Introduction
Urbanization is an accelerating global phenomenon. In developing countries, vehicle density, construction, and metal emissions increase with urban growth, leading to an overall lifestyle change [1]. Metals are contaminants that, due to their prevalence, toxicity, and persistence, are of concern in any environmental system [1]. These pollutants originate from varied industrial activities such as electroplating, tanning of hides, metallurgy, photography, battery production, car workshops, paint production, etc. Metal toxicological effects are relevant to both ecological and public health, since they are associated with respiratory diseases, skin diseases [2], and DNA damage, which increases carcinogenic risk [3].
Electroplating is a metalwork industry activity in which the treatment of metal surfaces is carried out through chemical and/or electrochemical processes [4]. It involves a sequence of pretreatment, coating and conversion baths, that lead to several types of wastewaters containing high amounts of metals, principally zinc, iron and hexavalent chromium [4]. Chromium (Cr) species are also waste byproducts of electroplating activities. Cr exists at valence states ranging from -2 to +6, with the dominant forms in the environment being Cr6+and Cr3+ [5]. Whereas Cr3+ has a lower toxicity and lower bioavailability due to its impermeability to biological membranes, Cr6+is toxic to biological systems due to its mutagenic, carcinogenic, and teratogenic effects, [5]. Consequently, aChromium waste discharge policyhas been locally set forth and a permissible chromium concentration in wastewater was set at 0.5 mg/L for discharge into clear water bodies or into the public sewer system in Colombia [6].
Adhering to this regulation, certain industries have implemented conventional methods for removing heavy metals, such as chemical precipitation, chemical oxide reduction, filtration, ionic exchange, and electrochemical treatment [7]. However, in conventional treatments the reduction of Cr6+may be incomplete and the chemicals used are hazardous to the environment, besides being costly [4]. Developing efficient, low-cost technologies such as bioremediation is, thus, necessary. These new technologies should provide the possibility of degrading, removing, or reducing pollutants to non-toxic forms, or to forms that are less toxic to human health and the environment [8].
Microorganisms of the genus Bacillus have been recognized for their ability to reduce Cr6+[9-13]. Specifically, it has been found that the Bt strain of B. cereus, isolated from tannery effluent water, tolerates up to 8 000 mg/L of Cr6+and reduces Cr6+at a concentration of 100 mg/L in Luria-Bertani (LB) medium (unpublished) with 100 % efficiencies Tripathi etal. [14], reported the ability of the B. cereus strain RMLAU1 to tolerate and bioremediate Cr6+at a concentration of 200 mg/L and pentachlorophenol (PCP) at a concentration of 500 mg/L, simultaneously. Taking this into account, this study intends to evaluate the ability of B. cereus B1 to reduce Cr6+present in the effluents of an electroplating plant.
Materials and methods
Sampling and sample processing
Wastewater samples were taken from the effluents of an electroplating facility located in the city of Cali, Departamento del Valle del Cauca, Colombia. Samples were stored in sterile glass jars previously washed with 10 % HNO3 (J.T. Baker), then transported in coolers at 4 ◦ C to the Microbiological Research laboratory at the Universidad del Valle, where they were processed within six hours. Effluent pH and temperature were measured at the sampling site. The pH of wastewater was later adjusted to 7.5 ± 0.2 using Tris buffer, which was seen during previous research to be optimal for B1 native strain growth.
Reduction bioassays
Bioassays were performed using a wild strain of B. cereus, which was previously identified using the BD BBL CRYSTAL GP kit. This was complemented using amplification (PCR) of the 16S rRNA gene region, from a bacterial colony grown in solid Luria-Bertani medium. The primers 16S-F (5'- AGAGTTTGATCCTGGCTCAG-3') and 16S-R (5'GGCTACCTTGTTACGACT T-3') were used. PCR cocktails underwent a “touch-down” thermic profile. PCR products were visualized in a 1.5 % agarose gel with TBE buffer stained with SYBR®Safe (Invitrogen). The sequencing process was performed by MACROGEN (Korea). 16S rRNA gene sequences were compared with known sequences from the GenBank database to identify the most similar sequence.
Bioassays were carried out in triplicate using real wastewater (RWW) from an electroplating facility with a Cr6+ concentration of 5 mg/L, as well as artificial wastewater (AWW), with and without glucose (Merck). The water was sterilized by filtration through a nitrocellulose membrane with 0.2 /dm pore size (Sartorius Stedim Biotech). The glucose concentration for all bioassays was 0.2 g/L. In order to determine whether other factors could influence the Cr6+ reduction process in RWW, AWW was prepared by dissolving the following: 14.11 mg/L NiSO4VH2O (Merck), 0.07 mg/L ZnSO4 .7H2O (Merck), 0.15 mg/L CuSO46H2O (Merck), 0.21 mg/L Pb.(NO3)2 (Merck), and 5.25 mg/L K2Cr2O7 (Merck). These concentrations were established based on prior physicochemical analysis on RWW obtained from the electroplating facility.
Each bioassay consisted of a 250 mL Erlenmeyer flask containing 50 mL of wastewater and a bacterial inoculum. The inoculum was prepared using colonies from a master-seed lot, seeded in 1 000 mL Luria-Bertani medium (Becton Dickinson AB) incubated at 30 ◦ C for 17 hours under constant 125 rpm agitation (126R Incubator Shaker Stack, New Brunswick Scientific). After the culture grew, it was transferred to 12 conical tubes (Falcon) containing 50 mL each, which were centrifuged at 4 000 rpm for 10 min (Hettich Universal 32R centrifuge). The supernatant was discarded, and the pellet was used as inoculum. For each treatment a chemical control containing 50 mL of wastewater with and without glucose was included. A total of 16 Erlenmeyer flasks were prepared and incubated at 30 ◦ C and 125 rpm (I26R Incubator Shaker Stack, New Brunswick Scientific).
Three independent samples were taken, and three assays were carried out for five days. The Cr6+ concentration and bacterial growth were measured three times per day, and the pH was determined at the beginning and end of each trial. The Cr6+ concentration was measured by spectrophotometry using 1,5-difenilcarbazide at 540 nm, using HACH DR 890 equipment [15]. Bacterial growth was measured using a spectrophotometer, quantifying optical density at 600 nm (Thermo Spectronic Genesys 20, digital UV VIS).
Data analysis
Results of Cr6+ reduction percentage and bacterial growth over time were analyzed graphically. Significant differences were evaluated using an analysis of variance with a 22 factorial design with blocking. When statistically significant differences were found, a post-hoc Tukey test was used. All statistical analyses were conducted with the R Software.
Scanning electron microscopy
A total of 2 mL from each assay and 2 mL from pure B. cereus B1 culture, (control) grown in liquid medium (LM) for 24 hours, were taken. These samples were centrifuged at 4 000 rpm for 10 min (Spectrafuge 16M centrifuge), discarding the supernatant. Microorganisms were later fixed in a glutaraldehyde solution (Carlo Erba) at 2.5 % and 0.1 M 7.2 pH phosphate buffer for 12 hours at 4 ◦ C. Fixed samples were then washed twice in a phosphate buffer and dehydrated at 4 ◦ C for 10 min in increasing ethanol concentrations (Sigma-Aldrich) ranging from 30 % to 99.8 %. Samples were immediately dried by adding hexamethyldisiloxane (Sigma-Aldrich) for 30 seconds and covered with a gold film for immediate observation.
Results and discussion
Results of the RWW physicochemical analysis are shown in Table 1. These parameters indicated chromium and nickel pollution, with values above the maximum permissible value according to Colombian environmental standards [6]. We also observed low biochemical oxygen demand and chemical oxygen demand levels, which means that the bacterium could hardly carry out metabolic processes as it is a heterotrophic organism.
Table 1 Physicochemical analysis of wastewater from an electroplating plant.*Maximum Allowable Value for the discharge of wastewaters from electroplating coating activities, according to the Colombian environmental standards [6].
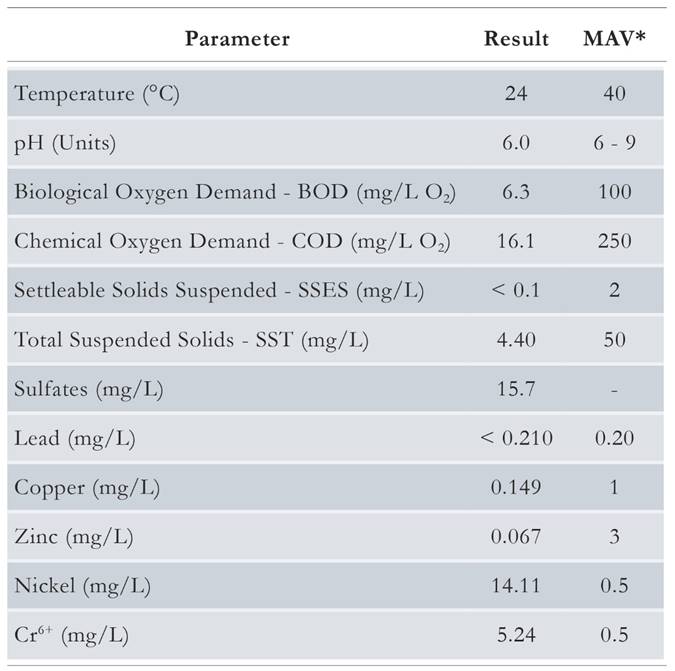
Treatments with glucose resulted in a higher reduction percentage of Cr6+concentration than treatments without glucose. Fig. 1 shows averages of Cr6+reduction percentage in treatment replicates and bacterial growth during 120 h of incubation. Cr6+ was reduced by 24 % during the first 30 h using RWW (Fig. 1a), reaching 29.3 % reduction at the end of the assay. Reduction was 72 % using AWW (Fig. 1b). In assays with glucose there was 83.3 % Cr6+ reduction after 78 h using RWW, and 100 % reduction using AWW after 48 h (Fig. 1c and 1d).
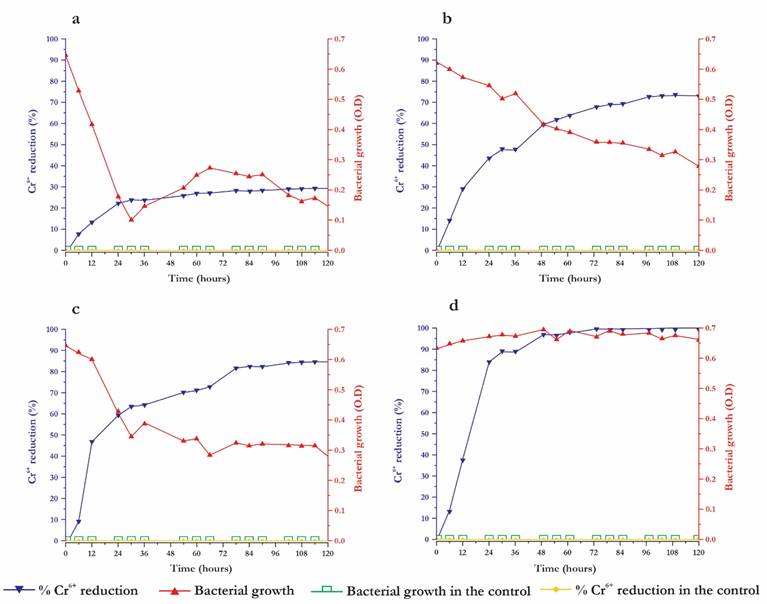
Figure 1 Percent of Cr6+ reduction and bacterial growth in treatments and controls: (a) real wastewater. (b) artificial w astewater. ( c) r eal w astewater w ith glucose. (d) artificial wastewater w ith g lucose. D ue to t he b acterial g rowth a nd the Cr(VI) reduction in the controls did not change, both are represented on the horizontal axis.
In bioassays 2 and 3 (data not shown) we observed similar results to bioassay 1 (Fig. 1), with lower Cr6+reduction values. Using RWW, the observed Cr6+reductions were 29%, after 54 h and 37.2 %, after 78 hours, while using AWW we obtained a Cr6+reduction of 64.2% after 78 h and of 77.6% after 114 h. Ostensibly, the presence of glucose accelerated the Cr6+reduction process. We obtained a 74.4 % reduction using RWW after 102 h and 69.2 % after 78 h, whereas using AWW with glucose we obtained 100% reduction after 102 h and 78 h. Tripathi et al. [16], point out that among different carbon sources (glucose, maltose and sucrose), glucose was the easiest metabolizeable sugar. Moreover, this molecule allows the conversion of NADP to its reduced form, which then provides reduced metabolites [17]. Glucose is also an important source of energy, and along with other trace elements, increases growth of live cells and facilitates metal bioabsorption [18].
The statistical analysis showed no significant differences between bioassays for Cr6+reduction (p > 0.05), but there were significant differences between water types (p < 0.05), glucose presence (p < 0.05), and the interaction effect ofwater type - glucose (p < 0.05) (Table 2). The post-ANOVA Tukey analysis showed that there was a difference of -0.4618 for wastewater type (real/artificial), and of 0.511 for glucose (presence/absence), indicating that the factors artificial water and glucose presence resulted in higher Cr6+reduction compared with real water and glucose absence.
Despite AWW being prepared in similar concentrations to those reported in the physicochemical analysis (Table 1), it resulted in a higher Cr6+reduction percentage than when using RWW. This is due to AWW being prepared from only sulfate and nitrate salts, which do not compete with Cr6+as electron acceptors under aerobic conditions, even at concentrations of 40 mg/L and 80 mg/L. [19] Industrial effluents are characterized by having a great variety of ionic components (including cations and anions), which have a negative effect on metal accumulation [20].
It has also been reported that the presence of Pb2+, Cu2+, and Zn2+, even at low concentrations, is limiting in terms of the affinity of said metals for functional groups present on the cell surface [21]. It has been found, for example, that in B. cereus the carboxyl, amide and phosphate groups have affinity for Cr6+, and the amide and carboxyl groups have affinity for Ni2+ [22]. Given that in this study the concentrations of Cr6+and Ni2+ were the highest, and that they share an affinity for the same functional groups, the competition between these two ions probably affected Cr6+reduction.
The Cr6+reduction rate showed an inverse relationship with respect to cell concentration (Fig. 1), mainly in the treatments with AWW and RWW with and without glucose, as was reported by Wang and Xiao [23]. This is because Cr6+ reduction can be inhibited by its toxicity, as it causes damage to DNA and interrupts normal cell replication [24]. However, some studies have shown that B. cereus exhibits resistance to multiple heavy metals (Pb, As, Hg, Zn, Co and Ni) [25], and despite a low growth rate, the cells that do grow are capable of continuously removing metals through internal detoxification mechanisms [26].
The toxic effects of RWW and AWW on the bacterium exposed to the different treatments can be observed in Fig. 2. In the control without Cr6+(Fig. 2a) the microorganism had an elongated shape and smooth surface, whereas in RWW (Fig. 2b-2c) cells became smaller, rounded and with some rugosities on the cell wall. Although in the AWW treatment (Fig. 2d-2e) the cell kept its elongated shape, there was a decrease in size and unequal edges on the walls.
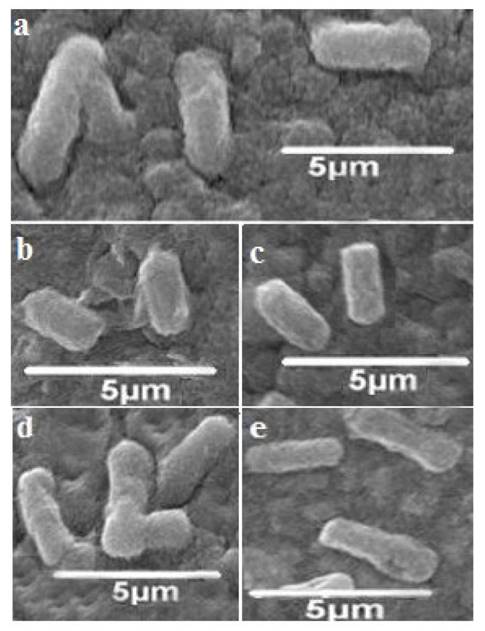
Figure 2 Scanning electron microscopy showing the effect on the morphologyand the size of B. cereus B1 cells: (a) control without Cr6+; (b) real wastewater; (c) real wastewater enriched with glucose; (d) artificial wastewater; (e) artificial wastewater enriched with glucose.
Although B. cereus B1 presented morphological changes in all treatments, the most drastic were observed in RWW. It seems these changes are the result of a resistance mechanism to medium toxicity [22]. Part of this mechanism consists in alterations of the cell membrane structure and size of the microbial cell [21], which are vital to sustain metabolic activity and to survive the absorption of chromium and other metals from the effluent [22].
It has also been determined that pH can strongly affect the properties of functional groups (carboxyl, phosphate, amine, among others) that are on the cell surface, and of metallic ions in solution [26]. As was reported by Liu et al. [19], we observed an increase in pH in the treatment with RWW (Table 3). The reason is that an electron donor is required (endogenous in this case) for the reduction of Cr6+ to Cr3+ , which results in a gradual decrease in the oxidation/reduction potential, and in consequence in a pH increase [27].
On the other hand, in the treatments with glucose we observed a pH decrease (Table 3). According to Chaturvedi [28], this occurs as a result of metabolite (organic acids) secretion by the cells. It has also been proposed that this pH decrease is the result of the formation and precipitation of chromium complexes [29]. It is therefore necessary to consider the role that glucose plays in bacterial metabolism, as well as the mechanisms implicated in the reduction and resistance to Cr6+[19].
Conclusions
This study reports on the ability of B. cereus B1 to reduce Cr6+ present in wastewaters of an electroplating plant, despite the toxicity that this type of effluent represented for this bacterium. Although the AWW was prepared using the same metals at similar concentrations to those found in RWW, the latter showed lower Cr6+ reduction efficiency than AWW, which demonstrates the existence of other components that interfere with the Cr6+ reduction process by B. cereus B1 in RWW.
Although treatments with glucose reached higher Cr6+ reduction efficiencies than those without glucose, Cr6+ concentrations of ± 0.14 mg/L were reached using RWW, a value which is within allowable levels (0.5 mg/L) set by Colombian regulations. This allows us to propose B. cereus B1 as a promising microorganism in the bioremediation of industrial effluents contaminated with Cr6+ .