Serviços Personalizados
Journal
Artigo
Indicadores
-
Citado por SciELO
-
Acessos
Links relacionados
-
Citado por Google
-
Similares em SciELO
-
Similares em Google
Compartilhar
Ciencia y Tecnología Agropecuaria
versão impressa ISSN 0122-8706
Corpoica cienc. tecnol. agropecu. vol.19 no.2 Mosquera maio/ago. 2018
https://doi.org/10.21930/rcta.vol19_num2_art:537
Plant physiology
Photosynthesis and biomass yield in Tabasco pepper, radish and maize subjected to magnetically treated water
1 PhD Student in Agricultural Sciences Universidad Nacional de Colombia, sede Palmira, Facultad de Ciencias Agropecuarias. Palmira, Colombia. Email: diospinas@unal.edu.co
2 Researcher, Universidad del Valle, Departamento de Física, Grupo de Investigación en Ciencias Ambientales y de la Tierra (Ilama) Cali, Colombia. Email: jhony.benavides@correounivalle.edu.co
3 Professor and Director, Universidad del Valle, Departamento de Física, Grupo de Investigación en Ciencias Ambientales y de la Tierra (Ilama). Cali, Colombia Email: orlando.zuniga@correounivalle.edu.co
4 Associate professor, Universidad Nacional de Colombia, sede Palmira, Facultad de Ciencias Agropecuarias. Email: cgmunozp@unal.edu.co
The aim of this study was to assess the effect of magnetically treated water (MTW) on physiological parameters of three species: Tabasco pepper, red radish and yellow maize. Half of the plants per species were irrigated with normal tap water and the other half with tap water treated with a magnetic device. Photosynthesis, biomass, and mineral content (the latter only in Tabasco pepper fruits) were measured. All the species grown under MTW increased their photosynthetic rate and stomatal conductance. Fluorescence parameters as Fv/Fm and non-photochemical quenching remained unchanged among the species tested. In Tabasco pepper, MTW produced higher yield measured as aerial biomass, fruits per plant and leaf area; moreover, nitrogen and divalent cation content in fruits increased as well.
In addition, there was a partial positive relation between leaf area and fruit yield (r2 = 0.52 for control and 0.30 for MTW). By contrast, there was an augment only in cob weight and grains per cob in maize plants, whereas radish plants showed a non-significant loss in total biomass. The higher biomass accumulation observed in Tabasco pepper and maize plants is attributed to a higher leaf area and/or carbon assimilation. Since chlorophyll fluorescence was unaffected, we propose that MTW does not trigger any change in the water-oxidizing complex of photosystem II (PSII). Moreover, the lack of response of several variables among the species tested showed that MTW might have interspecific effects. Despite the latter, this technology can be an alternative to improve crop yield, particularly in Tabasco pepper.
Keywords: agrotechnology; biomass; biophysics; crop yield; dry biomass; gas exchange; water conditioning
En este estudio se evaluó el efecto del agua tratada magnéticamente (ATM) en parámetros fisiológicos de ají Tabasco, rábano rojo y maíz amarillo. La mitad de las plantas de las tres especies se regaron con agua normal del acueducto, y la otra con agua tratada a través de un dispositivo magnético. Se midieron la fotosíntesis, la biomasa y el contenido mineral (este último solo en frutos de ají Tabasco). Todas las especies cultivadas con ATM aumentaron su tasa fotosintética y su conductancia estomática. Los parámetros de fluorescencia, como la fluorescencia variable (Fv/Fm) y la extinción no fotoquímica, permanecieron sin cambios en las especies evaluadas. En el ají Tabasco, el agua con tratamiento favoreció un mayor rendimiento, en parámetros como biomasa aérea, frutos por planta y área foliar, incrementándose también el contenido de nitrógeno y cationes divalentes en frutos. Además, hubo una relación parcialmente positiva entre el área foliar y el rendimiento de frutos por planta (r2 = 0,52 en el control y 0,30 con ATM). En contraste, en las plantas de maíz solo hubo un aumento en el peso y en los granos por mazorca, mientras que las de rábano mostraron una pérdida no significativa en la biomasa total. La mayor acumulación de biomasa observada en las plantas de ají Tabasco y maíz se atribuye a una mayor área foliar o a la asimilación de carbono. Debido a que la fluorescencia de la clorofila no se alteró, se propone que el atm no provoca ningún cambio en el complejo de oxidación del agua del fotosistema II. Por otra parte, la falta de respuesta de algunas variables en las tres especies mostró que el ATM puede tener efectos interespecíficos. A pesar de lo anterior, esta tecnología puede ser una alternativa para mejorar el rendimiento de los cultivos, en particular en del ají Tabasco.
Palabras clave: acondicionamiento de agua; agrotecnología; biomasa; biofísica; intercambio gaseoso; biomasa seca; rendimiento del cultivo
Introduction
Although the first impression one can have about water is that it does not have any magnetic response whatsoever, several investigations have shown that it actually does, but not due to an electron alignment phenomenon as in ferromagnetic substances (iron or nickel), because water has very low magnetic susceptibility. Instead, it is widely accepted that surface tension, evaporation rate and molecular clustering of water is affected in the presence of magnetic fields of different strengths (Chang & Weng, 2006; Otsuka & Ozeki, 2006; Pang & Deng, 2008). These processes are thought to be hydrogen bond-related (Cai, Yang, He & Zhu, 2009).
Researchers have proposed that the physicochemical changes in MTW might improve plant growth characteristics. For example, several authors have reported that prior exposure of irrigation water to magnetic fields leads to an increase in green and dry biomass, water use efficiency and mineral content (Abou El-Yazied, El-Gizawy, Khalf, El-Satar & Shalaby, 2012; Grewal & Maheshwari, 2011; Hozayn, Abd El Monem, Abdelraouf & Abdalla, 2013; Mahmood & Usman, 2014). Furthermore, some results indicate that deployment of MTW can alleviate the deleterious effect of limited or low-quality water supply in some crops. Ferrari et al. (2015) mentioned that lettuce plants supplied with half or a quarter of the water lost by evaporation, increased biomass (root weight and length) when using MTW, showing biomass yield close to that of well-watered plants. These results are consistent to what Aly, Thanaa, Osman and Abdelhamed (2015) mentioned, suggesting that MTW could be as effective as common anti-salinity substances (like ascorbic acid) to increase growth parameters and productivity of orange trees in desert conditions.
Photosynthetic rate, chlorophyll content and fluorescence are parameters that jointly indicate the physiological status and health of a plant, and how it is affected by experimental conditions. Thus, they are good predictors of crop development and productivity. Plants with lower chlorophyll status or yellowish leaves exhibit less efficient quantum yield in photosystems, and CO2 assimilation is inhibited (Dai et al., 2009). In this respect, Moussa (2011) showed that common beans grown under MTW exhibited higher photosynthetic activity, being consistent with a greater total chlorophyll content. Other authors (El-Sayed & El-Sayed, 2014) obtained similar results, showing also that there is an increase in total available carbohydrates in different plant organs of broad bean as a result of MTW application. Notwithstanding, the effect of this treatment on chlorophyll fluorescence parameters has not been studied thoroughly yet, and there is not a complete and uniform theory explaining the effects of MTW on plant metabolism. It is not unlikely that these effects are indirect, through a modification of the soil moisture profile, or by enhancing nutrient availability around the roots, as some works suggest (Khoshravesh, Mostafazadeh-Fard, Mousavi & Kiani, 2011; Maheshwari & Grewal, 2009; Noran, Shani & Lin, 1996). However, biological studies in vivo have shown that the permeability of ion channels into membranes and cells is increased, involving also calcium ion activity and ion concentration balance (Smith, McLeod, Liboff & Cooksey, 1987).
Although the magnetic treatment of water technology poses various advantages in improving crop production, there is still lack of information on the effect of this treatment on specific physiological processes. Therefore, the aim of this study was to assess the response of chlorophyll fluorescence and gas exchange parameters, as well as final biomass accumulation and mineral content, in three important plant species irrigated with water treated in a commercially available magnetic device. Plants under study were Tabasco pepper (Capsicum frutescens L. - Solanaceae), Crimson Giant red radish (Raphanus sativus L. - Brassicaceae) and ICA V-305 yellow maize (Zea mays L. - Poaceae).
Materials and methods
Study site, plant material and growing conditions
The experiment was conducted in a 125-m2 mesh house in the experimental farm station of Universidad del Valle, Cali, Valle del Cauca department, Colombia. The site is located at an altitude of 967 m a.s.l., with sub-humid tropical environmental conditions, with an annual average temperature of 26 °C and a relative humidity of 65 %. Seeds of Tabasco pepper were grown in trays with peat moss for 30 days to obtain vigorous and uniform plantlets for transplanting in pots. Seeds of red radish var. Crimson Giant and yellow maize var. ICA V-305 were sown directly in the pots. All pots contained 10 kg of clay loam topsoil previously homogenized and sifted, with a pH of 6.79 and an organic matter content of 2.57 %. A drip irrigation system was installed with 5 mm tubing connected to one emitter per pot with a flow rate of 3 L/h. Water was passed through the magnet at a flow rate of 2.8 L/min. The source of water was the municipal service company, having the same specifications as for drinking purpose. Irrigation was scheduled according to the reference evapotranspiration established for the crops assessed.
Magnetic treatment of water
Water was magnetically-treated using a Quantum Biotek (Omni Enviro Water Systems, Australia) magnet connected to the irrigation system before being derived to the drippers for each pot. This device is a twelve centimeters long polycarbonate cylinder with an internal diameter of one inch (2.54 centimeters). The magnetic induction was measured with an LD Didactic GmbH Teslameter (Huerth, Germany). The magnet generates a variable magnetic field from zero (0) to 156 mT (milli Tesla), that increases along the length and around the circumference of the cylinder. For further details of this device, see Grewal and Maheshwari (2011) .
Chlorophyll fluorescence determination
Chlorophyll fluorescence measurements were carried out in the first three fully expanded, sun-exposed young leaves, once a week in each plant during the growing season of each crop. This property was measured using an OS30p+ fluorometer (Opti-Sciences Inc., USA) for light and dark-adapted measurements. Dark adaptation was achieved by covering the leaves for 30 minutes with suitable clips. Fluorescence parameters were calculated according to Baker (2008) as follows:
Gas exchange, biomass accumulation and elemental composition
Photosynthetic rate (A), transpiration (E) and stomatal conductance (Gs) were measured in the same three fully-expanded, sun-exposed, young leaves, using a CI-340 handheld photosynthesis system (CID Bio-Science, USA). Measurements were carried out between 11:00 and 15:00 hours under natural light, humidity, CO2 atmospheric concentration and temperature conditions, during the growing season of each crop. Light intensity was recorded at each sampling time with a LI-190SA Quantum Sensor adapted to a LI-1400 Data Logger (LI-COR, USA). Water use efficiency (WUE) was calculated dividing CO2 assimilation by its corresponding H2O transpiration.
For biomass accumulation, radish and maize plants were harvested 40 days and 90 days after sowing, respectively, weighing apart the rhizomes and leaves in the case of radish, and the total cob, the caryopses and the naked cob in the case of maize. For Tabasco pepper, fruits were harvested four times from the fifth until the eighth month after transplanting. Dry fruits were sent to Agrilab laboratories in Bogotá, Colombia, for further mineral composition analysis. After this, the above-ground parts of the plants (stems and leaves) were separated. All the samples of the three species were fresh and dry weighed; dry weigh was measured after drying the samples in an oven at 65 °C for 72 hours. Leaf area (LA) in radish and Tabasco pepper was estimated from the dry weight of a leaf disc of 0.81 cm of radius and extrapolating to total dry weight of leaves. Furthermore, the leaf area ratio (LAR) of Tabasco pepper was calculated in relation to dry weight of fruits (equation 1), since fruits are a sink of photoassimilates, and leaves are the source of these. For radish, the calculus was done considering the entire plant dry weight (equation 2), according to the following equations:
(Equation 1)
Leaf area ratio (LAR) for Tabasco pepper
= LA/DWF (cm2/g)
(Equation 2)
Leaf area ratio (LAR) for radish
= LA/TDW (cm2/g)
Where:
DWF: dry weight fruits
TDW: total dry weight
Please note that leaf area and leaf area ratio was not calculated for maize because insects damaged many leaves and this was finally not possible.
Experimental design and statistical analysis
The experiment was settled in a completely randomized design with 38 replicates per treatment (magnetically treated water or MTW) and control (non-treated water) in Tabasco pepper and radish, while 21 replicates were used for maize. One plant in each pot was considered a single replicate. Data was analyzed with the statistical software IBM SPSS Statistics 22, running previously a homogeneity test of variances and normality. A general linear model procedure for repeated measurements was carried out for chlorophyll fluorescence parameters, testing significance between and within subjects. For one single measurements (i.e. final biomass), a mean comparison test was applied. Only the data for gas exchange variables at the same light intensities were included in the statistical analysis.
Results and discussion
Fluorescence parameters and chlorophyll content
The interaction between different chlorophyll fluorescence measurements of plants with MTW was consistently similar to those found in control plants. Maximum fluorescence (Fm) of dark-adapted leaves in maize was slightly higher in treated plants. As for minimum fluorescence (F0) and non-photochemical quenching (NPQ), all the values for MTW and control within the three species were practically the same. Regarding maximum quantum efficiency of PSII photochemistry (Fv/Fm), only radish showed a minimal decrease, whereas in quantum yield base line (F0/Fm) the opposite effect was observed. Moreover, the interaction between all the previous parameters among the three species was found to be strongly significant (table 1).
Table 1 Chlorophyll fluorescence parameters in Tabasco pepper, Crimson Giant red radish and ICA V-305 maize according to treatments
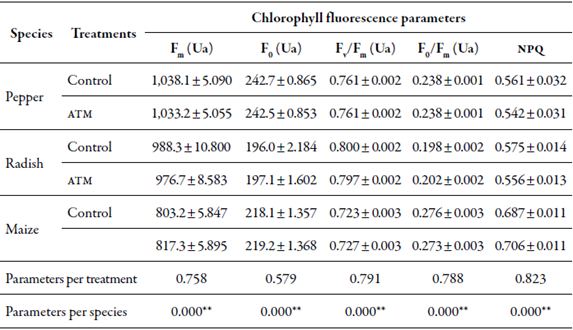
** p < 0.05. All the values are means ± standard error of 38 plants for Tabasco pepper and radish, and 21 plants for maize. MTW: magnetically treated water. Au: absorbance units; NPQ: non-photochemical quenching.
Source: Elaborated by the authors
Researchers have acknowledged chlorophyll a fluorescence emission as a reliable indicator of plant stress and photosynthetic performance (Baker, 2008). In this study, we report that the interaction between all the five fluorescence parameters measured (Fm, F0, Fv/Fm, F0/Fm and NPQ) and the treatment with MTW were not statistically different from that of the control. From this outcome, we can infer that this treatment is not a source of stress by itself, since the Fv/Fm values for maize (0.727) and radish (0.800) were close to the normal range for non-stressed C4 and C3 plants (0.780 and 0.830 respectively) (Pfündel, 1998). However, our Tabasco pepper readings for Fv/Fm (0.761) were lower than those reported (0.809) for pepper plants under different cultivation methods (Del Amor, 2006). This indicates that the conditions in the mesh house could be mildly harsh particularly for this species.
Despite the latter, NPQ did not exhibit a significant change among the three species tested with MTW, which means that there was no increase in excess of light quenching through heat dissipation as a result of this treatment; this would have been a stress measure. In addition, it has previously been reported that an impairment or promotion in the water-splitting process in PSII should be noticeable in fluorescence yield. For example, tobacco mutant plants that lack a specific polypeptide in their PSII water-splitting apparatus exhibits higher F0 values and almost zero variable fluorescence (F) values (Hager, Hermann, Biehler, Krieger-Liszkay & Bock, 2002). As long as no significant differences were observed in the fluorescence parameters between the control and the plants treated, we suggest that the metabolic magnetically-treated water is not susceptible to differential splitting in the H2O-oxidizing complex.
Biomass accumulation and yield
Final aerial biomass and yield of Tabasco pepper fruits in plants subjected to MTW was consistently higher in comparison with the control plants. Fresh weight of fruits per plant and number of fruits per plant were greater at a significant level in treated plants. The increase in these parameters was 27 % and 31 %, respectively, whereas the increase in fruit dry weight was 18 % (figure 1a). Fresh and dry weight of leaves and stems also increased in plants grown with MTW, but at a lower level of significance, ranging from 13 % for fresh weight of leaves (FWL), 15 % for dry weight of leaves (DWL), 14 % for fresh weigh of stems (FWS) and 12 % for dry weight of stems (DWS) (figure 1b). Leaf area (LA) and leaf area ratio (LAR) suffered an increase (15 %) and a decrease (5.5 %), respectively (figure 1c and 1d).
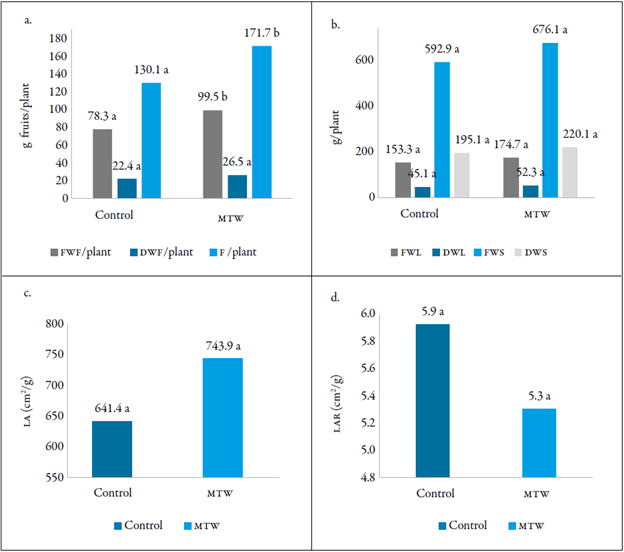
Figure 1 Biomass accumulation in Tabasco pepper plants. a. Fruit yield. FWF: fresh weight fruits (sig. = 0.034**). DWF: dry weight fruits (sig. = 0.125). F/plant: fruits per plant (sig. = 0.018**); b. Aerial final biomass. FWL: fresh weight leaves (sig. = 0.274). DWL: dry weight leaves (sig. = 0.214). FWS: fresh weight stems (sig. = 0.208). DWS: dry weight stems (sig. = 0.197); c. Leaf area. LA: leaf area (sig. = 0.214); d. Leaf area ratio. LAR: leaf area ratio (sig. = 0.352). All values are means of 39 plants.
In addition, leaf area and fruit dry weight showed a positive relation from a linear model by least squares (figure 2) in both treated and untreated plants. However, despite the larger leaf area observed in Tabasco pepper plants subjected to MTW, this variable did not explain completely the variation in dry biomass accumulation in fruits, since the coefficient of determination (r 2) only reached 29.89 % of the variation. In contrast, this value for the control plants (52.16 %) was almost double compared to the one found in treated plants.
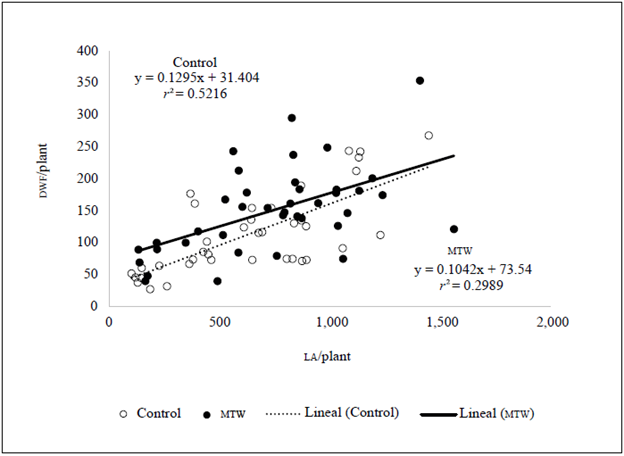
Source: Elaborated by the authors
Figure 2 Variation in dry weight of fruits (DWF) per plant in relation to leaf area (LA) in Tabasco pepper plants.
The analysis of the element composition in dry fruits of Tabasco pepper showed that several nutrients increased their concentration under MTW, when compared with the control plants (table 2). Among these, nitrogen, calcium, magnesium, sulfur, manganese and zinc exhibited the highest increases, being significant the values of nitrogen and zinc. Potassium and boron remained practically equal in both groups of plants. Phosphorus, iron, copper and sodium underwent decreases with MTW, but at lower levels of significance. The increase in Ca and Mg was also observed in the saturation of these cations, showing higher values in MTW, except for potassium, which exhibited lower saturation. All the ratios between cations in MTW were higher, and so did the N/P and Ca/B ratios. However, N/S ratio was almost the same between the two groups of plants, and the Fe/Mn ratio showed a reduction.
Table 2 Element composition of dry Tabasco pepper fruits
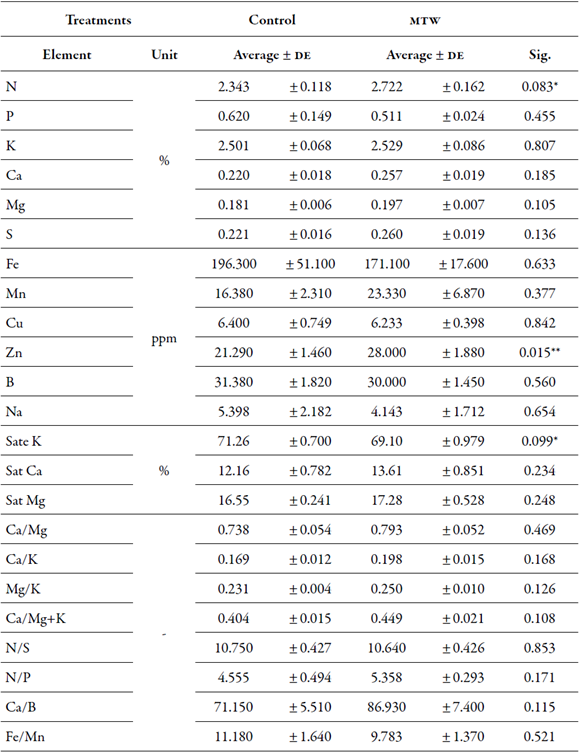
*p < 0.1; **p < 0.05. Data are mean values of nine samples. Concentration units are in function of dry weight.
Source: Elaborated by the authors
On the other hand, in Crimson Giant red radish plants, an opposite trend was observed regarding biomass accumulation. The final biomass in both leaves and stems (including the rhizomes) underwent a decrease in plants grown with MTW. However, this trend was not as significant as the increase in biomass observed in Tabasco pepper plants (figure 3a). Leaf area and leaf area ratio of radish plants with non-treated water were also slightly higher compared to treated plants (figure 3b).
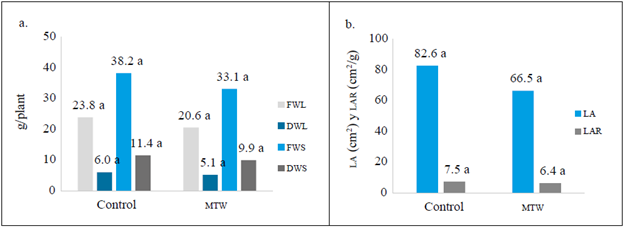
Figure 3 Biomass accumulation in Crimson Giant red radish plants. a. Total final biomass. FWL: fresh weight leaves (sig. = 0.858). DWL: dry weight leaves (sig. = 0.859). FWS: fresh weight stems (sig. = 0.863). DWS: dry weight stems (sig. = 0.863); b. Leaf area and leaf area ratio. LA: leaf area (sig. = 0.791). LAR: leaf area ratio (sig. = 0.680). All values are means of 39 plants.
Regarding cob yield of ICA V-135 maize plants, there was a similar effect produced by MTW as the one observed in Tabasco pepper plants, since total cob weight and number of grains per cob raised under this treatment; however, the sole weight of one hundred grains was practically equal to values found in control plants (figure 4). Increase in cob weight and grains per cob was 13 % and 5.3 %, respectively.
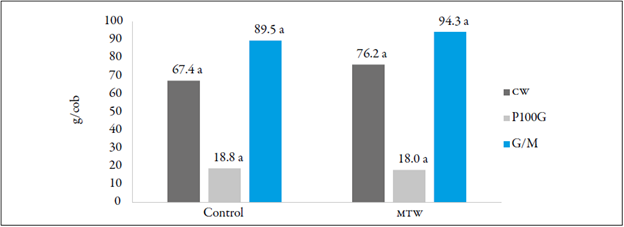
Source: Elaborated by the authors
Figure 4 Cob harvest in ICA V-135 maize plants. CW: cob weight (sig. = 0.127). W100G: weight of 100 grains (sig. = 0.157). G/C: grains per cob (sig. = 0.762). All values are means of 21 plants.
The effect of MTW on biomass accumulation was only consistent in Tabasco pepper and ICA V-135 maize plants. The higher biomass yields reported in treated plants in this study agrees with results obtained by previous authors who used the same magnetic device in celery and snow peas (Maheshwari & Grewal, 2009). The highest significant effect of MTW was found in Tabasco pepper plants observed in number and fresh weight of fruits per plant, although both fresh and dry weight of leaves and stems also increased; this in turn affected LA and LAR. Since green, light-exposed leaf area is the source of photoassimilates for the entire plant, this feature is frequently related with biomass accumulation in other plant organs. Authors as Graber et al. (2010) observed this relation in sweet pepper grown in a biochar substrate. Higher leaf area means higher light interception, which is a very important factor affecting plant growth, even more than net photosynthesis (Kang & Van Iersel, 2004). However, when contrasting fruit yield with LA by linear regression there was a partial adjustment. From this outcome, it is evident that dry biomass accumulation in fruits was only in part correlated with leaf area, particularly in plants subjected to MTW. We attribute this difference to the effect of the treatment, which leads to further physiological mechanisms triggering this response. Hence, it is possible that not only LA, but also net photosynthesis have helped increase the number and weight of Tabasco pepper fruits. This is consistent with the lower LAR values found in these plants, which was a consequence of the higher dry weight of fruits, rather than of a decreased leaf area. Viewing this from another angle, this means that Tabasco pepper plants subjected to MTW created more dry fruit biomass per LA unit, as long as fruits are a sink of photoassimilates.
By contrast, the decrease in leaf area and leaf area ratio observed in Crimson Giant red radish plants under MTW was associated with less fresh and dry biomass in leaves and stems, although this effect was found to be not significant at a reasonable level (p > 0.680). The pattern observed in ICA V-305 maize plants were that cob weight and grains per cob in treated plants were superior to those of their control counterparts; this indicates that an increase in cob weight was mainly due to the development of more grains per cob, instead of an increase in biomass accumulation in the caryopses. This is an important finding, since in maize these parameters are very sensitive to crop density, and are more likely to decrease when increasing plant population per area unit (Bavec & Bavec, 2002). Hence, we suggest that there could be a positive interaction between MTW and different crop densities in relation with maize yield.
Moreover, different patterns in element composition in fruits of Tabasco pepper indicates that MTW enhances the uptake of several of these, particularly N and Zn. Although we did not carry out an analysis of capsaicin, the higher nitrogen concentration might indicate a greater alkaloid (capsaicin) or protein content. In pepper, cation uptake (K, Ca, Mg and Na) and its concentration in shoots and roots is affected by the nitrogen source and salinity levels of the substrate (Huez-López, Ulery, Samani, Picchioni & Flynn, 2011). Here, we report that the percentage of Ca and Mg were always higher in treated plants, and Na decreased by more than 20 % without any addition of fertilizers. These results are contrary to those reported by Maheshwari and Grewal (2009) , who found a reduction in Ca and Mg and an increase in Na in snow pea pods, using the same magnetic device with potable water. At any rate, our findings suggest that there is a higher uptake of divalent cations as Ca, Mg and Zn (which have lower mobility than monovalent cations) at the expense of Na; this explains why K saturation was lower, although the concentration of this cation was not reduced.
Gas exchange measurements
Photosynthetic rate (A) in the three species evaluated exhibited an incremental behavior under irrigation with MTW. This effect was significant in ICA V-305 maize plants, and only slightly higher in Crimson Giant red radish and Tabasco pepper. This increase in A was associated with a higher transpiration, particularly in ICA V-305 maize and Tabasco pepper plants. Concomitant with the latter effects, stomatal conductance suffered an increase in all species under MTW, although this response was not significant. Despite the increase in H2O released from the leaves, water use efficiency within the three species tested did not undergo any significant reduction or increase in treated plants in comparison with the controls (table 3).
Table 3 Gas exchange parameters in Tabasco pepper, Crimson Giant red radish and ICA V-305 maize according to treatments
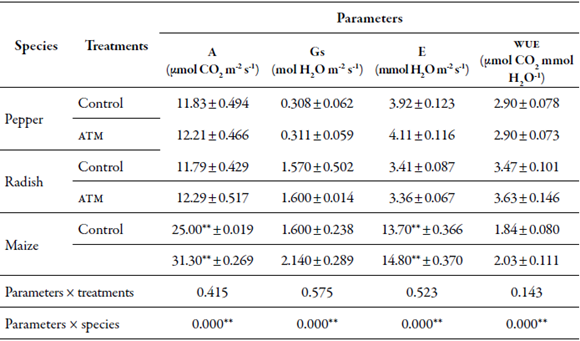
**p < 0,05. MTW: magnetically treated water. A: photosynthetic rate. Gs: stomatal conductance. E: transpiration. WUE: water use efficiency. All the values are means of 38 plants for Tabasco pepper and radish, and 21 plants for maize. Source: Elaborated by the authors
Biomass accumulation increase in plants under MTW could be consequence of higher CO2 uptake, particularly in ICA V-305 maize plants. Since this parameter is strongly correlated with stomatal conductance and transpiration, we ascribe the augment of the photosynthetic rate to a greater stomata opening observed in this species. How MTW elicits this effect is a question that is based in the understanding of the physical interaction between water and the stomatal system. Several authors have reported that MTW has higher evaporation rate (Guo et al., 2012; Szczes, Chibowski, Hohysz & Rafalski, 2011). Nonetheless, considering that higher transpiration from water to vapor was also observed in ICA V-305 maize plants subjected to MTW in this study, we suggest that this evaporative force triggers a wider stomatal opening, which in turn leads to higher CO2 intake.
This could explain why water use efficiency did not decrease with this treatment, and as long as it is concomitant with major transpiration, there will be a higher photosynthetic rate. However, we cannot ignore the possibility that this treatment might increase water depletion in the soil in a faster way. The lack of response of gas exchange parameters to MTW in Crimson Giant red radish plants explains why biomass yield per plant were not affected.
Conclusions
Irrigation effects with MTW on Tabasco pepper, Crimson Giant red radish and ICA V-305 maize were not consistent among these species in relation to the biomass accumulation and physiological parameters tested. Regarding chlorophyll fluorescence, no effects due to MTW were observed. This has two interpretations. The first one is that this treatment is not a source of stress per se that can be noticeable from changes in fluorescence yield, and the second one is that the intrinsic physical changes of MTW do not produce any particular interaction at the molecular level with the water-oxidizing complex in the PSII, which was a possible hypothesis. However, the greater biomass obtained in Tabasco pepper fruits and ICA V-305 maize cobs indicate that higher photosynthetic rate and larger leaf area observed in maize and pepper plants respectively, could have helped in this response. Despite an increase in transpiration, water use efficiency did not decrease because of the higher CO2 uptake. The lack of significant responses of Crimson Giant red radish to MTW demonstrates that not all species react to this treatment in the same way. Notwithstanding, the positive response of Tabasco pepper in relation to nutrient uptake and fruit yield allow us to propose irrigation with MTW as an easy and simple technique to improve its cultivation.
To cite this article: Ospina-Salazar, D. I., Benavides-Bolaños, J. A., Zúñiga-Escobar, O., & Muñoz-Perea, C. G. (2018). Photosynthesis and biomass yield in Tabasco pepper, radish and maize subjected to magnetically treated water Corpoica Ciencia y Tecnología Agropecuaria, 19(2), 307-321.
Disclaimers: This work was possible thanks to funding of the project "Implementation of a competitive rural agroindustrial system in a bioregion of Valle del Cauca". The authors declare that they do not have any contractual, family or economic relationship with Omni Enviro Water Systems.
REFERENCES
Abou El-Yazied, A., El-Gizawy, A. M., Khalf, S. M., El-Satar, A., & Shalaby, O. A. (2012). Effect ofmagnetic field treatments for seeds and irrigation water as well as N, P and K levels on productivity of tomato plants. Journal of Applied Sciences Research, 8(4), 2088-2099. Recuperado de http://www.aensiweb.com/old/jasr/jasr/2012/2088-2099.pdf. [ Links ]
Aly, M. A., Thanaa, M. E., Osman, S. M., & Abdelhamed, A. A. (2015). Effect of magnetic irrigation water and some anti-salinity substances on the growth and production of Valencia orange. Middle East Journal of Agriculture Research, 4(1), 88-98. Recuperado de http://www.curresweb.com/mejar/mejar/2015/88-98.pdf. [ Links ]
Baker, N. R. (2008). Chlorophyll fluorescence: a probe of photosynthesis in vivo. Annual Review of Plant Biology, 59, 89-113. doi:10.1146/annurev.arplant.59.032607.092759. [ Links ]
Bavec, F. & Bavec, M. (2002). Effects of plant population on leaf area index, cob characteristics and grain yield of early maturing maize cultivars (FAO 100-400). European Journal of Agronomy, 16(2), 151-159. doi:10.1016/S1161-0301(01)00126-5. [ Links ]
Cai, R., Yang, H., He, J., & Zhu, W. (2009). The effects of magnetic fields on water molecular hydrogen bonds. Journal of Molecular Structure, 938(1-3), 15-19. doi:10.1016/j.molstruc.2009.08.037. [ Links ]
Chang, K. T. & Weng, C. I. (2006). The effect of an external magnetic field on the structure of liquid water using molecular dynamics simulation. Journal of Applied Physics, 100(4), 1-6. doi:10.1063/1.2335971. [ Links ]
Dai, Y., Shen, Z., Liu, Y., Wang, L., Hannaway, D., & Lu, H. (2009). Effects of shade treatments on the photosynthetic capacity, chlorophyll fluorescence, and chlorophyll content of Tetrastigma hemsleyanum Diels et Gilg. Environmental and Experimental Botany, 65(2-3), 177-182. doi:10.1016/j.envexpbot.2008.12.008. [ Links ]
Del Amor, F. (2006). Growth, photosynthesis and chlorophyll fluorescence of sweet pepper plants as affected by the cultivation method. Annals of Applied Biology, 148(2), 133-139. doi:10.1111/j.1744-7348.2006.00048.x. [ Links ]
El-Sayed, H. & El-Sayed, A. (2014). Impact of magnetic water irrigation for improve the growth, chemical composition and yield production of broad bean (Vicia faba L.) plant. American Journal of Experimental Agriculture, 4(4), 476-496. Recuperado de http://www.sciencedomain.org/abstract/2942. [ Links ]
Ferrari, F., Almeida, L., Klar, A., Ferreira da Silva, J., Pires, C., & Ludwig, R. (2015). Response of lettuce crop to magnetically treated irrigation water and different irrigation depths. African Journal of Agricultural Research, 10(22), 2300-2308. Recuperado de http://www.academicjournals.org/article/article1433520416_Putti%20et%20al.pdf. [ Links ]
Graber, E. R., Meller-Harel, Y., Kolton, M., Cytryn, E., Silber, A., Rav-David, D. , ... Elad, Y. (2010). Biochar impact on development and productivity of pepper and tomato grown in fertigated soilless media. Plant and Soil, 337(1), 481-496. doi:10.1007/s11104-010-0544-6. [ Links ]
Grewal, H. S. & Maheshwari, B. L. (2011). Magnetic treatment of irrigation water and snow pea and chickpea seeds enhances early growth and nutrient contents of seedlings. Bioelectromagnetics, 32(1), 58-65. doi:10.1002/bem.20615. [ Links ]
Guo, Y. Z., Yin, D. C., Cao, H. L., Shi, J. Y., Zhang, C. Y., Liu, Y. M., ... Shang, P. (2012). Evaporation rate of water as a function of a magnetic field and field gradient. International Journal of Molecular Sciences, 13(12), 16916-16928. doi:10.3390/ijms131216916. [ Links ]
Hager, M., Hermann, M., Biehler, K., Krieger-Liszkay, A., & Bock, R. (2002). Lack of the small plastid-encoded PsbJ polypeptide results in a defective water-splitting apparatus of photosystem II, reduced photosystem I levels, and hypersensitivity to light. Journal of Biological Chemistry, 277(16), 14031-14039. doi:10.1074/jbc.M112053200. [ Links ]
Hozayn, M., Abd El Monem, A. A., Abdelraouf, R. E., & Abdalla, M. (2013). Do magnetic water affect water efficiency, quality and yield of sugar beet (Beta vulgaris L.) plant under arid regions conditions? Journal of Agronomy, 12(1), 1-10. doi:10.3923/ja.2013.1.10. [ Links ]
Huez-López, M. A., Ulery, A. L., Samani, Z., Picchioni, G., & Flynn R. P . (2011). Response of chile pepper (Capsicum annuum L.) to salt stress and organic and inorganic nitrogen sources: III. Ion uptake and translocation. Tropical and Subtropical Agroecosystems, 14(3), 765-776. Recuperado de http://www.redalyc.org/articulo.oa?id=93921493009. [ Links ]
Kang, J. G. & Van Iersel, M. W. (2004). Nutrient solution concentration affects shoot: root ratio, leaf area ratio, and growth of subirrigated salvia (Salvia splendens). HortScience, 39(1), 49-54. Recuperado de http://hortsci.ashspublications.org/content/39/1/49.full.pdf+html. [ Links ]
Khoshravesh, M., Mostafazadeh-Fard, B., Mousavi, S. F., & Kiani, A. R. (2011). Effects of magnetized water on the distribution pattern of soil water with respect to time in trickle irrigation. Soil Use and Management, 27(4), 515-522. doi:10.1111/j.1475-2743.2011.00358.x. [ Links ]
Maheshwari, B. L. & Grewal, H. S. (2009). Magnetic treatment of irrigation water: its effects on vegetable crop yield and water productivity. Agricultural Water Management, 96(8), 1229-1236. doi:10.1016/j.agwat.2009.03.016. [ Links ]
Mahmood, S. & Usman, M. (2014). Consequences ofmagnetized water application on maize seed emergence in sand culture. Journal of Agricultural Science and Technology, 16(1), 47 55. Recuperado de http://citeseerx.ist.psu.edu/viewdoc/download?doi=10.1.1.837.9646&rep=rep1&type=pdf. [ Links ]
Moussa, H. R. (2011). The impact of magnetic water application for improving common bean (Phaseolus vulgaris L.) production. New York Science Journal, 4(6), 15-20. [ Links ]
Noran, R., Shani, U., & Lin, I. (1996). The effect of irrigation with magnetically treated water on the translocation of minerals in the soil. Magnetic and Electrical Separation, 7(2), 109-122. Recuperado de https://www.hindawi.com/journals/psse/1996/046596/abs/. [ Links ]
Otsuka, I. & Ozeki, S. (2006). Does magnetic treatment of water change its properties ? The Journal of Physical Chemistry B, 110(4), 1509-1512. doi:10.1021/jp056198x. [ Links ]
Pang, X. F. & Deng, B. (2008). Investigation of changes in properties of water under the action of a magnetic field. Science in China Series G: Physics, Mechanics and Astronomy, 51(11), 1621-1632. doi:10.1007/s11433-008-0182-7. [ Links ]
Pfündel, E. (1998). Estimating the contribution of photosystem I to total leaf chlorophyll fluorescence. Photosynthesis Research, 56(2), 185-195. doi:10.1023/A:1006032804606. [ Links ]
Smith, S., McLeod, B. R., Liboff, A. R., & Cooksey, K. (1987). Calcium cyclotron resonance and diatom motility. Bioelectromagnetics , 8(3), 215-227. doi:10.1002/bem.2250080302. [ Links ]
Szcześ, A., Chibowski, E., Holysz, L., & Rafalski, P. (2011). Effects of static magnetic field on water at kinetic condition. Chemical Engineering and Processing: Process Intensification, 50(1), 124-127. doi:10.1016/j.cep.2010.12.005. [ Links ]
Received: October 27, 2016; Accepted: February 26, 2018