Introduction
Fish oil is an industrial product of great nutritional value due to its content of long chain omega-3 polyunsaturated fatty acids (PUFA), such as docosahexaenoic acid (DHA), docosapentaenoic acid (DPA) and eicosapentaenoic acid (EPA), which are currently highly valued for their prophylactic and therapeutic properties in nutritional and health fields. Fish oil, which was previously a by-product of fishmeal used for animal feed, is now recognized as the primary source of these fatty acids (Valenzuela, Sanhueza, & De la Barra, 2012).
EPA and DHA content in fish oil is an important quality parameter of this product. These fatty acids are related to different neuronal functions, and their absence is associated with diverse inflammatory processes and the precarious development of neurons in human patients. Likewise, its beneficial effects in cardiovascular diseases are recognized (Coronado, Vega y León, Gutiérrez, García, & Díaz, 2006).
Fish oil can be obtained from different species depending on the production area. The raw material is comprised of three major fractions, which include solids, oil and water. The goal is to separate these components as best as possible, commonly obtaining fishmeal and fish oil (United Nations Food and Agriculture Organization [FAO], 1986). Methods to extract them include cooking, use of solvents and, recently, extraction by supercritical fluids, by enzymatic procedures and by chemical (i.e. applying acids) or biological silages (Mbatia et al., 2010; Menegazzo, Petenuci, & Fonseca, 2014).
The crude oil contains impurities, which depend on the extraction method used (Chakraborty & Joseph, 2015a), and requires a purification process to reach quality features that make it acceptable for human consumption (Crexi, Legemann-Monte, Almeida de Souza, & De Almeida-Pinto, 2010).
To achieve such characteristics, different impurities must be eliminated while maintaining the most desirable compounds such as omega-3 and other PUFAs, so the refining process is designed to achieve this, minimizing oil losses and maximizing the availability of beneficial constituents (Vaisali, Charanyaa, Belur, & Regupathi, 2015).
The interest in obtaining higher good quality PUFA concentration is evident in several investigations that aim at extracting fish oil, purifying it and increasing its PUFA content, especially EPA and DHA, using different techniques. Regarding PUFA some studies include extraction and fractionation (Rubio et al., 2010; Sahena et al., 2009), but they focus on supercritical fluid technology and only cover studies carried out until 2009.
Therefore, the objective of this study is to carry out a general review of the progress that has been made since year 2000 regarding different fish oil extraction technologies, as well as the advances in refining and fractionation, focused on the conservation and enrichment in omega-3 PUFAs.
Fish oil extraction
Several studies have been developed around the extraction and quality analysis of oil obtained from different fish species, as well as by-products of their processing, in which different techniques are used, such as supercritical fluids, wet pressing, extraction using solvents, and fish silage employing enzymes present in fish or from other sources (Adeoti & Hawboldt, 2014).
The extraction of fish oil by wet pressing is the most commonly used method for production on an industrial scale, and is basically carried out in four stages: fish cooking, pressing, decantation and centrifugation (FAO, 1986).
Drastic temperature and pressure conditions used for protein coagulation and subsequent oil release may partially modify the PUFAs present, due to degradation reactions such as hydrolysis and oxidation (Linder, Fanni, & Parmentier, 2005; Mbatia et al., 2010). Table 1 shows studies that have been carried out using wet pressing in the laboratory, comparing this technique with other methods and including further test conditions.
Another conventional procedure is the extraction using solvents, applied generally for analytical purposes but not for industrial production, because of the disadvantages of using substances with restrictions in the food industry (Rubio et al., 2010). This process is based on the solubility of lipids in organic solvents and their insolubility in water, thanks to which these, as well as its soluble components such as proteins, carbohydrates and minerals, can be separated from water.
The main limitations of this technique are that it requires a relatively dry sample that is destroyed, and it takes a long time, in addition to generating large amounts of residual solvent (Adeoti & Hawboldt, 2014; Sahena et al., 2009).
Several methods vary according to the type of solvent or treatment used on the sample. The most common are the ones of Soxhlet and Bligh-Dyer, however, others such as McGill-Moffatt and the one of Randall and Folch have also been evaluated (Fiori et al., 2012; Immanuel et al., 2009; Rincón-Cervera, Villarreal-Rubio, Valenzuela, & Valenzuela, 2017).
These methods have been applied for the extraction of fish oil as the sole technology used (Adeniyi & Bawa, 2006; Boran, Karaçam, & Boran, 2006; Kołakowska, Domiszewski, Kozłowski, & Gajowniczek, 2006; Shamsudin & Salimon, 2006). Moreover, these have also been used in comparison to other methods (Chakraborty & Joseph, 2015a; Fiori et al., 2012; Immanuel et al., 2009; Létisse, Rozières, Hiol, Sergent, & Comeau, 2006; Rincón-Cervera et al., 2017; Sahena et al., 2010; Silva-Aguiar & Soares-Goulart, 2013) (table 2)
Supercritical fluid extraction
Supercritical fluid extraction (SFE) is an emerging extraction technology using solvents that has gained great interest in recent years, due to advantages such as the use of a moderate temperature, an oxygen- free environment and extraction of low polarity lipids, which avoids the extraction of impurities (Rubio et al., 2012).
Supercritical fluids have a lower viscosity and a higher diffusivity than conventional solvents, improving mass transfer and, in general, reduces the time needed for extraction. Its biggest disadvantage is the high cost of applying this technology at an industrial level. The fluid that is mostly used is CO2; it is employed as an inert solvent and is safe for oil extraction. Its main advantage is that it does not remain in the product, since at room temperature and pressure it returns to its gas state and evaporates (Rubio et al., 2010, 2012).
The parameters that are usually evaluated are pressure, temperature, CO2 flow and time. Yet, the effect of moisture, flow direction, solvent: material ratio, and particle size have also been studied in fish oils (Rubio et al., 2008), and the process has been modeled to find optimal conditions (Ferdosh et al., 2013; Sarker et al., 2012).
Other studies, which compare SFE in fish oils with other extraction techniques (table 3) (Ferdosh, Sarker, Norulaini, Oliveira et al., 2014; Fiori et al., 2012; Hao et al., 2015; Rubio et al., 2012; Sahena et al., 2010) show that this technology is attractive in conventional processes, to obtain oils rich in PUFA and with a reduced content of contaminants.
Extraction by fish silage
Fish silage is a semi-liquid product made from whole fish or parts of fish, to which acids (chemical silage), enzymes (silage or enzymatic extraction) or lactic acid bacteria (biological silage) are added, causing protein hydrolysis (Ferraz de Arruda, Borghesi, & Oetterer, 2007).
This process has been shown as a good alternative to traditional methods, since it can be simpler and cheaper in terms of investment costs and energy expenditure. In addition, this technology does not use solvents or employs high temperatures, and the physicochemical and microbiological changes caused can not only improve extraction performance, but also prevent undesirable processes, i.e. fat oxidation. Furthermore, essential fatty acids and other functional ingredients, such as protein hydrolysate and collagen, among others can be recovered (Ferraz de Arruda et al., 2007; Rai, Swapna, Bhaskar, Halami, & Sachindra, 2010; Rubio et al., 2010).
Some studies have focused on the evaluation of this procedure for the extraction of oil from whole fish or its by-products (table 4). One of the most studied techniques is enzymatic silage with different types of proteases, but biological and chemical silages are also used in various investigations for the separation of fish oil.
Fish oil refining
Once fish oils are extracted these require a purification process to achieve the quality characteristics that make it acceptable for human and animal consumption (Crexi et al., 2010), since they contain insoluble impurities, phospholipids, free fatty acids, moisture, primary oxidation products, minerals, pigments and even persistent organic pollutants (POP).
Impurities in the oil reduces its quality (Huang& Sathivel, 2010) and must be eliminated while maintaining the most desirable compounds, such as omega-3 and other PUFA, so the refining process must be designed in such a way that this goal is achieved, minimizing oil loss and maximizing the availability of beneficial constituents (Vaisali et al., 2015).
The traditional refining process includes several stages, such as degumming, neutralization, bleaching, deodorization and, in some cases, winterization, although this could be considered to a greater extent a PUFA concentration method. Each of the stages is especially important to remove the different classes of compounds (table 5) and is the most studied and industrially applied process for the refining of fish oils.
Table 5 Minor oil components, effect on the quality and refining stage for its removal
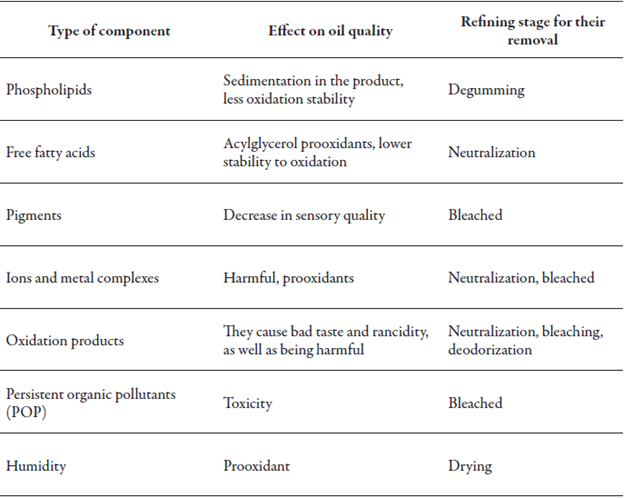
Source: Elaborated by the authors
Several studies have been carried out to evaluate the effect of the refining process on different oil properties, through the establishment of parameters such as acidity, peroxides, thiobarbituric acid, iodine, saponification and anisidine indexes, which are some of the most important ones.
However, in all assessments, the peroxide (PI) and acidity (IA) indexes are included as essential indicators in the evaluation process (table 6). Furthermore, in most cases, these are negatively affected in the stages in which exposure to high temperatures is higher, such as neutralization, drying and deodorization, because it increases the susceptibility to oxidation and the formation of peroxides (Crexi et al., 2010).
Table 6 Evaluation parameters in fish oil refining
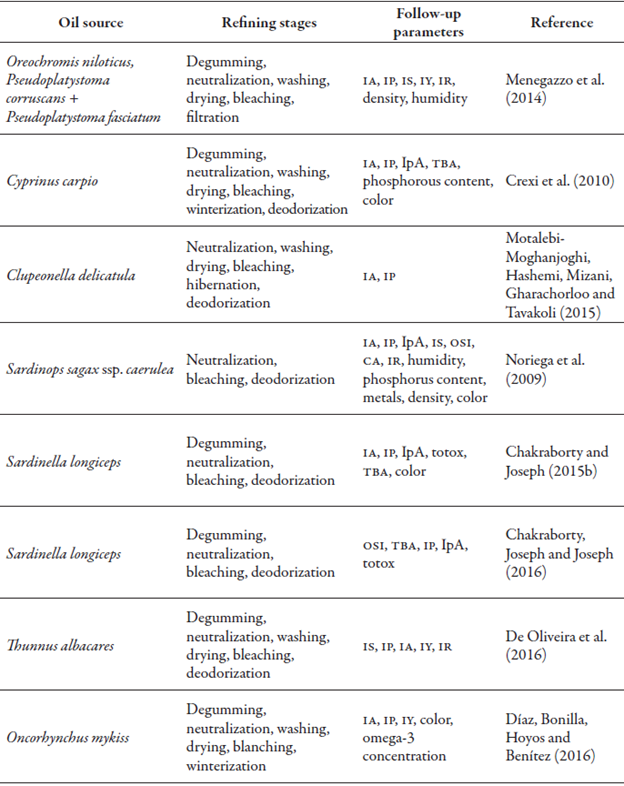
CA: conjugated acids; AI: acidity index; PV: peroxide value; IpA: anisidine index; SI: saponification index; RI: refraction index; IOI: iodine index; OSI: oxidative stability index; TBI: thiobarbituric acid index; Totox: total oxidation value.
Source: Elaborated by the authors
Although the refining process has been studied for several years, many of the investigations are still directed towards oils of vegetable origin, which involves the evaluation of several stages and new techniques that have not yet been reported for fish oil, but that have the potential for its application in this material.
Some authors have carried out a review of different discovered and rediscovered technologies (as in the case of enzymatic processes) for the optimization of the refining process of edible oils (table 7) (Čmolik, &Pokorný, 2000; Kumar & Krishna, 2015; Misra, Nandi, & Nandi, 2013; Moharana, Byreddy, Puri, Barrow, & Rao, 2016; Vaisali et al., 2015).
Table 7 Technologies with application potential in fish oil refining
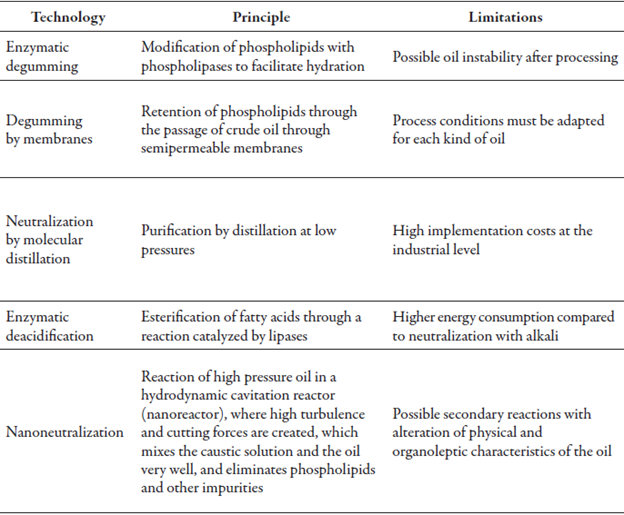
Source: Elaborated by the authors
The real challenge is to achieve a balance between process quality and sustainability, and savings in potential costs and oil yield, i.e. key factors for the implementation of new technologies (De Greyt, 2012).
PUFA concentration in fish oil
Several techniques have been proposed for the concentration of PUFA, and especially for omega-3, including winterization, concentration by enzymatic methods, fractionation by supercritical fluids and by chromatographic methods, formation of com-plexes with urea, and concentration by membranes.
Fish oil winterization
Winterization is a process that involves partial crystallization of the oil by controlled cooling, followed by filtration. Its main objective is to separate saturated fatty acids from unsaturated ones. This separation is possible due to differences in melting points of the fatty acids, which depends mainly on the chain length and the unsaturation degree.
Thus, saturated and monounsaturated fatty acids, which have a higher melting temperature, crystallize and can be separated by filtration, while PUFAs remain in liquid form in the oil (Vázquez & Akoh, 2012).
The use of organic solvents is common in the winterization process in order to increase the rate of mass transfer and fraction crystallization of saturated fatty acids, from oil dissolved in a suitable solvent (López-Martínez, Campra-Madrid, & Guil-Guerrero, 2004; Morales, Muñío, Pérez, Guadix, & Guadix, 2013). This methodology has been one of the main techniques developed for the concentration of omega-3 PUFA from triacylglycerols in natural form (Lei et al., 2016).
Hence, Cunha, Crexi and De Almeida-Pinto (2009) performed the optimization of the fish oil winterization process, evaluating the type of solvent (acetone and hexane), its proportion (40 % and 60 %), and agitation in the second cooling stage (0 and 40 rpm), and obtained a statistical model for the concentration of unsaturated and saturated fatty acids. The best conditions obtained were 40 % hexane without agitation in the second cooling stage, which increased the concentration of unsaturated fatty acids by 9.2 % and reduced the saturated fatty acids by 13.3 % compared to the bleached oil.
Likewise, Tengku‐Rozaina and Birch (2013) carried out winterization of hoki or blue grenadier oil (Macruronus novaezelandiae) with and without hexane as solvent. They observed that the use of this solvent facilitated the separation of the oil fractions and allowed a higher concentration of omega-3 fatty acids.
Meanwhile, Homayooni, Sahari and Barzegar (2014) evaluated three different winterization temperatures (-5, 0 and 10 °C) in sardine oil (Dussumieria acuta) with ethanol as a solvent, and observed that at -5 °C there was a higher decrease in saturated fatty acids and an increase of the unsaturated ones. Results indicated that the concentration of omega -3 PUFA in the non-crystallized portion increased as the temperature decreased.
Nonetheless, Díaz et al. (2016) also evaluated this process in trout oil, by optimizing the percentage of acetone and the crystallization time and achieved an increase in DHA and EPA of 69 % and 51.6 %, respectively.
Fractionation by supercritical fluids (FSCF)
This technique has been proposed for the extraction of oil, as well as for the concentration of PUFA, in particular, omega-3 such as DHA and EPA, from oil extracted with conventional methods, which is also known as fractionation.
Several studies address oil fractionation in its natural state, but it seems to be ineffective, possibly due to the complex structure of fish oil, composed of a large number of triacylglycerols (Corrêa, Peixoto, Gonçalves, & Cabral, 2008; Homayooni et al., 2014).
Lopes et al. (2012) evaluated the fractionation of fish oil from fresh water with a low omega-3 content of approximately 10 %. The best fractionation was obtained in the isotherms of 33 and 40 °C at 20,000 kPa. Ferdosh, Sarker Norulaini, Akanda et al. (2014) also studied this process in tuna oil, using ethanol as a co-solvent, and found that it was highly effective in PUFA recovery.
The structural complexity of fish oil has hindered its fractionation, so the interest in the synthesis and fractionation of methyl esters and ethyl esters of triacylglycerols from fish oil has increased, being more stable compounds than fatty acids. These are obtained by hydrolysis of triacylglycerols (TAG) and alkylation of fatty acids, before the FSCF (Lopes et al., 2012; Rubio et al., 2010).
Perretti et al. (2007) studied the concentration of omega-3 from a commercial mixture of fatty acid esters obtained from fish oil, using FSCF with supercritical fluids-CO2. They studied different pressures (10,000, 14,000, 15,000 and 30,000 kPa) and various CO2 fluxes, maintaining the temperature of the three column sections at 40, 50 and 60 °C, respectively, to increase the concentration of DHA and reduce the EPA: DHA ratio, an important characteristic to define its functional properties.
The increase in pressure and flow rate caused an increase in DHA concentration (from 24.54 % to 49.57 %) and a desired reduction in the EPA: DHA ratio from 1.61 to 0.65, whereas saturated and monounsaturated fatty acids decreased from an average of 3.33 % to 0.6 %.
Létisse and Comeau (2008) applied this type of fractionation in fatty acid ethyl esters of sardine oil and observed the effect of temperature when obtaining a higher concentration ratio of EPA (24.74 %) and DHA (26.02 %) at 60 °C compared to 40 °C (4.28 % and 7.53 %). Likewise, the density of CO2 showed a higher concentration of DHA and EPA, increasing from 700 to 800 kg/m3, reaching a composition close to 40 % and 60 % of EPA and DHA, respectively.
On the other hand, some thermodynamic models and simulations of this process have been carried out based on state equations such as the ones published by Gironi and Maschietti (2006), con-tribution of groups (GC-EOS) (Espinosa, Díaz, & Brignole, 2002) and the methods of McCabe-Thiele and Ponchon-Savarit (Riha & Brunner, 2000).
Likewise, also relationships between top temperature, number of theoretical stages, the ratio solvent: food in countercurrent fractionation with internal reflux (Maschietti & Pedacchia, 2014), and simplified models in the equilibrium stage (Pieck, Crampon, Charton, & Badens, 2016) to design a separation process of fatty acid ethyl esters that maximizes the concentration of omega-3 fatty acids.
Fractionation by chromatography
The chromatographic methods are used for the concentration of PUFA, in particular of EPA and DHA, and include liquid chromatography (HPLC), and argentometric and supercritical fluids, to obtain products of high purity (> 95 %) (Dillon, Aponte, Tarozo, & Huang, 2013). In general, the separations are carried out through argentometric chromatography and are conducted on ethyl esters of fatty acids, in some cases combining preconcentration steps prior to the final fractionation by chromatography (table 8).
Table 8 Fractionation of fish oil by chromatographic methods (since 2000)
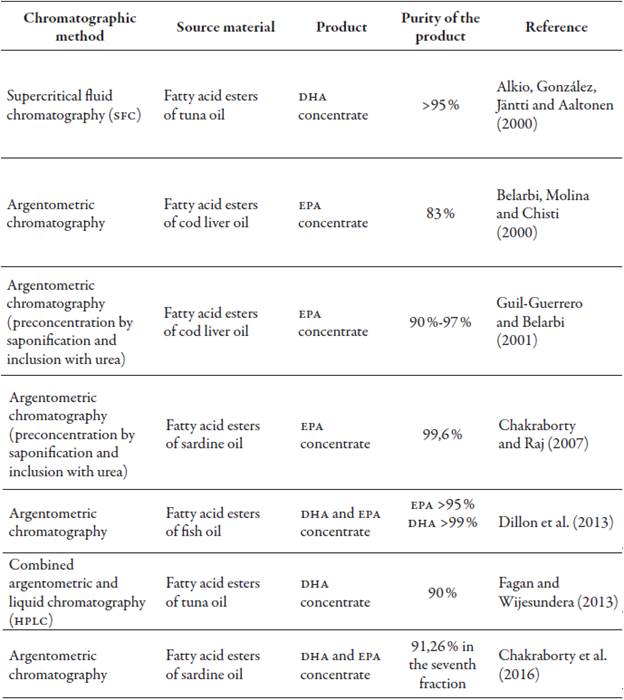
Source: Elaborated by the authors
Liquid chromatography (HPLC) is used for the separation of omega-3 fatty acids from microalgae and fish oil. However, authors have reported that it is easier to separate DHA from oil, from simple cells compared to fish oil, because the latter has a more complex composition. With this technique, the esters of fatty acids are eluted from their number of carbon equivalents. Moreover, the esters with the same number can be separated under certain optimal conditions (Fagan & Wijesundera, 2013).
Argentometric chromatography is frequently used for the concentration of fatty acids, according to the degree of unsaturation, since the ability to form complexes with silver ions increases with this grade. For this reason, highly unsaturated fatty acids such as DHA and EPA are retained more strongly than others with less unsaturation, facilitating their separation.
Different configurations have been designed in the stationary phase, in order to improve the stability of the silver ion (i) and reduce its mobility, such as the use of silver nitrate, silver covalently bound to 3-mercaptopropyl as a stationary phase, and the stationary phase thiolate-silver (Dillon et al., 2013; Fagan & Wijesundera, 2013).
On the other hand, supercritical fluids have the density and solvent capacity of certain liquids, but with lower viscosity and better diffusion, so they can be used as substance carriers such as the mobile phase in gas chromatography or dissolve them as the solvents in HPLC. This technique is known as supercritical fluid chromatography (SCFC) (Taylor, 2009).
SCFC is especially suitable to separate high purity omega-3 PUFA, since it combines the high selectivity of the supercritical fluids and the stationary phase (Rubio et al., 2010). As with other techniques, investigations are conducted mainly on ethyl esters of fatty acids.
In fish oil, Alkio et al. (2000) applied this technique for the concentration of ethyl esters of EPA and DHA, using supercritical CO2 as a mobile phase and a stationary phase of octadecylsilane (ODS). They obtained a production rate of DHA ethyl ester with a 90 % purity of 0.85 g/(kg ODS*h) and EPA ethyl ester with 53 % purity of 0.23 g/(kg ODS*h).
Concentration using enzymatic methods
The concentration by enzymatic methods is based on the selectivity of some lipases for certain fatty acids or positions in the triacylglycerol molecules, catalyzing reactions of hydrolysis, alcoholysis or transesterification of triacylglycerols (Correa, Tejeda, Martín, García, & Noriega, 2017; Miranda, Baeza, Noriega, García, & Otero, 2013; Rubio et al., 2010).
Some studies propose the application of these processes before other stages, such as molecular distillation or filtration by membranes, to obtain concentrates of omega-3 fatty acids. Table 9 shows the materials studied and the type of enzymes used to concentrate fish oil.
Other investigations have studied the process of alcoholysis in the presence of solvents such as supercritical CO2, because it is green, non-toxic and can easily be removed from the reaction products, which makes it a good mean to carry out the enzymatic reactions (Lin, Chen, & Chang, 2006). Besides, it can easily be coupled with other processes such as SCFE, FSCF, SCFC and encapsulation with supercritical CO2 (Rubio et al., 2010).
Furthermore, Lin et al. (2006) studied the concentration of omega-3 PUFA from Sardinella aurita oil using Lipozyme IM-60, obtaining favorable results and finding that when using supercritical CO2 the conversion was 40 % higher than under regular environmental conditions.
However, in more recent investigations (Shin, Sim, Kishimura, & Chun, 2012; Tanbirul-Haque & Chun, 2015), no significant effect was found when using this same solvent in the alcoholysis of fish oil with Lipozyme TL-IM, Lipozyme RM-IM and Novozyme 435, although it seems to improve the thermal stability of the enzyme and reduces the oxidation of omega-3 PUFA, tuna oil (Thunnus sp.) and sardine (S. pilchardus) (Melgosa et al., 2017).
Other PUFA concentration methods
Other methods for the concentration of PUFA include molecular or short-path distillation, filtration by membranes and the formation of complexes with urea.
Molecular distillation is a technology that can be used appropriately for the separation, purification or concentration of thermolabile substances, since it operates with high vacuum pressures (lower than an absolute pressure of 1,000-500 kPa) (Cerón, Cardona, & Toro, 2012; Pramparo, Prizzon, & Martinello, 2005).
Although in some cases this technology is used as an oil purification process for the removal of organic pollutants (Olli, Breivik, & Thorstad, 2013), it has also been applied in the concentration of PUFA, particularly of EPA and DHA in free form or as ethyl esters (Oliveira & Miller, 2014; Solaesa, Sanz, Falkeborg, Beltrán, & Guo, 2016; Wang et al., 2012). Likewise, in tuna oil, Wang et al. (2012) achieved a concentration increase of the total content of EPA and DHA from 32.11 % to 82.23 %, and similarly, Solaesa et al. (2016) reached an increase in the concentration of acylglycerols of omega-3 PUFA from 63 % to 91 % in sardine oil.
Membrane filtration is characterized by the application of hydraulic pressure as a driving force for mass transfer. The nature of the membrane controls which components will permeate, and which will be retained, according to their molar mass or particle size.
This technology has been used in degumming, recovery of solvents in extraction processes, pigment removal, acidity reduction, concentration of minor components, removal of waxes and separation of emulsions (De Morais- Coutinho et al., 2009), just as in PUFA (Ghasemian, Sahari, Barzegar, & Ahmadi, 2016; Ghasemian, Sahari, Barzegar, & Gavlighi, 2015; Linder et al., 2005; Linder, Matouba, Fanni, & Parmentier, 2002).
Thus, in salmon oil, the decrease in saturated fatty acids has been observed from 27.2 % to 20.2 %, while the PUFA content increased from 41.6 % to 46.5 %, with an increase of DHA from 9.9 % to 11.6 %, and EPA from 3.6 % to 5.6 % (Linder et al., 2005).
Nevertheless, Ghasemian et al. (2015) performed an optimization study for the concentration of omega-3 PUFA by polymeric membrane in fish oil, in which they evaluated the effect of temperature, pressure and agitation speed. These authors found that the optimal conditions were 36.19 °C, 4.82 bars and 43.01 r.p.m., respectively, which resulted in a maximum omega-3 PUFA value of 35.11 %.
On the other hand, the formation of complexes with urea is the simplest and most efficient technique to obtain omega-3 PUFA concentrates as free fatty acids or ethyl esters of triacylglycerols. Saturated and monounsaturated fatty acids are separated from polyunsaturated fatty acids starting from a saturated solution of urea, in which all the fatty acids are found.
By cooling and filtration, the compounds formed between the saturated and monounsaturated fatty acids can be removed during crystallization. PUFAs do not form inclusion complexes with urea, so they remain concentrated in the liquid fraction (Homayooni et al., 2014).
Studies on this technique mainly evaluate the relationship between urea and fatty acid, tempera-ture and crystallization time (Gámez et al., 2003; Homayooni et al., 2014; Liu, Zhang, Hong, & Ji, 2006; Suriani, Lawalata, & Komansilan, 2014; Tengku‐Rozaina & Birch, 2013).
With this method, DHA and EPA contents higher than 85.02 % in tuna oil were obtained (Liu et al., 2006), with a ratio of urea: fatty acid of 15, a temperature of -5 °C and in a time period of 20 hours. In sardine oil, the highest amounts of DHA and EPA were found at -10 °C and 1 °C, respectively, where the DHA was enriched from 17.45 % to 29.61 % and the EPA from 15.39 % to 19.76 % (Homayooni et al., 2014).
Conclusions
The omega-3 polyunsaturated fatty acids (PUFA) are highly valued at present for their beneficial effects on health. Fish oil is recognized as the primary source of these fatty acids, mainly eicosapentaenoic (EPA) and docosahexaenoic (DHA) acids.
Some studies employ the extraction of fish oil by traditional methods, such as wet pressing and conventional solvents, but recent research is opting for the application of technologies such as super-critical fluids and fish silage. Refining methods are still based on traditional stages, although there is an important potential for the application of new technologies such as enzymatic and physical refining.
Likewise, for the concentration of PUFA different methods have been proposed, such as enzymatic and chromatographic methods, winterization, supercritical fluids, complex formation with urea and filtration by membranes. However, currently the combination of different techniques seems to offer a good alternative to increase the purity and performance of these components.