Introduction
The Orchidaceae is the largest vascular plant family in Ecuador, with nearly 4000 species, and is of economic importance for this country due to its significant share in the international ornamental plant market (Simpson 2006).
Cattleya is one of the most noted genera of the family, for its popularity and richness of colors (Krapiec 2003). One native representative species of the genus is Cattleya maxima (Dodson & Escobar 2005). Unfortunately, an intense specimen harvest, linked to habitat loss and deforestation threatens most native and endemic Ecuadorian orchid species (Dodson 2005). To counter this situation, ex situ clonal propagation methodologies for orchids are being developed (Cuoco & Cronan 2009). For instance, several techniques of clonal propagation have been developed for the genus Cattleya (reviewed by Arditti 2008; Krapiec 2003). Transgenesis is a powerful tool to aid the success of clonal propagation of orchids. Pioneering work in the early eighties (Fraley et al. 1983) paved the way for the production of transgenic plants engineered for several purposes (Deo etal. 2010). Currently, successful orchid transformation protocols rely on an efficient regeneration procedure based on Somatic Embryogenesis (SE) and on culture conditions favoring the formation of the specific structures called Protocorm-Like-Bodies (PLBs), as a prerequisite for optimal clonal propagation (Young et al 2000, Texeira et al 2006, Cueva et al 2014).
During SE, differential expression of several genes takes place (Chugh & Paramjit 2002). However, only few of the differentially expressed genes are considered embryogenesis-specific (Ikeda et al. 2003). The Somatic Embryogenesis Receptor-like Kinase (SERK) gene, is one of such genes expressed during SE as well as in zygotic embryogenesis. SERK expression is characteristic of embryo-forming masses in induced carrot suspension cultures (Schmidt et al. 1997). Homologues of carrot SERK have been described in different species and are encoded by small gene families of 1-6 members (described in Cueva et al 2012). In rice Oryza sativa, two SERK genes have been identified, OsSERK1 and OsSERK2 (Ito et al. 2005). Presumably, by modulating the expression of genes such as SERK during SE, the success of clonal propagation procedures could be improved. However, for orchids, and particularly for those of the genus Cattleya, reaching to this point first requires the development of the appropriate transgenesis toolkit.
Transgenic orchid production has been reported only for a few orchid genera (Men et al. 2003). Agrobacterium tumefaciens-mediated and microprojectile particle bombardment (biolistic protocol) have been the most successfully used methods for orchid transgenesis (Deo et al. 2010, Texeira da Silva et al. 2011). The biolistic method is thus far the most employed approach for orchid transformation, as in the genera Dendrobium (Men et al. 2003, Kuehnle & Sugii 1992, Chia et al. 1994, Yu et al. 1999, Tee et al. 2003), Phalaenopsis, Cymbidium (Yang et al. 1999), Cattleya, Brassia, and Doritaenopsis (Knapp et al. 2000). The Agrobacterium-mediated method has been used for Dendrobium (Wasana et al. 2015, Men et al. 2003, Yu et al. 2001), Phalaenopsis (Belarmino & Mii 2000, Chai et al. 2002, Mishiba etal. 2005, Sjahril & Mii 2006), Cymbidium (Chen & Chang 2002, Chin etal. 2007), Oncidium (Liau etal. 2003), Vanda (Pavallekoodi etal. 2014), Erycina (Lee et al. 2015), and Cattleya (Zhang et al. 2010). Compared to the biolistic method, Agrobacterium-mediated transformation offers the following advantages: delivery of a lower transgene copy number, lower level of transgene rearrangement, transfer of relatively large segments of DNA, and no special equipment requirements (Hei etal. 1994, Cheng etal. 1997). The Agrobacterium method proved to be efficient for most dicotyledonous species, but it appears to be less suited for monocots. The genus Cattleya was found to be recalcitrant to Agrobacterium-mediated transformation. Only one successful application of the method has been reported for a commercial Cattleya variety (CM2450) (Zhang etal. 2010).
Selection of transformants relies on the use of the two types of markers: reporter genes and selectable markers. The most commonly used reporter genes for orchid transformation are: β-glucuronidase (GUS), the anthocyanin pigmentation gene, the firefly luciferase gene, and the Green Fluorescent Protein (GFP) gene. As for selectable markers, several antibiotic, or herbicide resistance genes have been utilized, including neomicyn phosphotransferase (nptII), hygromycin phosphotransferase (hpt), and phosphinotricinacetyltransferase (bar) (Suwanaketchanatit etal. 2007). However, orchid cells are normally poorly sensitive to most selective agents. For instance, a high concentration of gentamicin and kanamycin (600 mg l-1) was used to eliminate untransformed cells and plants of various Dendrobium species (Chia et al. 1994). Instead, hygromycin (Hyg) was reported to be a reliable selective agent for the transformation of orchids (Men et al. 2003, Belarmino & Mii 2000, Chai et al. 2002, Liau et al. 2003, You et al. 2003, Liao et al. 2004). In this study, an Agrobacterium-mediated protocol for the transformation of C. maxima was established as a mean to promote the overexpression of the Os-SERK gene on SE in this orchid species.
Materials and Methods
Plant Material
Protocorms of C. maxima were obtained from seeds germinated in vitro on half strength Murashige & Skoog (MS) medium containing 20 g/L of sucrose and grown at 57 µmol m-2 s-1 photon flux density (fluorescent lamps of 40 W), at 21 ± 2 °C with a 12 h photoperiod.
Plasmid Construction
The well-characterized monocot SERK1 gene from rice (OsSERK1) (Kikuchi et al. 2003) was used in this study. The complete OsSERK1 cDNA (Clone, PS-JO33117E16-National Institute of Agricultural Sciences NIAS-Japan) was amplified using primers bearing the Spel and SphI restriction sites (Spel-OsSERK-fw: 5’ACTAGTATGGCGGCGCATCGGTGG 3’ and Sphl-OsSERK-rv: 5’GCATGCTCACCTCGGCCCTGGATAG3’) Subsequently, it was cloned in the pGEM®-T Easy vector (Promega). The DNA was then digested with SpeI and SphI and subcloned in an pFF19 vector (Timmermans et al. 1990) that contains 35S promoter and terminator sequences of the cauliflower mosaic virus (CaMV) using XbaI and SphI sites. The recombinant pro35S::OsSERK1::ter35S cassette was then digested with HindIII and EcoRI and subcloned into a pCAMBIA 1301 vector (Center for the Application of Molecular Biology to International Agriculture, Australia) harboring the hygromycin resistance marker gene (hpt) (Chin etal. 2007, Keiichiro etal. 2005). The recombinant cassette was introduced in two A. tumefaciens strains (GV3101/pMP90) by electroporation, with the following conditions: 2.5 kV, 25 µF, 400 Ω. The map of the recombinant T-DNA is shown in Fig. 1.
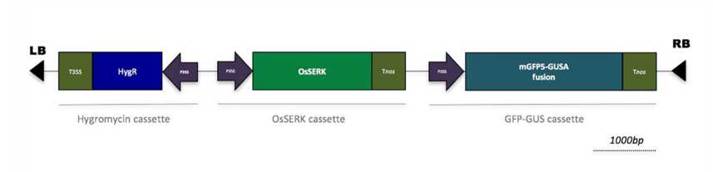
Figure 1. Schematic structure of the T-DNA region in the constructp CAMBIA1301-OsSERK. LB-Left border, RB-Right border, T35S: 35SCaMV terminator, P35S: 35SCaMV promoter. HygR (hygromycin phosphotransferase gene), OsSERK- Orysa sativa Somatic Embryogenesis Receptor like Kinase Gene; mGFP5-GusA fusion. All the coding regions are under the control of CaMV35S promoter and CaMV35S terminator. The orientation of each single cassette is indicated in the figure.
Assessment of A. tumefaciens strains
The transformation efficiencies of the A. tumefaciens strains GV3101/pMP90 (Koncz & Schell 1986) and EHA105 (Hood et al. 1993) were assessed. GV3101/pMP90 carries a gentamycin-resistance gene on the Ti plasmid and a rifampicin-resistance gene on the chromosome, and EHA105 carries a gene for resistance to rifampicin on its chromosome. Three-month old protocorms, in eight replicates (flasks), were transformed with each strain.
Inoculation and co-cultivation
A. tumefaciens was grown for 2 d at 28 °C in 5 mL of LB medium containing 100 mg/L of kanamycin and 100 mg/L of rifampicin. The culture was transferred to 50 mL of LB medium and cultured overnight at 28°C. Four hours prior to transformation, acetosyringone at a final concentration of 200 µM was added. After centrifugation at 3 000 rpm, the pellet was resuspended in 20 mL of MS medium. About 20 mL of resuspended bacteria were added to each plate containing pre-cultured protocorms and incubated at room temperature for 3 h. The protocorms were blotted dry on sterile filter paper and co-cultivated at 25 °C in the dark for 4 d in MS medium containing 200 µM acetosyringone.
Selection of Transgenic PLBs and plant regeneration
To eradicate A. tumefaciens after co-cultivation, the protocorms were washed with MS medium containing 200 mg/L of timentin, transferred to MS medium with 200 mg/L of timentin and 50 mg/L of cefatoxime and subcultured weekly for five additional weeks. A preliminary test using C. maxima PLBs (primary) showed that the minimal selective concentration of hygromycin was 20 mg/L over a period of two months (data not shown). In this study, the selection was extended to five months, with monthly transplant to fresh medium.
PCR screening of transformants
DNA was extracted from 30 mg of leaves, using the Wizard® SV Genomic DNA Purification System (Promega) and following the manufacturer’s instructions. Genomic DNA (8 mg) was directly used for PCR screening with primers annealing on the OsSERK1 gene (5 ’-TGCTCGTCTAGCCAATGATG-3’), and (5’-ATGAGGGCCAAGCTCTACC-3’). PCR amplification was carried out in an Applied Biosystem Thermocycler as follows: 94 °C (5 min), 32 amplification cycles (94 °C for 30 s, 58 °C for 30 s, 72 °C for 1 min) and a final extension cycle of 5 min at 72 °C using GoTAQ polymerase (Promega, Spain).
GFP Analysis
The pCAMBIA-OsSERK1 construct contains a mGFP5-gusA fusion under the control of 35SCaMV promoter and terminators for visual selection of transformants. Detection of GFP epifluorescence was performed using a fluorescent stereomicroscope (Olympus DFPL 0,5X-4, Japan) equipped with a U- RFL-T Filter (Olympus). Images were taken with a Camedia camera (C5060 Olympus, Japan) connected to the stereomicroscope and adjusted using ImageJ software (Rasband, 2016)
Somatic embryogenesis in transformed lines carrying OsSERKl
A total of 80 leaf explants of transformed and control C. maxima plants were cultured either on MS hormone-free medium or MS medium containing TDZ (0.3 mg/L) or BA (0.3 mg/L). The percentage of developing somatic embryos was evaluated after 30 d. The statistical significance of the results was assessed via F-test.
Results and Discussion
Selection of transformed explants
The hygromycin resistance marker gene was chosen because it was previously used for A. tumefaciens-mediated transformation of Oncidium species (You et al. 2003, Liao et al. 2004), and for Phalaenopsis hybrids along with a β-glucuronidase gene sequence (Belarmino & Mii 2000, Chai et al. 2002, Mishiba et al. 2005, Chan et al. 2005). Based on these observations, in the present study we have used a pCAMBIA 1301 vector harboring gene sequences for hygromycin resistance, GUS (uidA), and GFP in addition to an OsSERKl gene copy to evaluate its possible role in the stimulation of somatic embryo formation.
During the 5-month selection step, plants derived from PLBs, characterized by the presence of one or two leaves (Fig. 2a, white arrow) died after two months, while juveniles PLB without leaves formed calli producing new PLBs (Fig. 2b, black arrow) that further developed into plantlets (Fig. 2c). It is worth noting that the developmental stage of the explant is crucial for orchid transformation success. For instance, it was reported that for Oncidium the age of the plantlets used for transformation influenced hygromycin tolerance (Liau et al. 2003). Additionally, in a review (Texeira da Silva 2013) mentioned that coniferyl alcohol, which is known as a vir gene inducer, is present in PLB at a level higher than in any other tissues, thus suggesting that PLBs are ideal for Agrobacterium-mediated transformation. In this work, this observation is confirmed also for C. maxima.
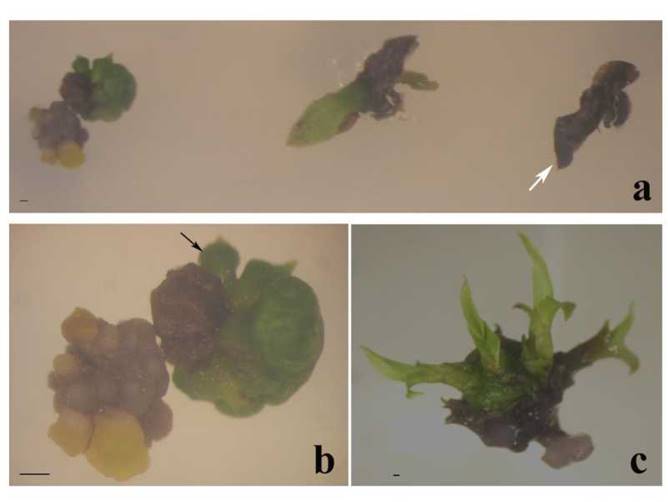
Figure 2 Selection of putative transformants of Cattleya maxima. a) Protocorms of different ages growing on the selection medium (20 mg L-1 hygromycin, MS). The white arrow indicates a dead protocorms. b) Surviving protocorms on the same selective medium (black arrow). c) Shoots developed six months after co-cultivation. (Bar = 0.5 cm).
PCR and OsSERKl gene analysis of putative C. maxima transformants
After a month of growth on the MS selection medium, 89.5% of the of the PLBs had survived. To verify whether secondary PLBs were transformed, a PCR-based test was used to verify the presence of the OsSERK1 gene. An expected fragment (612 bp) was observed in 4 out of the 30 putative transformants (Fig. 3). This low transformation efficiency (approximately 10%), confirmed the need for a more stringent selection step to eliminate false positives. To this end, the selection time was prolonged to five months.
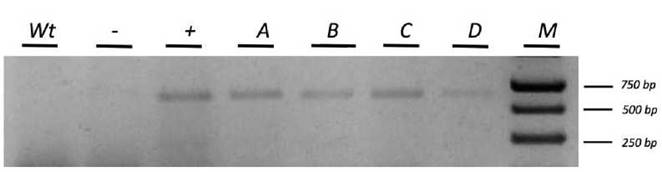
Figure 3 Control of the presence of OsSERK gene in transgenic lines. A band of the expected size (612bp) was observed in selected plants. As expected, the 612bp fragment failed to be amplified in both blank and wild-type, untransformed plant. “Wt” =Wild type plant “A, B, C, D” = selected plants “-” = blank, negative control “+” = pCAMBIA-OsSERK plasmid, positive control.
The ability to transfer a given T-DNA to a host cell depends on its chromosomal background (Yasmine & Debener 2010), the efficiency of the two A. tumefaciens strains was assessed by evaluating the percentage of protocorms surviving to hygromycin selection. GV3101/pMP90 failed to give any transformant while EHA105 strain, typically used for monocot species, yielded (7.4 ± 1.8)% viable PLBs after protracted following hygromycin selection.
The presence of GFP5-GUSA fusion protein in pCAMBIA-OsSERK1 offers the possibility to identify transformants by observing epifluorescence. After a growth period of five months on Hyg-selective medium, putative transformants were randomly screened. At inspection, all putative transformants presented normal phenotypes, and no signs of dwarfism were observed. Fluorescence was detected in 70% of both calli (Fig. 4) and regenerated plants (Fig. 5). No background fluorescence was observed in untransformed calli and plants (data not shown).
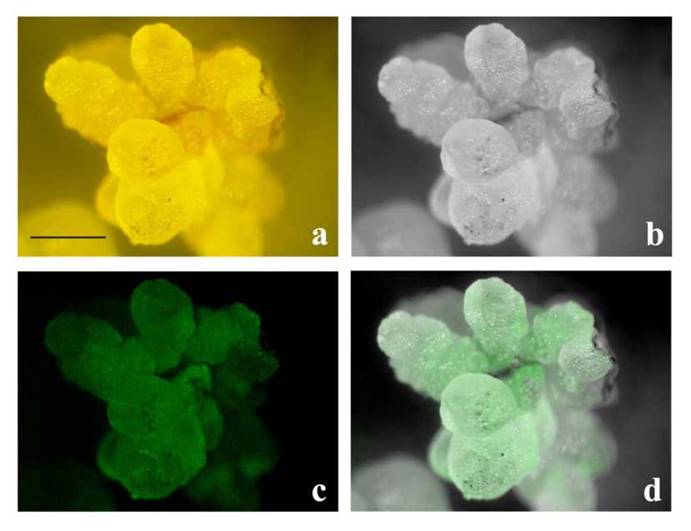
Figure 4 GFP-epifluoresence in a callus generated from a protocorm of C. maxima co-cultivated with A. tumefaciens. Panels a) and b) show a regenerated transformant examined using a fluorescence stereomicroscope. a) is in true color while, b) is the black-and-white version, c) GFP epifluorescence, d) overlay of b) and c). (Bar = 0.5 cm)
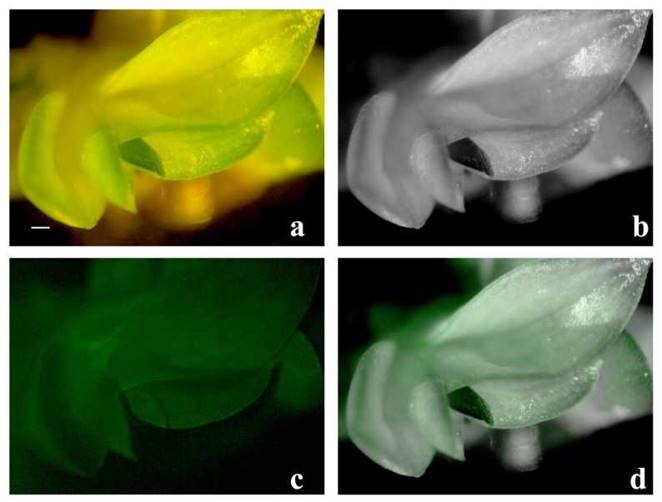
Figure 5 GFP epifluorescence in an eight-month regenerated plant of C. maxima. Panels a) and b) show a putative transformant examined using a fluorescence stereomicroscope; a) is in true color while b) is the black-and- white version; panels c) shows epifluorescence in the sample and panel d) shows the overlay of b) and c). No background fluorescence was observed in wild type plants (data not shown). (Bar = 0.5 cm)
Somatic embryogenesis in transformed explants
A significantly higher percentage of embryos (80% compared to 57%) were formed from in C. maxima leaf explants, containing the OsSERK1 cassette, obtained from plantlets regenerated from secondary PLBs (Fig. 2C) as compared to those derived from wild type plants when cultured on hormone-free MS medium (Table 1). Furthermore, the total number of embryos per leaf was significantly higher in leaves obtained from transformants grown on hormone-free MS medium compared to untransformed leaves: 2.3 ± 0.7 versus 1.5 ± 0.2 (F = 0.022). Interestingly, the presence of TDZ or BA resulted in a reduced percentage of embryo formation (Table 1). Since it is well known that a proper balance of auxin/cytokinin stimulates embryogenesis in orchids (Novak et al. 2014) it is tempting to speculate that the external addition of cytokinins might interfere with the endogenous physiological auxin/cytokinin ratio necessary for somatic embryogenesis.
Conclusion
In the present work, we describe a method for the stable transformation of the wild orchid C. maxima mediated by A. tumefaciens. We have also developed a method for the regeneration of transformed plants via somatic embryogenesis through a dedifferention-regeneration cycle that includes the formation of secondary PLBs. The higher number of embryos obtained from leaves containing recombinant heterologous OsSERK1 gene, as compared to untransformed plants, points to its involvement in orchid somatic embryogenesis.