteria, filamentous fungi and yeasts) (1-3).
Currently, biosurfactants do not compete with surfactants chemically synthesized due to the high production costs of the former (3, 4). This situation is related to inefficient processes, low productivity of microbial strains and the cost of raw materials. Therefore, for biosurfactants to achieve a significant market share, it is necessary to carry out research to increase the capacity to manage both the metabolism of the producing strains and the possibility of using low-cost substrates, and technological upgrading process production (5, 6). This will contribute to the discovery of a variety of biotensoactives, with a growing list of microorganisms producing biosurfactants and expanding the range of physical and chemical properties, leading to the discovery of compounds suitable for special applications. The growing interest in the potential applications of microbial surface active compounds is based on the different functional properties that include emulsification, demulsification, phase separation, wetting, foaming, solubilization and reduction of the viscosity of heavy oils. In consequence, there are many fields of industrial application where chemical surfactants can be replaced by biosurfactants, as diverse as agriculture, construction, food industries, textiles, paper and oils (7-9).
Under certain conditions many microorganisms can be induced to produce biosurfactants. In this sense, the study of the effect of different factors that influence the production of these compounds is of great importance. The types, quantities and qualities of biosurfactants are influenced by the nature of the substrate, pH conditions, temperature, agitation, concentration of Mg, P, Fe, and K ions, and biomass activity (10).
The synthesis of biosurfactants depends largely on the availability of carbon sources and the balance between carbon and other limiting nutrients (11). Production of biosurfactants also varies according to the microbial groups that produce them, generating compounds with different composition, and physical and chemical properties (11).
This study pays particular attention to the effect of the nutritional requirements and fermentation conditions for the production of extracellular biosurfactants by microorganisms, especially Pseudomonas fluorescens, isolated from the rhizosphere of fique plants in crop fields located in the department of Cauca in Colombia. It should also be noted that this new Pseudomonas isolate has the potential to be employed in the agricultural sector for its ability to produce substances that promote plant growth and biocontrol activity against fungal zoospores, which are responsible for causing diseases in fique plants and have generated important economic losses in the fique sector in Colombia.
Materials and methods
Sample collection from fique crop fields (Furcraea sp.)
Bacteria used in this study were isolated from the rhizosphere of fique plants of productive age in the municipalities of Silvia (2° 36' 50'' North and 76° 22' 58'' West, at 2,600 m, and 18°C), Totoró (2° 38' North and 2° 15' West, at 2,750 m, and 14°C), and the plateau of Popayan in the department of Cauca, Colombia.
Fifteen fique plant roots were collected from three different crop fields in each of the three localities assessed. Samples were placed in carefully labeled paper bags, geo-referenced, stored in closed containers at 4°C and transported in the storage conditions.
Isolation and preservation of strains
Samples were processed according to the methodology described by Kuklinsky-Sobral, et al. (12), separating the smaller roots and washing them with drinking water to eliminate the excess soil. Ten grams from each sample were then weighed and placed into 500 ml Erlemeyers containing 200 ml of 0.1% Tween 80 (v/v) and 25 g of glass beads of 0.5 cm in diameter. Each flask was placed at constant agitation at 150 rpm for 1 h.
All the samples were subdivided into two aliquots of 100 ml each. In the first aliquot, a heat shock was applied at 80°C for 10 minutes, and decimal dilutions were then carried out down to 10-9, and 0.1 ml of each dilution was inoculated on Luria Bertoni agar (LB). Plates were incubated at 28°C for 48 h following the protocol described by De Souza et al. (13)
In samples not subjected to heat treatment, the same dilutions were done and 0.1 ml of each dilution were inoculated on King B selective agar (kb) (14), Cetrimide agar supplemented with nalidixic acid (0.3 g/l) and incubated at 30°C for 48 hours, and oatmeal agar supplemented with 0.1% nystatin (v/v) and incubated at 22°C for 10 days.
Colonies that exhibited the characteristic morphology associated with the genus (Pseudomonas, Bacillus) or functional group (Actinomycetes) were again isolated in selective media through successive passes to obtain pure colonies. The isolates were coded and cryopreserved in 10% glycerol (v/v) at -70°C, accordingly to the protocol created by Corpoica's genebank of microorganisms used in biological control (Corpoica, 2005).
Semi-qualitative evidence of biosurfactant production
The initial selection of biosurfactant-producing bacteria was done by cultivating them on selective medium SW (15), which is composed of: NaNO3 1 g/l, KH2PO4 0.1 g/l, MgSO4.7H2O 0.1 g/l, CaCl2 0.1 g/l, yeast extract 0.2 g/l, glycerol 2% (v/v) (16), cetyltrimethylammonium bromide (CTAB) 0.2 g/l, and 0.005 g/l methylene blue, and pH 6.5. The test was carried out by introducing three plastic rings 5-mm in diameter and 10-mm high in the SW agar at a depth of 5 mm. In each ring, 50 ul were added of a cell suspension with a concentration of 108 cfu/ml of each organism grown in nutrient broth after centrifugation (30,100 x g for 15 min at 4°C). The agar plates were incubated at 30°C for 7 days. At the end of the incubation period, the blue halo of precipitation formed around each ring was measured using a digital caliper (EMC CE) (17).
Emulsification tests
Rhizobacteria were cultured on nutrient broth at 30°C for 24 hours. Subsequently, the biomass was recovered by centrifugation at 5,181 x g for 15 minutes and cells were washed twice with NaCl 0.85% (w/v). The biomass was then resuspended in 5 ml of NaCl 0.85% (w/v) and used to inoculate 45 ml of saline Davis minimal broth with an inoculum ratio of 1% (v/v) (17). The composition was: K2HPO4 5.23 g/l, KH2PO4 1.91 g/l, MgSO4 0.09 g/l, (NH4)2SO4 1 g/l, as well as 1 ml/l of trace elements solution (CoCl3 20 mg/l, H3BO3 30 mg/l, ZnSO4 10 mg/l, Cu2SO4 1 mg/l, Na2MoO4 3 mg/l, FeSO4 10 mg/l and MgSO4 2.6 mg/ l). Cultures were incubated at 30°C ± 2 at 150 rpm for 3 days.
The biomass was separated from the supernatant by centrifugation (30,100 x g for 15 min at 4°C), and the emulsifying activity was evaluated by adding 0.5 ml of cell-free supernatant, 0.5 ml of kerosene and 4 ml of distilled water to a 13 x 100 mm tube. Control 1 consisted of distilled water and kerosene, and control 2 consisted of distilled water, kerosene and Triton X-100 (100 mg/ml). Each tube was agitated in a vortex for 1 min and was left to stand for 24 hours. The height of the emulsification ring was then measured in mm and compared to that of the chemical emulsifier. The emulsification index was calculated using the following equation:

Additionally, we assessed the quality of the emulsification by verifying whether the ring formed was destabilized after vigorous shaking of the tubes (18).
Quantification of rhamnolipids
The indirect quantification of rhamnolipids was done using the orcinol method for quantifying rhamnose (19), by mixing 1 ml of diethyl ether with 333 ml of supernatant. The ether was fully evaporated in a desiccator, and 0.5 ml of sterile distilled water were added to the dry product. 100 ml of the dry and resuspended sample were taken to mix them with 900 ml of 0.19% orcinol m/v prepared in 53% H2SO4 (v/v). The mixture was heated to 80°C for 30 minutes, allowing it to stand until it reached a temperature of 17°C. The absorbance of the solution was measured at 426 nm and the concentration was calculated replacing the values in the standard curve of rhamnose (5-350 mg/l), using distilled water for comparison Tuleva et al., (17, 20).
Selection of culture conditions for biosurfactant production
Plackett Burman Design
We set a Plackett Burman experimental design to evaluate the effect of 10 factors at two levels on the production of the biosurfactant (table 1). Each experiment was carried out in triplicate, and values presented in the figures correspond to the average of the data with a standard deviation less than 15%. The emulsifying activity (EC24) (21, 17) and the extracellular concentration of rhamnolipids expressed as rhamnose (g/l) (19) Chandrasekaran & Bemiller, 1980 (17) Tuleva et al. 2002) were measured as dependent variables. To carry out the experiments, 250-ml Erlenmeyers were used with 45 ml of supplemented minimal medium and incubated according to each treatment and then taken to the operating condition to be evaluated. Each flask was inoculated with 5 ml of a suspension of microorganisms at a concentration of 9 x 106 cel/ml. The results were treated with an empirical model that relates the quantified responses to the factors evaluated and their respective levels. For a Plackett-Burman design, the first-order model was:

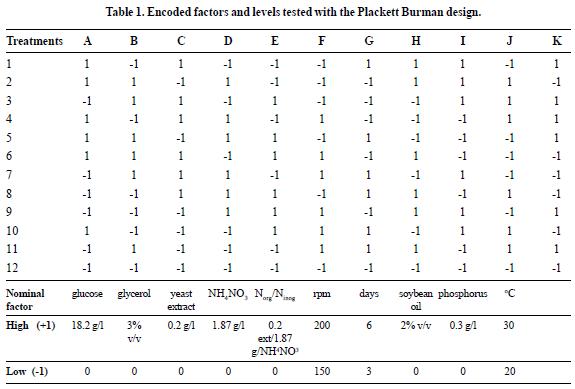
Where Y is the response (EC24 and Rhamnose), βo is the model intercept and βi is the estimated coefficient for each variable or factor Xi. Data analysis was carried out using SAS 9.0®.
22 Factorial Design
Based on the factors that were significant in the Plackett- Burman design, a 22 factorial design was additionally carried out to assess the effect of the percentage of inoculum X1 (1% and 10% v/v) and time X2 (6 and 13 days) on the emulsification and production of rhamnolipids (22, 23). The tests were performed in 250 ml Erlenmeyer flasks, containing 45 ml of saline Davis minimal medium (24) with soybean oil 2% (v/v) and a combination of nitrogen and organic/ inorganic nitrogen. Cultures were incubated at 30°C ± 2 and 150 rpm of constant shaking. The design produced a matrix of four treatments carried out in triplicate. Data analysis was performed using the programs SAS® 9.0 and Design Expert 6.0®. The results were treated with an empirical model that relates the quantified responses to the factors evaluated and the respective levels. For a design of two factors, the first-order model was:

Where: y corresponds to the dependent variable, bo is the intercept, b1, b2 are the linear coefficients and X1 and X2 are independent variables or factors. The dependent variables were emulsification (% EC24) and production of rhamnolipids (g/l).
Kinetics of growth and production of rhamnolipids
A preinoculum of Pseudomonas fluorescens was cultured in nutrient broth at 30°C for 24 hours. Subsequently, the biomass was recovered by centrifugation at 5,181 x g for 15 minutes and cells were washed twice with NaCl 0.85% (w/v). 60 ml of a cell suspension at 9x108 cel/ml was prepared and then added as the inoculum into 540 ml of MMS broth (Davis Minimal Medium Saline) at pH 7.2, maintaining constant temperature at 30°C and constant agitation at 150 rpm. Samples were taken every 2 h during the first day and every 6 hours until completing 15 days of processing. The following dependent variables were evaluated: biomass dry weight (25), EC24, rhamnose concentration, and pH. Additionally, we calculated parameters as: specific growth rate (μx), product yield on biomass (Yp/x) and productivity (26).
Biochemical characterization of isolates
The isolates that showed the highest biosurfactant activity were identified using the BIOLOGTM Automatic Identification System (Biolog, Inc. of Hayward). The results were compared with the database of Microlog software to determine the coefficient of similarity of previously studied genera in the identification system (27).
Results
Isolation of microorganisms
From surveys conducted in the municipalities, a total of 31 isolates were recovered, and bacteria of the genus Pseudomonas were identified as the most representative with 16 isolates (50%), followed by the genus Bacillus with 9 isolates (31%) and 6 isolates of the group Actinomycetes (19%).
Of the 31 isolates grown in agar SW, qualitative biosurfactant (rhamnolipid) production associated with the formation of a blue pigment around the colonies and the formation of yellow halos on the culture medium (figures 1, 2) was observed in 52% of the strains (15 Gram-negative bacilli and 1 Gram-positive bacilli). The diameter of the halos for Gram-negative bacilli was larger than 10.6 mm and the one of the Gram-positive bacilli was 8 mm at day 7of incubation. On the other hand, no growth or pigment production in the culture medium was detected with the other 15 strains.
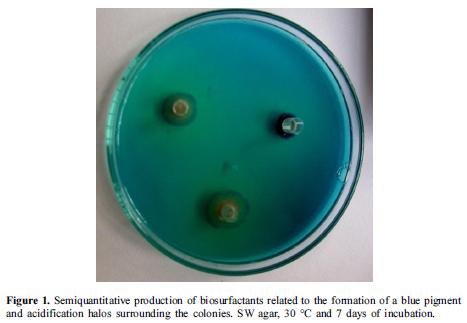
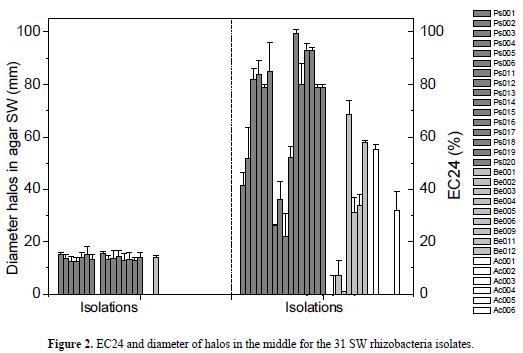
With respect to the preliminary tests of emulsification (figure 2), 41.9% of the isolates showed an EC24 greater than 50% with a white ring that did not destabilize when shaking vigorously the tubes after 24 h of incubation at 17° C. In 22.5% of the isolates, no emulsification of kerosene was found, and in 35.6% the EC24 was lower than 50%. According to the morphology distribution of bacilli that exceeded 50% of emulsification, 9 isolates were Gramnegative bacilli, 3 were Gram-positive bacilli and 1 was an Actinomycetes. Results obtained in the preliminary test showed that Gram-negative bacilli were the dominant group.
Selection of culture conditions for the production of biosurfactants
The selection of conditions for the biosurfactant production was made specifically for the genus Pseudomonas, choosing the best strain (Ps006) because this group was significantly better with respect to the production of biosurfactant than the other genera evaluated (p<0.0001).
The effect of medium components and operating conditions were evaluated using the Plackett Burman design. According to the analysis of variance with a confidence level of 95%, the factors that significantly affected the production of rhamnolipids and EC24 were the mixture of organic and inorganic nitrogen or factor E (p = 0.0071), and the addition of soybean oil at 2% (v/v) or factor H (p = 0.0081). Thus, first-order equations describing the models are:
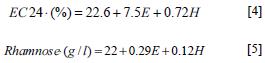
Under these conditions, the EC24 was 66% and 62% for treatments 8 and 9 with a rhamnose concentration of 1.9 g/l and 1.98 g/l, respectively, resulting in a production medium composed of: vegetable oil 2% (v/v), K2HPO4 0.2% (w/v), yeast extract 0.4 g/l, NH4NO3 3.7 g/l, 1 ml of trace elements (CoCl3 20 mg/l, 30 mg/l H3BO3, 10 mg/l ZnSO4, CuSO4 1 mg/l, Na2MoO4 3 mg/l, FeSO4 10 mg/l and MnSO4 2.6 mg/l) with pH 7.2 and operating conditions of 150 rpm, 6 days, and 30°C (Figure 3).
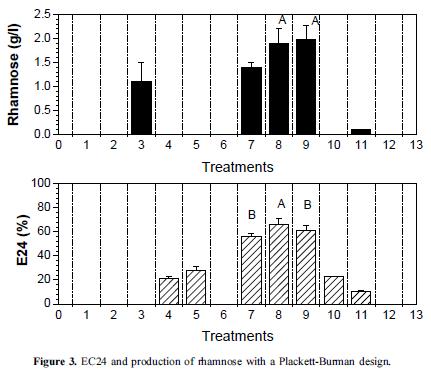
22 Factorial Design:
According to the results of the ANOVA, the interaction of X1 (time) and X2 (% inoculum) at the highest levels had a significant effect on the EC24 (p=0.0014), demonstrating that with an increase of the percentage of inoculum to 10% (v/v) and cultivating the bacteria for 13 days, the emulsification achieved was of 71%. Under these conditions there was an increase in the concentration of rhamnose (3.2 g/l), a value 41% higher than that obtained in the first experimental design. The first-order equations that allowed the prediction of the model were:

Additionally, 3D graphs are shown in which the interaction of X1 and X2 is most evident at the highest levels of each factor, confirming the results of the statistical analysis (Figures 4 and 5).
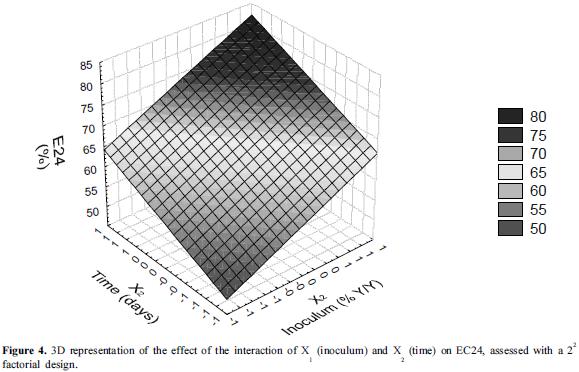
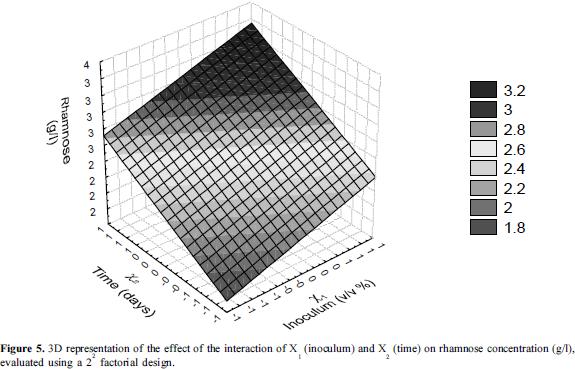
Kinetics of growth and production of rhamnolipids
Growth curves were prepared with Ps006 strain to evaluate the behavior of the parameters of interest as a function of time. Biosurfactant production was evident from the first hours of incubation, possibly behaving as a metabolite associated with output growth of 3.74 g/l at 10.6 days (254 h) of culture, with a productivity of 364 mg/l/d (Figure 6).
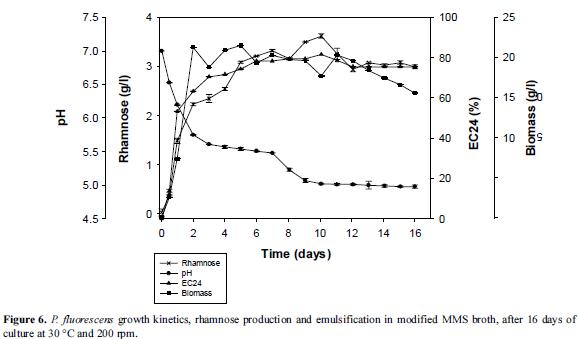
With regard to the formation of biomass as a function of time, the strain did not show an adaptation phase, and the logarithmic phase was extended to 2.2 days (52.8 hours), period during which the specific growth rate was 0.109 h-1 with time duplication of 6.3 h. Subsequently, the stationary phase was observed and possibly the death phase, as evidenced by a loss in viability when making surface counts (data not shown). Regarding product yields in biomass (Yp/x), the estimated value was 0.18 g/g. Figure 6 additionally shows the results for EC24, noting that the emulsifying activity increased proportionally with the biomass and the concentration of rhamnose, with a maximum of 81% after 10 days for processing. A slight decrease of 6% was subsequently showed, which remained constant until the end of the process, determining that at 16 days the final emulsification was 75%. With regard to the changes in pH values, a decrease was observed until 7 days with a value of 5.0.
Identification of selected isolates
All the Gram-negative bacilli found belong to the genus Pseudomonas and include several species such as Pseudomonas fluorescens, Pseudomonas aeruginosa and Pseudomonas maculicula. Ps006 isolate was identified as Pseudomonas fluorescens.
Discussion
The genera Bacillus and Pseudomonas were the dominant groups in the rhizosphere of fique considering all the municipalities. The isolates belonging to both genera surpassed 81% of recovery, and in turn, 50% of this 81% correspond to the genus Pseudomonas.
Gram-negative bacteria were the majority in the rhizosphere of fique, a finding consistent with that one reported by Kloepper (28), who also showed that within these bacteria, the group consisting of fluorescent Pseudomonas is prevalent. However, authors such as Atlas and Bartha (29) suggest the opposite, that it is the Gram-positive that predominate. It must be noted, though, that a determining factor of the rhizobacterial composition in plants is the selective pressure they exert through root exudates, and that the characteristics of these exudates are influenced by a large number of biotic and abiotic factors that alter the composition from one crop field to another (30).
The methodology described by Tuleva et al. (17) for qualitative determination of the production of glycolipids allowed efficient selection of the isolates with biosurfactant activity, which were examined using the formation of pigment around the colonies and dark halos as a qualitative indicator of biotensoactive production with similar results to those reported by Perfumo et al. (31). These authors evaluated the production of anionic glycolipids in culture plates with agar SW by P. aeruginosa AP02-1 isolated from hot springs and agro-industrial effluents with the formation of colonies surrounded by large halos of 2.0 cm in diameter, after 24 hours of incubation at 45°C.
Biosurfactant production was strongly dependent on the composition of the medium, affecting the efficiency of their production (23). In flask experiments, changing the carbon source affected both the emulsifying activity and the production of biosurfactant (32). The selected carbon source was soybean oil because it showed the highest rates of emulsification of rhamnose and, in addition, showed higher concentrations compared with other treatments. The production from soybean oil 2% had the highest concentrations when it was used as the sole source of carbon, and the most likely reason for this trend is the lipase activity of P. fluorescens, which facilitated the uptake of fatty acids contained in the soybean oil fractions. On the other hand, treatments in which glucose alone had mixed results or were low, these findings might be related to the presence of uronic acids, which could confer to the biosurfactant an increased resistance to acid hydrolysis with H2SO4 thus preventing the formation of rhamnose, or its concentration was underestimated by an interference in the technique as such (33, 34).
Literature shows that vegetable oils were more efficient substrates in the production of rhamnolipids by P. aeruginosa and P. fluorescens (Ps006) when compared with glucose and glycerol (35, 36). In this study, a similar trend was observed in the use of the three carbon sources tested, but vegetable oils (especially soybean oil) allowed a higher production of rhamnolipids by P. aeruginosa and P. fluorescens (Ps006). Similar results have been obtained by Stanguellini and Miller (37), Soberon-Chavez (38) and Santos et al. (39), evaluating P. fluorescens and P. aeruginosa with several carbon sources.
The ineffectiveness of hydrophilic substrates (for example, glucose and glycerol) in producing rhamnolipids seems reasonable since they are highly soluble and therefore there is no need for the cells to secrete biosurfactants to improve their solubility. In contrast, vegetable oils and hydrocarbons are hydrophobic substrates and this could trigger the formation of biosurfactants. However, hydrocarbons are generally less biodegradable than vegetable oils, leading to a poor cell growth, consequently to an ineffective production of rhamnolipids and a possible toxic effect on cells due to the nature of organic compounds (40, 41). On the other hand, vegetable oils can be hydrolyzed by the lipases of P. aeruginosa and P. fluorescens (42) to form fatty-acid long chains (FALC) composed essentially of 12-18 carbon atoms. The FALC can be further degraded by cellular oxidation to support growth or could become the precursor of lipids to promote the biosynthesis of rhamnolipids (42, 43). The results presented in Figure 3 show the dependence of the emulsion percentage on the concentration of rhamnolipids produced by P. aeruginosa and P. fluorescens in a medium containing 2% soybean oil.
Results presented in this study showed significant variations in the concentration of biosurfactants (rhamnolipids) when varying the carbon source (vegetable oils, glycerol and carbohydrates, or a combination) because there is a general trend to incorporate preferentially Pseudomonas 3HD monomers and 3HDd from carbohydrates compared to soybean oil. On the other hand, 3HHx and 3HO monomers seem to be generally incorporated preferentially from soybean oil compared to carbohydrates (44).
The production of rhamnolipids was observed in the presence of yeast extract and NH4NO3 at low concentrations, but was stimulated by the combination of the two sources and is inhibited by the absence of these compounds, which is consistent with the findings reported by Gibbs and Mulligan (45). This was demonstrated during the testing of the emulsifying activity and the concentrations of rhamnose; therefore, the best source of nitrogen for the production of rhamnolipids under the conditions tested in our study was the combination of yeast extract (0.4 g/l) and NH4NO3 (3.7 g/l). This was demonstrated by Deziel et al. (10) who also observed increased rhlAB gene expression with compounds containing NO3, only in the presence of NH4+.
Environmental factors and growth conditions such as pH, temperature, agitation, and oxygen availability also affect the production of biosurfactants because of their effects on cell growth (46, 47). The production of rhamnolipids in Pseudomonas spp. reaches its highest value with pHs between 5.0 and 5.5 and a sudden pH decrease below 7.0 (33).
We found that biosurfactants produced by selected rhizobacteria of the genus Pseudomonas are associated with cell growth. The yield of biosurfactant production was increased using experimental designs that allowed the improvement of the culture medium in order to favour a higher production of biomass. This association has been widely described in the production of biosurfactants for P. fluorescens (48).
When comparing the productivity results obtained with the P. fluorescens fique rhizospheres selected with those reported by Stanguellini and Miller (37), we found that both the changes to the environment and the selected strains favored the production and presented higher yields than those found by these authors, who reported a productivity of between 50 and 1000 (μg/ml) using P. fluorescens. By contrast, Kim et al. (4) reported a higher productivity than the one reported in our study, given that it was 260 mg/l/h when there were limitations in the nitrogen source for the strain P. aeruginosa NCIMB 11 599. The productivity values obtained in these initial fermentations in agitated Erlenmeyer flasks are comparable to those found in the scientific literature (37, 49), although it is necessary to optimize the culture medium and the conditions of operation.
Therefore, our present observations show that the timing and amount of inoculum have an effect on biosurfactant production in Pseudomonas and, in turn, the production is the highest in stationary phase cells, in accordance with the general idea found in the literature on this subject. On the other hand, there is a direct relationship between biosurfactant production and cell growth shown by a decrease in emulsifying activity when varying the composition of the medium and the culture conditions, in such a way that biosurfactant production can be associated with cell growth.
The biosurfactant obtained with P. fluorescens using soybean oil as a substrate may provide a promising approach for future research on its application as a compound with efficient biological activity for agricultural and environmental uses. Viewed altogether, our results demonstrate the utility of the regulation of physiological parameters and its effect on biosurfactant production and unique biochemical properties of these natural microbial metabolites.
An analysis of the structure, biological activity and kinetics of biosurfactant production would elucidate the potential of this biosurfactant produced by rhizobacteria; such studies are already taking place in our laboratory.
Conclusions
We successfully isolated a bacterial strain of P. fluorescens that is capable of effectively producing rhamnolipids from various carbon sources. Ps006 strain can degrade vegetable oils, and other carbon sources to produce biosurfactant.
The product has the potential to be applied in several processes. Among the carbon substrates evaluated, soybean oil was the most efficient for rhamnolipid production. At a concentration of 2%, soybean oil gave a maximum output level of 3.74 g/l and an optimal production rate of 364 mg/ l/d. Rhamnolipid production was optimal in batch cultures when the temperature and agitation were controlled at 30°C and 200 rpm, respectively. Rhamnolipids produced by P. fluorescens showed excellent emulsifying activity for kerosene (E24 = 78%).
With a factorial experimental design we determined the variation of independent variables on the production of rhamnolipids, the rate of emulsification, and the concentration of rhamnose. The present study demonstrated the potential that P. fluorescens isolated from fique rhizosfere has for the production of biosurfactants using soybean oil as an inductor.
Economy is the bottleneck for biotechnology products, often in the case of biosurfactants. Success on biosurfactant production from soybean oil in Colombia may allow the development of a less expensive process using low cost materials and renewable energy, and this would represent a decrease in production costs between 10% and 30%. There is a need for further research on the process of purification and concentration of the substance for commercial purposes of the rhamnolipid obtained.
Acknowledgments
This study was carried out as part of an international agreement of cooperation between the Research and Advanced Studies Center of the National Polytechnic Institute of Mexico (CINVESTAV-IPN) and the Pontificia Universidad Javeriana.
Financial support
Special thanks to the Colombian Ministry of Agriculture for financial support of the project number 2007J4761-492 and Pontificia Universidad Javeriana (Bogotá) for the technical and financial support provided for research internships.
Conflict of interest
The authors declare no conflict of interest regarding this study.
Referencias
1. Cameotra SS, Makkar RS. Recent applications of biosurfactants as biological and immunological molecules. Current Opinion Microbiology 2004; 7 (3): 15. [ Links ]
2. Nitschke M, Siddahartha GVAO, Contiero J. Rhamnolipid Surfactants: An Update on the General Aspects of these Remarkable Biomolecules. Biotechnology Progress 2005a; 21 (6): 1593-1600. [ Links ]
3. Banat IM, Franzetti A, Gandolfi I, Bestetti G, Martinotti MG, Fracchia L, Smyth TJ, Marchant R, Microbial biosurfactants production, applications and future potential. Applied Microbiology Biotechnology 2010; 87 (2):427-444. [ Links ]
4. Kim SH, Lim EJ, Lee SO, Lee JD, Lee TH. Purification and characterization of biosurfactants from Nocardia sp. L-417. Applied Biotechnology Biochemistry 2000; 31: 249-253. [ Links ]
5. Haba E, Espuny MJ, Busquets M, Manresa A. Screening and production of rhamnolipids by Pseudomonas aeruginosa 47T2 NCIB 40044 from waste frying oils, Journal Applied Environmental Microbiology 2000; 88 (3): 379-387. [ Links ]
6. Mukherjee S, Das P, and Sen R. Towards commercial production of microbial surfactants. Trends Biotechnology 2006; 24 (11): 509-515. [ Links ]
7. Costa SGVAO, Nitschke M, Haddad R, Eberlin M, Contiero J. Production of Pseudomonas aeruginosa LBI rhamnolipids following growth on Brazilian native oils. Process Biochemistry 2006; 41 (2): 483-488. [ Links ]
8. Makkar RS, Cameotra SS. An update on the use of unconventional substrates for biosurfactant production and their new applications. Applied Microbiology Biotechnology 2002; 58 (4): 428-434. [ Links ]
9. Rahman KSM, Rhaman TJ, McCLean S, Marchant R, Banat IM. Rhamnolipid biosurfactant production by strains of Pseudomonas aeruginosa using low cost raw materials. Biotechnology Progress 2002; 18 (6): 1277-1281. [ Links ]
10. Déziel E, Lépine F, Milot S, VillemurI R. RhlA is required for the production of a novel biosurfactant promoting swarming motility in Pseudomonas aeruginosa Ç3-(3-hydroxyalkanoykoxy) alkanoic acids (HAAs), the precursors of rhamnolipids. Microbiology 2003; 149, 2005-2013. [ Links ]
11. Prutti V, Cameotra SS. Effect of nutrients on optimal production of biosurfactants by Pseudomonas putidaa Gujarat oil field isolate, Journal Surfactant Detergent. 2003; 6: 6568. [ Links ]
12. Kuklinsky-Sobral WL, Araujo R, Mendes IO, Geraldi AA, Pizzirani-Kleiner JL. Isolation and characterization of soybean-associated bacteria and their potential for plant growth promotion, Environmental Microbiology 2004; 6 (12): 12441251. [ Links ]
13. De Souza AN, Martins ML. Isolation, properties, and kinetics of growth of a thermophilic Bacillus. Brazilian Journal Microbiology 2001; 32 (4): 271-275. [ Links ]
14. King EO, Ward MK, Raney DE. Two simple media for the demonstration of pyocyanin and fluorescin. Journal of Laboratory and Clinical Medicine 1954; 44: 301-307. [ Links ]
15. Siegmund I, Wagner F. New method for detecting rhamnolipids excreted by Pseudomonas species during growth on mineral agar. Biotechnoogy Technology 1991; 5 (4): 265-268. [ Links ]
16. Deziel E, Paqette G, Villemur R, Lepine F, Bisaillon JG. Biosurfactant production by a soil Pseudomonas strain growing on polycyclic aromatic hydrocarbons. Applied and Environmental Microbiology 1996; 62 (6): 1908-1912. [ Links ]
17. Tuleva BK, Ivanov GR, Christova N. Biosurfactant production by a new Pseudomonas putida strain. Verlag der Zeitschrift für Naturforschung. 2002; 57 (3-4): 356-360. [ Links ]
18. Christova N, Tuleva B, Lalchev Z, Jordanova A, Jordanov B. Rhamnolipid biosurfactants produced by Renibacterium salmoninarum 27BN during growth on n-hexadecane. Zeitschrift fuer Naturforschung. Section C Biosciences 2004; 59 (1-2): 7074 [ Links ]
19. Chandrasekaran EV, Bemiller JN. Constituint analysis of glycoaminoglycans, In: Methods in carbohydrate chemistry, v. III, Academic Press, New York, USA. 1980, 89-96 p. [ Links ]
20. Déziel E, Lépine F, Milot S, Villemur IR. Mass spectrometry monitoring of rhamnolipids form a growing culture of Pseudomonas aeruginosa strain 57RP. Biochemistry Biophycs Acta 2000; 1485 (2-3): 145-152. [ Links ]
21. Cooper DG, Goldberg BG. Surface active agents from two Bacillus species. Applied Environmental Microbiology 1987; 53 (2): 224-229. [ Links ]
22. Gizel CF, Priscilla FF, Marcio N, Maria ZC, "Factorial Design to Optimize Biosurfactant Production by Yarrowia lipolytica". Journal of Biomedicine and Biotechnology 2010; 2010:1-8 [ Links ]
23. Pattanathu KSM, Rahman GP, Vincent A, Zulfiqur A. 2010. Production of rhamnolipid biosurfactants by Pseudomonas aeruginosa DS10-129 in a microfluidic bioreactor. Biotechnology Applied Biochemistry 55 (1): 45-52. [ Links ]
24. Nielsen TH, Christophersen C, Anthoni U, Sørensen J. 1999. Viscosinamide, a new cyclic depsipeptide with surfactant and antifungal properties produced by Pseudomonas fluorescens and Pseudomonas fluorescens DR54. Journal Applied Microbiology 86 (10): 80-90. [ Links ]
25. Li E, Mira de Orduña R. A rapid method for the determination of microbial biomass by weight using a moisture analyser with an infrared heating source and an analytical balance. Letters in Applied Microbiology 2010; 50 (3):283-288. [ Links ]
26. Doran P. Principios de Ingeniería de los Bioprocesos. Editorial Acribia. Zaragoza, España. 1998, 468. p. [ Links ]
27. Bochner BR. Biolog: Modern Phenotypic Microbial Identification. Encyclopedia of Rapid Microbiological Methods, 2006; 2 (3): 55-73. [ Links ]
28. Kloepper JW, Rodriguez-Ubana R, Zehnder GW, Murphy J, Sikora E, Fernandez C. Plant root-bacterial interactions in biological control of soilborne diseases and potential extension to systemic and foliar diseases. Austral. Plant Patholology. 1999; 28: 21-26. [ Links ]
29. Atlas RM, Bartha R. "Ecología microbiana y Microbiología ambiental". Addison Wesley, Madrid. España. 2001. [ Links ]
30. Grayston SJ, Vaughan D, Jones D. Rhizosphere carbon flow in trees, in comparison with annual plants: the importance of root exudation and its impact on microbial activity and nutrient availability. Applied Soil Ecology 1996; 5 (1): 2956. [ Links ]
31. Perfumo A, Banat I, Canganella F, Marchant R. Rhamnolipid production by a novel thermophilic hydrocarbon-degrading Pseudomonas aeruginosa AP02-1. Applied Microbiology Biotechnology 2006; 72 (1): 132-138. [ Links ]
32. Perfumo A, Smyth TJP, Marchant R, Banat IM. Production and roles of biosurfactants and bioemulsifiers in accessing hydrophobic substrates. In: Timmis KN (ed) Handbook of hydrocarbon and lipid microbiology, vol. 2. Springer, Heidelberg Berlin,Germany. 2010ª. 15011512 p. [ Links ]
33. Guerra-Santos LH, Kapelli O, Fiechter A. Dependence of Pseudomonas aeruginosa continuous culture biosurfactant production on nutritional and environmental factors. Applied Microbiology Biotechnology 1986; 24 (6): 443-448. [ Links ]
34. Healy MG, Devine CM, Murphy R. Microbial production of biosurfactants. Resource Conserved Recycle 1996; 18 (1):41-57. [ Links ]
35. Reiling HE, Thanei-Wyss U, Guerra-Santos LH, Hirt R, Käppeli O, Fiechter A. Pilot plant production of rhamnolipid biosurfactant by Pseudomonas aeruginosa. Applied Environmental Microbiology 1986; 51 (5):985-989. [ Links ]
36. MataSandoval J, Karns C, Torrents A. Effect of nutritional and environmental conditions on the production and composition of rhamnolipids by Pseudomonas aeruginosa UG2. Microbiology Research, 2001; 155: 249-256. [ Links ]
37. Stanguellini ME. Miller RM. Biosurfactants. Their identity and potential efficacy in the biological control of zoosporic plant pathogens. Plant Disease 1997; 81: 4-12. [ Links ]
38. Soberón-Chavez G, Lépine F, Déziel E. Production of rhamnolipids by Pseudomonas aeruginosa. Applied Microbiology Biotechnology 2005b; 68 (6): 718-725. [ Links ]
39. Santos AS, Sampaio APW, Vasquez GS, Santa Anna LM, Freire DMG. Evaluation of different Carbon and Nitrogen Sources in Production of Rhamnolipids by a Strain of Pseudomonas aeruginosa. Applied Biochemistry Biotechnology 2002; 98 (1-9): 1025-1034. [ Links ]
40. Shabtai J, Wang DIC. Production of emulsion in a fermentation process using soy bean oil in a carbon nitrogen coordinated feed. Biotechnolology Bioengineering 1990; 35 (8): 753-765. [ Links ]
41. Chayabutra C, WU J, JU LK. Rhamnolipid production by Pseudomonas aeruginosa under denitrification: effects of limiting nutrients and carbon substrates. Biotechnology Bioengineering 2001; 72 (1):25-33. [ Links ]
43. Lang S, Wullbrandt D. Rhamnose lipids-biosynthesis. Microbial production and application potential. Applied Microbiology Biotechnology 1999; 51 (1):22-32. [ Links ]
44. Soberon-Chavez G, Aguirre-Ramirez M. Is Pseudomonas aeruginosa Only "Sensing Quorum"? Critical Reviews in Microbiology 2005a; 31 (3): 171-1782. [ Links ]
45. Gibbs BF, Mulligan CN. Factors influencing the economics of biosurfactants. Biosurfactants, Production, Properties, Applications. Marcel Dekker Kosaric, N. (Ed.), New York, United States. 1993, 329371 p. [ Links ]
46. Desai, J. D., Banat, I. M. Microbial production of surfactants and their commercial potential. Microbiology and Molecular Biology Reviews 1997; 61 (1): 47-64. [ Links ]
47. Kosaric N, Gray NCC, Cairns WL. Microbial emulsifiers and de-emulsifiers, In Rehm HJ, Reed G. (ed.), Biotechnology, vol. 3. Verlag Chemie, Deerfield Beach, Florida, United States. 1983, 575592. p. [ Links ]
48. Desai JD, Desai AJ. Production of biosurfactants. In Kosaric N. (ed.), Biosurfactans. Marcel Dekker, New York, United States. 1993, 65-97p. [ Links ]
49. Pruthi V, Cameotra SS. Effect of nutrients on optimal production of biosurfactants by Pseudomonas putida a Gujarat oil field isolate, Journal of Surfactants and Detergents 2003; 6 (1): 6568. [ Links ]