Introduction
Probiotics are live microbial organisms that, when administered in adequate amounts, are beneficial to their host’s health. Probiotic bacteria are known to inhabit several places of the host organism and are part of the intestinal microbiota in humans. Probiotics have the capacity to prevent and improve health conditions, such as infections caused by several pathogens, including viruses [1-4].
Several mechanisms whereby probiotic bacteria are beneficial to their hosts have been proposed. First, probiotic bacteria can prevent the attachment of some pathogens via colonization of the intestinal epithelial surface. Secondly, it has been observed that probiotics have the capacity of binding to cellular receptors on the surface of mature enterocytes, thereby interfering with pathogen penetration processes into these cells. Thirdly, probiotics can also elicit the activation of the intracellular mechanisms of transcription, translation, and stimulate the host’s immune response, thus strengthening its defenses against various pathogens, including viruses [3,4].
Rotaviruses (RVs) are considered as one of the most important viral pathogens leading to diarrhea in children worldwide. Although vaccines have proven effective in decreasing the strength of RV-induced diarrhea [5,6], researchers continue seeking for alternative ways to prevent this disease [3,7-9].
The mechanism of cell infection of several RV strains has been hypothesized to be mediated by sialic acid residues on the cell membrane, acting as receptors. However, RV infection also requires co-receptors, such as integrins (i.e., α2β1, αvβ3, αxβ2, and α4β1) and thermal shock proteins (i.e., hsc70) [10-16].
Four probiotic bacterial strains that decrease RV infection have been identified via in vitro assays [17,18]. This finding raises the question whether the observed positive effect of these probiotic bacteria is achieved by blocking specific host cell receptors necessary to viral adhesion and penetration. The aim of the present study was to determine if probiotic bacteria extracts could decrease RV infection by binding to host cell receptors necessary for RV entry.
Materials and methods
Probiotic bacteria, cell line, and virus strain
Probiotic bacteria of the species Lactobacillus casei, Lactobacillus fermentum, Bifidobacterium adolescentis, and Bifidobacterium bifidum, previously studied by Fernandez et al. [17] and Olaya et al. [18], were used for the experimental approaches in the present study. Bacteria were grown on MRS broth under anaerobic conditions until exponential growth was obtained (between 8-10 hours depending on the strain).Cultures at a cellular concentration of 108 CFU/mL were used for the development of the experiments as reported elsewhere [19].
Rhesus monkey kidney-derived MA104 cells were used to replicate the virus and to evaluate bacterial extracts. MA104 cells were grown in Dulbecco’s modified Eagle’s medium (DMEM) supplemented with 4% fetal bovine serum (FBS), 2 mM L-glutamine, and an antibiotic/antimicotic cocktail (comprising 10 000 μg/mL penicillin, 10 000 μg/mL streptomycin, and 25 ug/mL Fungizone at a 100X concentration). Cells were harvested using phosphate buffer solution (PBS) with trypsin (0.25 %) at 37 °C until they detached from the culture flask. The assays were performed in 24-well plates, with a cell confluence of 90%.
Antiviral assays were performed with the Rhesus rotavirus (RRV) strain. Prior to MA104 infection, viral particles were activated with 10 μg/mL of trypsin to cleavage capsid proteins. All viral infections were done at a multiplicity of infectionof 0.5. The times for viral adhesion and penetration were set to 90 minutes for all experiments.
Probiotic bacteriaextracts and MA104 cell viability
Bacterial strain cultures at exponential growth and a cell density of 108 UFC/mL were centrifuged at 3-000 x g for 30 minutes at 4 °C. Subsequently, supernatants were discarded, and bacterial pellets were incubated with Tris-HCl20 mM and 80 μL Lysozyme (25 mg/mL; Thermo, Massachusetts, USA) at 37 °C for 30 minutes. A volume of 1 mL 1 % of N-lauroylsarcosine (Sigma, Darmstadt, Germany) was added for solubilization and separation of bacterial membrane proteins and glycoproteins.
Bacterial cells were then sonicated at an amplitude of 45 % for 10 cycles. Each cycle lasted 15 seconds and was followed by 45 seconds at 4 °C. Sonicated bacterial preparations were then centrifuged at 2 800 x g for 3 minutes, supernatants recovered, and pellets resuspended in Tris-HCl 20 mM to a volume equal to that prior to centrifugation. This sonication-centrifugation step was repeated 10 times.
Bacterial suspensions were vortexed and centrifuged at 11200 x g for 30 minutes at 4 °C and supernatants were discarded. A total of 10 mL of PBS were added to each pellet, enriched for surface proteins, and vortexed. Then, suspensions were filtered through an AG 11A8 resin column to remove acid from non-ionic molecules (Bio-Rad, California, USA).
Each sample was centrifuged once more with 1 mL Tris-HCl20 mM at 11 200 x g for 30 minutes at 4 °C to remove any further residual detergent. The supernatant was discarded, and the remaining pellet (henceforth probiotic extract) was re-suspended in 2 mL of Tris-HCl 20 mM. Protein concentrations were measured using a NanoDrop 2000 UV-Vis Spectrophotometer (Thermo, Massachusetts, USA) and by the bicinchoninic acid technique.
To assess the cytotoxic effect of obtained probiotic extracts against MA104 cell cultures, viability assays were conducted. MA104 cells were exposed to extracted bacterial proteins at a concertation of 1 mg/mL and were incubated for 12 hours. Cytotoxic effect was evidenced by the MTT method, measured at 580 nm in a Multiskan FC microplate photometer (Thermo scientific, Massachusetts, USA) using DMEM as a negative control.
Antiviral activity of the bacterial extracts
All experiments were performed with MA104 cells cultured to 90 % confluence and RRV cultured for 15 hours at 37 °C. The antiviral activity of the four probiotic bacteria extracts was measured with immunocytochemistry assays. Briefly, MA104 cells infected with RRV and treated with probiotic bacteria extracts were fixed with methanol for 1 hour at -20 °C. A polyclonal antibody directed to RV structural proteins was employed to detect viral infection. The level of viral infection was evidenced with a horseradish peroxidase (HRP) goat anti-rabbit IgG conjugate. The reaction was analyzed using 0.64 mg/mL of aminoethylcarbazole (AEC) prepared in dimethylformamide with acetate buffer (sodium acetate30 mM, acetic acid 12 mM), pH 5.0, and 0.36 % hydrogen peroxide as substrate. Infected cells were counted using common light microscopy and were identified by their brown color, because of the presence of viral antigens.
The following experiments were aimed at establishing if probiotic extracts interacted with cellular receptors or co-receptors associated with the attachment or entrance of viral particles, as necessary first steps of viral infection. After exposure of the MA104 cells to probiotic extracts, virus adhesion and penetration were tested. Incubations of viral suspensions were tested at two different temperatures. Adhesion assays were tested at 4 °C, to block the immediate entrance of the virus, while penetration assays were done at 37 °C to guarantee an immediate entrance of the virus after adhesion.
Initially, serial dilutions of the probiotic extracts were added to the MA104 cells (from 1 mg/mL to 0.1 μg/mL) to identify the probiotic extract concentration that best decreases viral infection under adhesion and penetration conditions. Probiotic extracts at a concentration of 1 mg/mL were used in all subsequent experiments. Bacterial protein extracts were incubated at 37 °C for 1 hour with MA104 cells seeded in 24 well-plates and then washed with PBS to remove unbound protein molecules. Next, viral suspensions were added to the wells and were incubated either at 4 °C or at 37 °C for 90 minutes. After incubation time elapsed, supernatants of the viral suspensions were removed, and infected plates were incubated at 37 °C in the presence of CO2 for 10 hours. Untreated infected cells were used as a positive control of infection. Experiments were performed in duplicate and replicated twice in time.
Further assays were conducted to confirm if the mechanism of antiviral activity exerted by probiotic extracts was achieved by interfering with virus-cell receptors/co-receptors biding at the adhesion or penetration steps of the infection. In the viral adhesion interference assay, viral particles were first added to MA104 cell cultures and then probiotic extracts were added. Whereas, in the viral penetration interference assay probiotic extracts were added first to MA104 cell cultures followed by viral particles. Both experiments were performed at 4 °C for 1 hour. Subsequently, supernatants were removed, and cultures were incubated at 37 °C for 10 hours.
Probiotic bacteriaextract composition: protein nature assays
All obtained probiotic extracts were characterized following three protein analysis approaches. (i) SDS-polyacrylamide gel electrophoresis (PAGE) were performed with a 10 to 220 kDa Bench Mark Protein Ladder, used as a molecular weight marker and stained with Coomassie blue. (ii) A heat denaturation assay, where probiotic extracts were boiled at 99 °C for 15 minutes. Finally, (iii) Enzymatic digestion of bacterial extracts was performed. Probiotic extracts, at a concentration of 1 mg/mL, were treated with 20 ug/mL of proteinase K. Following both heat denaturation and enzymatic digestion, antiviral assays were repeated to determine whether trated extracts maintained their inhibitory effect. Assay results were evidenced by immunohistochemistry.
Confirmatory assay of antiviral activity
After characterizing probiotic extracts composition, confirmatory assays for their antiviral activity were performed. Probiotic extract antiviral activity was challenged with the following assay. Polyclonal sera directed to each probiotic extract were obtained by inoculating mice with each probiotic extract following the protocol established in the “Guidelines For The Production Of Antibodies In Laboratory Animals” (2014). Afterwards, probiotic extracts were incubated with serial dilutions(1/10, 1/20, 1/40, 1/80, 1/160, 1/320) of anti-extract antibodies in a volume of 100 μL for 1 hour. Then, these mixtures were added to MA104 cells and were exposed to the virus.
Interaction of bacterial protein extracts with β3-integrin and Hsc70 proteins
The interaction between cellular receptors (Hsc70 and β3-integrin proteins) and proteins in the probiotic extracts was evaluated via co-immunoprecipitation assays, immunodotting, and immunoblotting.
The co-immunoprecipitation assay proceeded as follows: MA104 cells, at 90 % confluence, were exposed to bacterial extracts at a concentration of 1 mg/mL for 1 hour at 37 °C. Cells were then washed, detached with cell scrapers, and recovered in DMEM medium without FBS. They were centrifuged at 2 500 x g for 5 minutes and lysed with 500 μL radio-immunoprecipitation buffer (NaCl150 mM, Tris50 mM, NP1 %, DOC 0.5 %, Triton X100) in the presence of 20 μg/mL phenyl-methane-sulfonyl-fluoride (PMSF and serine protease inhibitor). Subsequently, cell lysates were incubated for 1 hour at 37 °C followed by centrifugation at 11 200 x g.
For the immunoprecipitation assay, 5% A-agarose protein beads (BioRad, California, USA) were incubated with rabbit polyclonal sera directed to β3-integrin or anti-Hsc70, at a 1:20 ratio, with 2% ovalbumin. This incubation was performed for 10 minutes at room temperature at constant shaking, and it was followed by three washes with PBS and centrifugation at 2 500 x g for 3 minutes per washing. Afterward, the A-agarose-Ac anti- β3 or anti-Hsc70 protein beads were incubated for 1 hour at 37 °C at constant shaking with cell lysates. The beads were washed three times with PBS and centrifuged at 2500 x g for 3 minutes between washes.
The immunoprecipitate was also used to perform an immunodot. In this assay, 20 μL of the previously immunoprecipitated samples were added to a PVDF membrane. The membrane was blocked with 5 % skim milk for 1 hour at room temperature with agitation and washed with PBS. Then, a final incubation with 50 μL of the polyclonal sera against the protein extracts was done. The solution was incubated for 1 hour in constant movement, and after washing, anti-mouse HRP-conjugated IgG was added.
Statistical analysis
The results were analyzed using ANOVA, in which treatments were considered as fixed effects. This analysis was followed by multiple comparison tests (post hoc tests) including Tukey’s, least significant d ifferences, and Dunnett’s tests. P values of each test were obtained at a significance level of α = 10 %. Results were considered significant when two out of three post hoc tests obtained a value of 3.
Results
Antiviral effect of probiotic bacteria extracts
Initial cell viability assays revealed that the toxicity of the four probiotic bacteria extracts on the MA104 cells did not exceed 30 %. The antiviral effect of probiotic bacteria extracts was tested with two assays. In the first approach, both adhesion and penetration steps of the viral cycle evaluated at 4 °C and 37 °C, respectively, resulted in viral infection reductions (Fig. 1) At 4 °C, the presence of all four bacterial extracts led to a normalized fraction of infected MA104 cells below 8 %, indicating a significant (P < 0.1) reduction of the fraction of MA104 cells infected with RV. In contrast, at 37 °C, the lowest normalized fraction of infected MA104 cells was 15 %, being observed in the presence B. bifidum extracts, and the highest normalized fraction of infected cells was 32 %, observed in the presence of L. casei extracts. In this assay none of the tested probiotic extracts significantly reduced the fraction of viral infections on MA104 cells (Fig. 1).
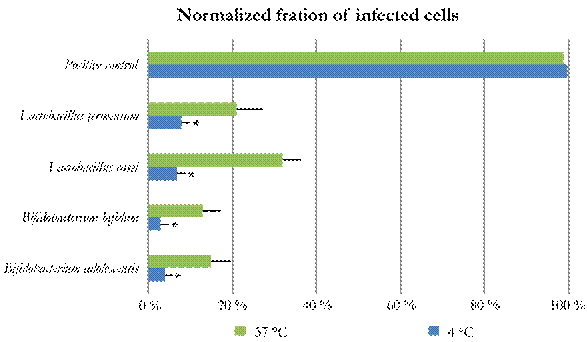
Figure 1 Inhibition of viral replication at two assay temperatures. Inhibition is understod as 1 - the fraction of infected cells. Inhibition assays at 4 °C . Inhibition assays at 37 °C. A singnificant viral infection inhibition was observed at 4 °C (P < 0.1). Error bars correspond to 90 % confidence intervals.
The second assay was intended to test whether bacterial extracts could inhibit viral infection by interacting with cellular receptors or co-receptors, changing the order of exposure of the MA104 cells to probiotic extracts. When probiotic extracts were first added to the cells, the observed normalized fraction of infected cells was below 27% (P < 0.1); whereas, when the virus was first added to the cells, and then the bacterial extracts, the observed values of the normalized fraction of infected cells ranged from 50 % to 60 % (Fig. 2). This effect of probiotic extracts during the phases of viral adherence and penetration indicated that probiotic extracts were more effective during virus adherence phase rather than during its penetration phase.
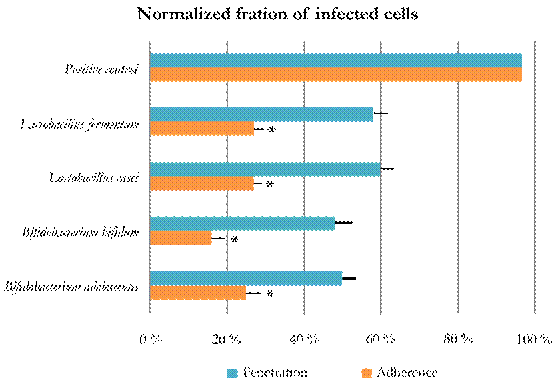
Figure 2 Inhibition of viral replication depending on the order of addition of probiotic bacterial extracts and virus. Inhibition is understod as 1 - the fraction of infected cells. Adherence inhibition assays. Penetration inhibition assays. Singnificant viral infection inhibition was observed in all adherence assays (P < 0.1). Error bars correspond to 90 % confidence intervals.
Protein extract composition nature assays
All four probiotic bacteria extracts were first subjected to 10 % SDS-PAGE, in which bands between 20 to 100 KDa were observed. This suggested that all four bacterial extracts were enriched for proteins. Subsequently, the antiviral effect of these probiotic extracts was evaluated under two denaturating conditions. Antiviral assays performed with extracts treated with heat or proteinase K failed to inhibit viral infection, unlike the antiviral assays performed with protein extracts in their native state (Fig. 3). In the latter, a viral infection inhibition higher than 60 % was observed, the extracts of some strains achieved low infection percentages.
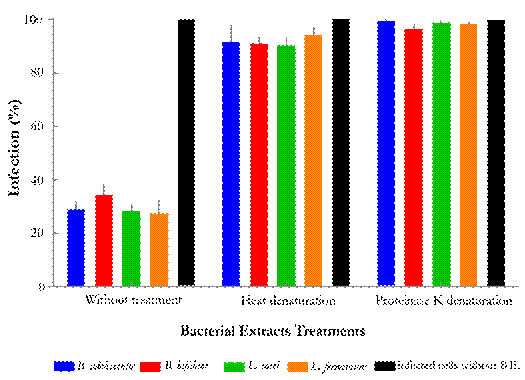
Figure 3 Percent of MA104 cells infected with virus in presence of four denaturated probiotic protein extracts. Cells were in contact with virus and the extracts of the four probiotic bacteria. One group of extracts was heated and the other one was mixed with proteinase K. The antiviral activity decrease when bacterial extracts were treated with both different denaturation approaches. B. adolescentis; B. bifidum; L. casei; L. fermentum; Positive control - RRV infected cells.
Antiviral activity confirmation
One last test was performed to confirm the inhibitory effect of the probiotic bacteria extract on viral infection. As shown in Fig. 4, when bacterial extracts were mixed with their respective anti-sera, their antiviral effect was compromised. As the concentration of antibodies against the extracts decreased, antiviral activity increased, confirming the ability of these extracts to inhibit RV infection.
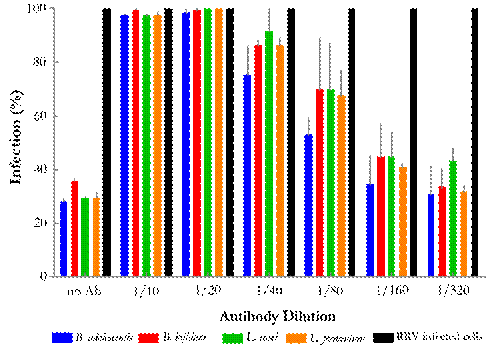
Figure 4 Specific-antibody assay directed to probiotic bacteria extracts to demonstrate their antiviral action. Notice antiviral activity when antibody concentrations decrease, mediated by the blocking effect exerted by probiotic proteins. B. adolescentis; B. bifidum; L. casei; L. fermentum; Positive control - RRV infected cells.
Interaction of probiotic extracts with β3-integrin and Hsc70 cellular receptors
After co-incubation and immunoprecipitation of cell membrane-probiotic protein extract complexes with protein A sepharose, ligated either toanti- β3 or anti-Hsc70 antibodies, immunodot and immunoblot assays were performed using anti-bacterial protein extract antibodies. Immunodot analyses revealed that all four probiotic extracts were bound to proteins Hsc70 and β3-integrin (Fig. 5). Likewise, the immunoblot assay showed reaction bands at different molecular weights that bound to Hsc70 and β3-integrin upon precipitation (Fig. 6). This assay showed the presence of an extract-Hsc70-anti-Hsc70 complex using the anti-extract antibody previously produced in mice.
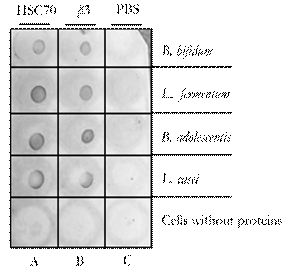
Figure 5 Immunodot assay. Dotblot showed the presence of bacterial extracts binding to cell proteins Hsc70 (A) and β3 (B). Cell without protein bacterial whit PBS (C).
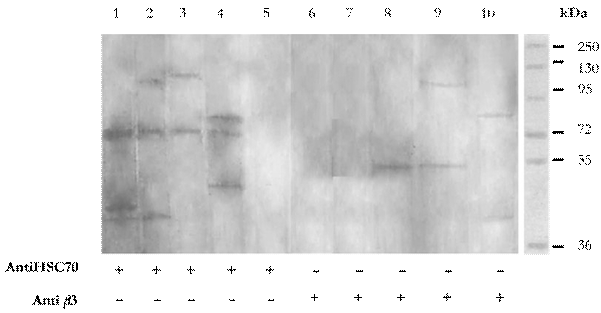
Figure 6 Immunoblotting assay. Lanes 1 and 7 reveal the presence of Hsc70 and β3 integrin in the membrane of L. fermentum. Lines 2 and 8 reveal the presence of Hsc70 and β3 integrin in the membrane of β. bifidum. Lanes 3 and 9 reveal the presence of Hsc70 and β3 integrin in the membrane of L. casei and lanes 4 and 10 reveal the presence of Hsc70 and 33 integrin in the membrane of B. adolescentis. Lanes 5 and 6 had cells MA104 without extracts.
Discussion
Probiotics have the capacity to prevent infectious processes. Several mechanisms have been proposed to describe their antiviral role. These include saturation of the mucous layer in the intestine to prevent pathogen passage and blockage of cellular receptors preventing the adhesion and penetration of pathogens [3,4,20,21]. Release of substances that block the transcription and translation mechanisms of pathogens; or activating the immune response to increase its effectiveness against pathogens [21].
The results shown in this paper provide evidence of the in vitro mechanisms exerted by probiotics to reduce RV infection by means of blocking adhesion of viral particles to host cells, as observed in all experiments involving probiotic bacterial extracts, virus, and MA104 cells. Comparable antiviral effects were observed with all the tested probiotic extracts. Considering the composition of bacterial cell membranes [3], and regarding the extraction method selected for this study (N-lauroylsarcosineprecipatates), probiotic extracts were expected to be protein rich [22]. Thus, the quantification strategies of Coomassie blue staining, spectrophotometry, and bicinchoninic acid assays were chosen according to this expectation. In addition, the protein denaturation methods of proteinase K and thermal shock at 99 °C were performed to the same batches of probiotic extracts with the expectation of inhibiting their antiviral effect [23,24]. The results of these assays showed that probiotic extract antiviral activity was blocked after denaturation assays were performed.
In addition, co-immunoprecipitaion assays revealed the ability of probiotic extracts to interact with cellular Hsc70 and β3-integrin. These molecules are involved in RV entrance into mature enterocytes of the small intestine villi and into cell lines MA104 and Caco2, that possess enterocyte-like cellular receptors [10,16,25]. However, it is important to clarify that Hsc70 and β3-integrin are not the only receptors for RV. Other molecules are also involved in this RV infection [11,16,25,26]. In this study, it was also shown that probiotic extracts can bind to MA104 cells, possibly via electrostatic interactions between cellular receptors and biding motifs in the extract [27,28].
The processes of bacterial adhesion to the gastrointestinal mucous layer are complex and involve non-specific interactions, like electrostatic forces, hydrophobic interactions, and specific interactions. These are excreted by bacterial adhesins and surface proteins that bind to mucosal receptors [21]. From a clinical perspective, these processes possibly take place in pediatric patients with viral gastroenteritis when supplied with probiotic bacteria [29,30].
The present work results further support the view that probiotic bacteria extracts have proteins with antiviral effects. It has been demonstrated that compounds present on the cell surfaces of probiotic bacteria have the capacity to prevent colonization of the intestinal tissue by different pathogens [3,31]. Additionally, some Bifidobacterium spp. and Lactobacillus spp. strains possess surface proteins that allow the first contact with cell surface molecules in the host, thus improving their adaptive capacities to the gastrointestinal environment, the colonization of different tissues, and the induction of cell responses in the host carrying these bacteria [21,32].
Other studies with probiotic strains with antiviral effect such as Lactobacillus delbrueckii OLL1073R-1 (LDR-1) indicated that they produce immunomodulatory extracellular polysaccharides (EPSs). EPSs are molecules that allow the communication of immunobiotics with the host by interacting with pattern recognition receptors expressed in non-immune and immune cells [33]. Kanmani et al. [34] showed that innate immune response triggered by Toll-like receptor (TLR3) activation in porcine intestinal epithelial cells (IECs) was differentially modulated by EPS from LDR-1 have a potential to be used for improving intestinal innate antiviral response and protecting against intestinal viruses such as rotavirus.
The results of all viral infection inhibition assays in the preset study support the view that proteins present in probiotic extracts compete with those of enteropathogenic ones for binding to host cell receptors involved in infection [21,25,35].
The line of evidence in support of the above comes from the viral adherence interference assay. When the extracts were applied first to MA104 cells, followed by the addition of the virus at 4 °C, probiotic proteins were likely to bind to cellular receptors necessary for adherence and subsequent entrance of the virus. Guerrero et al. [25] proposed that at 4 °C, a virus can successfully adhere to the cell surface but cannot penetrate it. This can explain the contrasting results obtained with the adherence- vs. penetration interference assays.
A thorough study of probiotic bacteria molecules with effective antiviral activity could led to the development of antiviral therapeutics, particularly trough specific inhibition of RV infection. In this study, it has been shown, with complementary approaches, that the molecules most likely involved in the antiviral activity of the tested probiotics were proteins.
In conclusion, protein extracts obtained from the probiotic bacteria Lactobacillus casei, Lactobacillus fermentum, Bifidobacterium adolescentis, and Bifidobacterium bifidum were capable of inhibiting RRV strain infection of MA104 cells, by blocking viral adhesion rather than penetration. The host membrane proteins Hsc70 and β3-integrin, which act as co-receptors of the viral infection, are involved in this blockage mechanism. Further studies, should focus on purifying and characterizing the inhibitory molecules to propose new antiviral substances against RV.