Introduction
Biofilm is the most common system of microbial organization. It is formed by one or several microorganisms attached to a living or inert surface. Biofilms possess functional characteristics and complex structures. In a biofilm, microorganisms are wrapped in an extra-cellular matrix made of polysaccharides that display a tulip or mushroom shape with the presence of internal water channels. Additionally, biofilms form interaction stages between microorganisms and host cells creating a metabolic community that contributes in a complex manner to the microorganism’s survival.
Biofilm formation confers bacteria phenotypic characteristics that are not seen in the planktonic state. Under this state microorganisms can change their individual, genetic and physiological characteristics. This allows microorganisms not only to achieve a higher survival rate, but also to acquire virulence characteristics of other microorganisms present in the biofilm. This is clinically significant since the host’s defense system and other mechanisms including chemical or mechanical treatments are not sufficient to eradicate infections that become persistent 1)-(5.
Dental pulp is a structure composed of soft connective tissue, blood vessels, and nerves located in root canals. They are surrounded by enamel and dentin that act as a barrier to avoid microorganism contamination. However, when teeth hard tissues are destroyed by dental or periodontal infections and trauma, microorganisms can penetrate through the pulp. This causes inflammation followed by pulp and periapical tissue necrosis. Therefore, to preserve the tooth, it is necessary to perform endodontic treatment, removing microorganisms from the root canal. To this end, chemo-mechanical debridement of dentin walls must be performed with sealing to prevent re-contamination. Nevertheless, sometimes a correctly performed endodontic treatment can fail due to apical radicular dentinal tubule microorganism invasion capable of adhering and surviving in the root canal by biofilm formation 1), (3), (6)-(8.
Endodontic pathogenic microorganisms are defined by their capacity to induce tissue destruction in apical periodontitis. Thus, they must have efficient adaptive mechanisms allowing them to survive such environment including pH regulation, tolerance to temperature changes, equilibrated substrate utilization, facultative oxygen management, adhesion protein expression, biofilm formation, and the ability to interact with other microorganisms. These factors endow bacteria with diverse phenotypic features that enable them to survive in hostile environments and make them more virulent 1), (3), (9)-(10.
Although many microorganisms that cause persistent endodontic infections have been identified, most studies have focused on evaluating new antimicrobial irrigant effectiveness and different preparation techniques used to eliminate biofilm formed only by one microorganism, mainly Enterococcus faecais (EF). However, considering polymicrobial endodontic re-infection, recent studies have employed multispecies biofilm formed by two or more microorganisms including Enterococcus, Streptococcus sp., Candida albicans and Staphylococcus sp.11)-(14
Enterococcus faecais is described as the primary cause of persistent endodontic infections. It has often been detected in root canal system infections, when endodontic treatment fails. Enterococcus faecais has a high capacity to survive and grow in microenvironments with the presence of calcium hydroxide resulting in colonization and root canal infection. 15)-(17. Another species related to dental intracanal biofilm formation is Sthaphylococcus aureus (SA). This is a facultative anaerobic, immobile, non- sporulated, Gram-positive coccus, which is source of periodontal, periapical, and endodontic infections 18)-(19.
Even though fungi are not frequently detected in primary endodontic infections, its presence is related to endodontically filled teeth with peri-radicular lesions resistant to root canal treatment. In addition, they can be the result of disease management contamination. It has been reported that yeasts like Candida albicans (CA) have the ability to grow as mono-infections and invade dentinal tubules through pseudo-mycelia. Moreover, is also commonly found in some cases of persistent endodontic disease 18)-(20.
A variety of models have been used for biofilm formation, but few studies have used natural teeth, simulating the clinical reality taking into account root canal anatomical complexity. Therefore, the objective of this study was to standardize optimal in litro conditions for endodontic biofilm formation. These included: atmosphere, incubation period, and detection. Three microorganisms were evaluated on human premolar root canals: Enterococcus faecalis, Staphylococcus aureus, and Candida albicans. This represents a starting point for new projects to characterize bacterial root canal behavior, and identify the most appropriate method for elimination.
Materials and Methods
This study was carried out in accordance with the recommendations of the Research and Ethics Committee of Dental School at Pontificia Universidad Javeriana. All subjects gave written informed consent in accordance with the Declaration of Helsinki. The present study used 128 lower premolars that were extracted for orthodontic purposes and had no periodontal disease, previous endodontic treatment or restorations
Dental crowns were removed and root length was standardized to 16 mm, followed by 5.25 % (v/v) sodium hypochlorite (NaOCl) immersion for 30 minutes, to remove remaining organic tissue. Corono-apical technique 1 mm below the apical foramen was used for root canal preparation employing a K-type file # 50. In addition, 3 ml of 5.25 % NaOCl was used for irrigation between each file. Subsequently, root canals were dried and soaked in 17 % EDTA for 3 minutes for dentin detritus removal. Each premolar was then sterilized in autoclave at 120 °C.
The experimental system was designed by placing a root into a sterile 1.5 ml polypropylene tube (Eppendorf, Hauppauge, NY USA) cut off at the distal end, sealed with cyanoacrylate adhesive and epoxy resin. Moreover, it was covered with two coats of nail polish. Each tube-root system was sterilized in 5 % NaOCl for 30 minutes and then washed with sterilized water for 30 minutes.
Enterococcus faecais 9212, Staphylococcus aureus 29213, and Candida albicans 10231 ATCC strains were used. Selective media Enterocult agar was used for Enterococcus faecais microbiological confirmation. Colonies with morphological and stain characteristics compatible with the genus were identified using biochemical tests such as catalase, PYR, 6.5 % NaCl, hemolysis on blood agar, Inulin, Mannitol, and Bile esculin agar followed by semiautomatic Micro Scan system confirmation (Dade Behring Microscan, West Sacramnento CA, EEUU).
Staphylococcus aureus was confirmed by Trypticase Soy Agar primary isolation. Colonies with morpho-tinctorial characteristics (Gram Staining) of the Staphylococcus genus were selected from this media. Subsequently, biochemical identification tests such as catalase, DNAase agar, Mannitol were carried out, followed by Micro Scan system confirmation 21.
Candida albicans confirmation was first carried-out by primary Sabourad agar isolation. Gram staining was used to analyze typical conidia and blastoconidia. In addition, it was grown on CHROMagar Candida Medium (CHROMagar, Microbiology, France). Moreover, biochemical identification tests such as urease, auxonogram for carbohydrate assimilation and filamentation at 42 °C were performed. Candida spp. manually identified colonies were confirmed using Micro Scan automated panel.
Each microorganism was proliferated in brain heart infusion broth (BHI) at 37 °C for 24 h. Samples were centrifuged and re-suspended in an isotonic saline buffer supplemented with BHI broth until reaching an approximate final concentration of 3 x 10 8 cells/mL, corresponding to an absorbance of 0.200 from turbidity tube No. 1 of McFarland's nephelometer.
128 experimental systems were incubated in a moist chamber at 37 °C under two different atmospheres: 64 in aerophilic atmosphere and 64 in microaerophilic (10 % CO2). From these, eight groups with eight experimental systems were inoculated in the following manner: 1 Enterococcus faecalis (EF), 2 Staphylococcus aureus (SA), 3 Candida albicans (CA), 4 Negative control (NC), 5 (EF + SA), 6 (EF + CA), 7 (SA + CA), 8 (EF + SA + CA). 50 µl from each ATCC strain were inoculated in each experimental system. All experiments were carried-out in duplicates, and evaluations were performed after 15, 30, 45 and 60 days.
Microbial growth was confirmed introducing sterile absorbent paper strips for 2 - 3 minutes into root canals of all experimental systems. Each paper strip was submerged in 7 mL BHI broth and it was incubated at 37 °C for 24 h. Microbial growth was evaluated using three basic parameters: culture media turbidity, Gram staining, and biochemical identification. Negative controls were evaluated under the same experimental conditions.
Biofilm formation was confirmed by electron microscopy. To this end, experimental systems were sectioned by cryo-fracture with liquid nitrogen and subsequently subjected to gold metallography preparation for Scanning Electron Microscope (SEM) analysis JEOL, Peaboy MA USA Model JSM 6490-LV).
For biofilm formation evaluation, the apical portion of the crown root cut was observed and two micrographs for each sample were recorded: one performed on the coronal third and the other on the apical third at 5.000 X (1µm) and 20.000 X (5 µm) magnification, respectively. All samples were analyzed including controls.
Results
Optimal Period of Time for Biofilm Formation: After having conducted microbiological evaluation and biofilm detection by SEM on each tooth, it was evidenced biofilm formation was observed primarily on surface areas after 15 days: in aerophilic media and microaerophilic media. In contrast, on apical zones adherence and invasion phenomena were detected, but no biofilm formation was recorded.
Microbial functional interactions within extracellular polymeric matrix on the apical third of the root canal were noted. A complete surface-associated growth was obtained after 30 days of incubation (Figure 1). Subsequent evaluations made it possible to observe that these conditions remained up to 45 days. Therefore, no further SEM analyses were performed after 45 and 60 days.
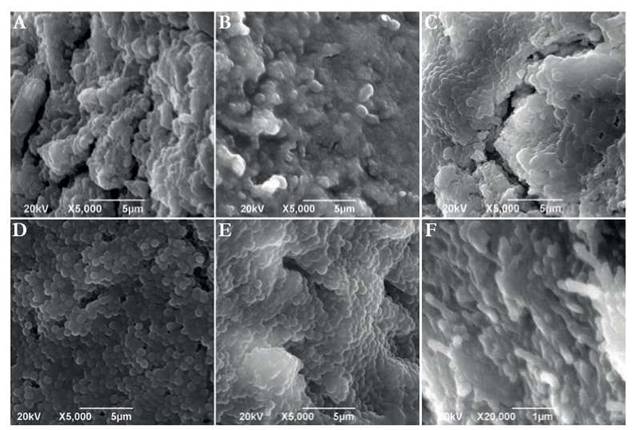
Fig. 1 Comparison between cultures in aerophilic media (top micrographs) and microaerophilic media (bottom micrographs) in the apical tooth portion after 30 days of incubation. (A) Micrograph of E. faecalis + S. aureus + O2 culture. (B) Micrograph of E. faecalis + C. albicans +O2 culture. (C) Micrograph of E. faecalis + C. albicans + S. aureus + O2 culture. (D) Micrograph of E.faecalis + S. aureus + CO2 culture. (E) Micrograph of E. faecalis + C. albicans + CO2 culture. (F) Micrograph of E. faecalis + C. albicans + S. aureus + CO2 culture. 5,000 X. Scale bar 5 µm.
Once the effect of oxygen as a factor on biofilm development was analyzed, it was evidenced under anaerobic atmosphere biofilm developed on the surface area after 15 days. Although bacterial aggregates on dentin surface at the coronal third of the root was observed, dentinal tubules were still visible. However, at the apical level no bacterial growth was detected for any of the analyzed samples (Figure 2). Never the less, bacteria presented a better development in terms of growth as biofilm at 10 % CO2. Under these conditions, a better bacterial aggregation on the dentin surface without presence of dentinal tubules on the coronal portion was observed (Figure 3). Under CO2 atmosphere, the only microbial combination capable of growing on the apical third during this period of time was CA and EF. This was evident taking into account the aforementioned recovered CFU.
On the dentinal surface a better formed biofilm and well developed structures were observed along the entire canal. However, regarding aerophilic cultures, it was possible to evidence the presence of some dentinal tubules on apical portions. In contrast, microaerophilic cultures seemed to grow even better on the apical fraction.
Biofilm Maturity: Scanning electron microscopy results evidenced microorganisms surrounded by glycocalyx and adhered to a hard surface. The matrix formed by the combination of EF, SA, and CA under an aerophilic atmosphere and incubated for 15 days demonstrated greater bacterial growth and biofilm behavior, than that observed in the anaerobic environment.
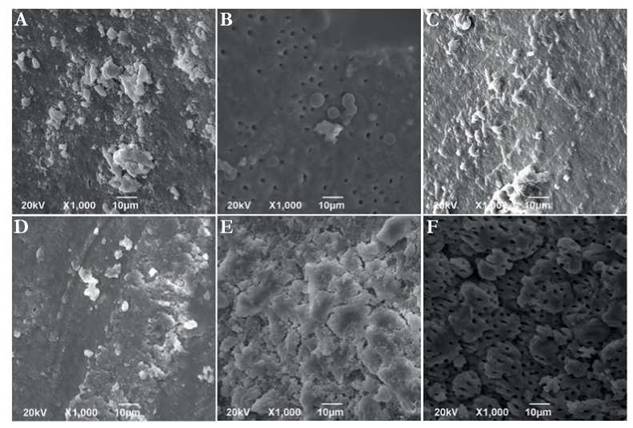
Fig. 2 SEM Micrographs on the apical parts and teeth crown performed after 15 days of culture. (A) Micrograph of E. faecalis culture. (B) Micrograph of C. albicans culture. (C) Micrograph of E. faecalis + C. albicans culture. (D) Micrograph of C. albicans + S. aureus culture. (E) Micrograph of E. faecalis + C. albicans+ S. aureus culture. (F) Micrograph of apical portion where no growth was observed. 1,000 X. Scale bar 10 pm.
Identification and Detection of Microbial Morphotypes: Gram staining microscopic observations and solid culture media colony macroscopic characteristics made it possible to select colonies for each of the microorganisms used in the experimental phase. Such colonies were identified through manual and Micro Scan systems getting a concordance rate of 100 % with respect to the inoculated microorganism in each root canaliculi. Isolation and identification were useful to simultaneously evaluate culture purity after inoculating a tooth. Results revealed 100 % pure isolation. Furthermore, as established by Gram staining negative controls did not have microorganisms, thus cultures were 100 % negative for bacterial growth.
Discussion
This study evaluated three factors possibly affecting root canal biofilm growth and development, namely: O2 concentration, microbiota etiology in a timely associated processes, and species interaction. For this latter one E. faecalis (EF), S. aureus (SA), and C. albicans (CA) were evaluated for adherence and virulence features.
After 15 days of incubation at 37 °C, it was observed for both atmospheres (O2 and 10 % CO2) that microorganisms formed an exopolysaccharide matrix, with initiation of surface-adhesion and cell division. Proliferation grew exponentially after 30 days in reduced oxygen atmosphere. SEM revealed a consolidated and mature biofilm with deep penetration of the microorganism into the dentinal tubules. As a case in point it has been proposed for EF that biofilm formation is a response mechanism against adverse environmental conditions; displaying important clonal variation in their ability to form this biofilm 22.
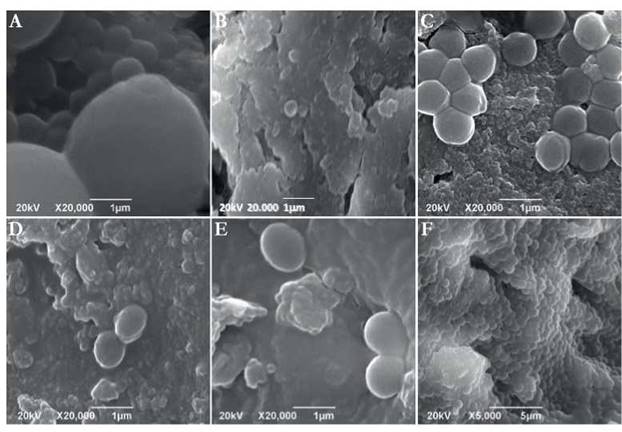
Fig. 3 SEM micrographs of crown and apical portion of teeth performed after 15 days of culture under microaerophilic media. (A) Micrograph of C. albicans culture. (B) Micrograph of E. faecalis + S. aureus culture. (C) Micrograph of E. faecalis + C. albicans culture. (D) Micrograph of C. albicans + S. aureus culture. (E) Micrograph of E. faecalis + C. albicans + S. aureus culture. (F) Micrograph of E. faecalis + C. albicans culture on the apical portion. 20,000 X. Scale bar 1 pm.
Similary to our study other authors detected that EF formed bacterial aggregations together with an exopolysaccharide layer adhered to root canal walls at week two. Furthermore, at week four it was able to develop co-aggregates covering the dentin surface 23),(24.
Conditions of aerobiosis, microaerophilic, and anaerobic atmospheres on paper or nylon matrixes and direct cultures among others have been evaluated. The authors recognize that reduced molecular oxygen at the root canal level benefits biofilm formation, and can increase antimicrobial agent resistance. Therefore, it is important to standardize optimal O2 conditions for an in vitro biofilm model: this represents a great advancement to evaluate antimicrobial sensitivity and environmental resistance to microorganisms, especially EF 25),(26.
The current study established that microorganisms remained viable during the whole experiment. Additionally, pure strains were isolated free of any contamination. Individual count for every microorganism evaluated made it possible to establish a growth pattern resembling the classical microbial growth curve. Initially, a lag phase did not exceed 1 x 102 CFU/mL. Subsequently, log phase counts ranged between 1 x 104 CFU/mL to 1 x 107 CFU/mL. Last, while in the stationary phase and decline phase microbial population decreased to 1 x 102 CFU/mL - 1 x 104 CFU/mL. In systems where two or three microorganisms were combined, a greater growth was observed, when compared with only one species interaction. It is likely under more than one species microorganisms can exchange substrates and nutrients. When comparing SA to EF or CA, it was observed that the initial growth was slow and required interaction with other microorganisms to improve development and maturity. CA and EF have the ability to grow as mono-infections because they are microorganisms with multiple pathogenicity factors, such as adhesion proteases, resistance to high temperature ranges, tolerance to wide pH ranges; additionally, they were able to survive nutrient shortage. These features demonstrate that they are aggressive microorganisms, not depending on bacterial interactions for their development and growth 27),(28.
A mature biofilm and rapid growth was observed by SEM analysis for both aerophilic and microaerophilic cultures when three microorganisms interacted in a same root canal. This suggests bacteria colonized a space and performed a specific function. Microorganisms in a sessile state possess mechanisms to protect themselves against the environment (immune system, antibiotics, or disinfectants). Furthermore, it is an efficient system to capture nutrients, and cross feeding strategy between species can take place. In addition, toxic metabolites can be removed, last develop an appropriate environment with physicochemical conditions for growth 4.
Although an in vivo biofilm formation occurs spontaneously and requires a low rate of nutrients, the development of each of its stages and required generation time differs considerably from an in vitro scenario. Experimental models do not involve the totality of microorganisms. There is no evidence of active supply of external nutrients or intervention of host factors during the process. Furthermore, some stages of biofilm development such as dispersion, absorption and fixation depend on quality and type of adhesive material used in the experimental model. Hence, data from biofilm formation in vitro cannot be explored at the same level of in vivo biofilm formation 4.
This standard model revealed the optimal period of time for immature biofilm establishment on surfaces occurred after 15 days. In contrast, mature biofilm formation including apical third of root canal invasion occurred after 30 days. Experimental designs intended to standardize in vitro biofilm formation methods have contradicting results with studies reporting growth ranging from three days to even 60 days 26),(29.
SEM data demonstrated biofilms grew more rapidly on the apical region due to CO2 presence. This was mainly the case for EF and the combination of three microorganisms (EF + SA + CA). CA and SA independent cultures did not form any mature or compact film.
Additionally, differences in time may be related to various experimental factors such as biofilm growth on a solid-liquid medium. In addition, fluid medium irrigation velocity may influence nutrient transport, toxic molecule elimination, and detachment of microorganisms. Likewise, experimental models do not possess substrates that are supplied by the various interrelations between other bacteria Fusobacterium, Eubacterium, Bacteroides, Capnocytophaga, Eikenella, Streptococcus, Actinomyces, Campylobacter, Porphyromonas such as hemin, succinate, ammonia, formate, acetate, menadione among others. These substrates influence the rate of adaptation to the biological niche and also the scale of biofilm formation 30.
The time needed for biofilm formation on an infected root canal depends on the use of disinfectant and alkalizing substances such as calcium hydroxide, presence or absence of exudate from the root canal, the type of microorganisms involved, and its location on the root canal. These variables are not considered in in vitro models 31),(32.
Other studies have contributed to evidence EF ability to colonize mechanically and chemically treated root canals. EF has the capacity to adhere on the dentine surface to collagen, where protease synthesized by bacteria and collagen binding protein (Ace) are probably related to the process of bacterial adhesion. Thus, allowing bacteria to colonize the root canal 33.
From this experimental design it was possible to infer that there is no unique biofilm model. Therefore, individual contribution of each variable from the proposed model should be analyzed. This is necessary in order to determine the adequate environmental conditions for development and modifications of biofilms formed on the root canal, as well as their capacity of penetration into the dentinal tubules.
Conclusions
The in vitro biofilm model formed by micro-organisms such as EF, CA and SA grew best in microaerophilic atmospheres of 10 % CO2 at 37 °C Biofilm maturation and development was attained on the entire root surface after 30 days of incubation.
Bacteria inoculated as a group had a much more rapid growth in comparison with individual inoculations. This can be attributed to synergistic and additive interactions among them.
Understanding the capacity of these microorganisms, individually and collectively to form biofilm within the root canal fosters a novel approach to the physiopathology of the infectious process. Furthermore it provides a standardized in vitro model that will be useful to evaluate the antimicrobial effect of irrigants, chelating and antiseptic solutions on these biofilms.