Serviços Personalizados
Journal
Artigo
Indicadores
-
Citado por SciELO
-
Acessos
Links relacionados
-
Citado por Google
-
Similares em SciELO
-
Similares em Google
Compartilhar
Ciencia y Tecnología Agropecuaria
versão impressa ISSN 0122-8706versão On-line ISSN 2500-5308
Cienc. Tecnol. Agropecuaria vol.21 no.1 Mosquera jan./abr. 2020 Epub 30-Dez-2019
https://doi.org/10.21930/rcta.vol21_num1_art:1342
Gestión y sostenibilidad ambiental
Techniques applied in agricultural research to quantify nitrogen fixation: a systematic review
1Estudiante, Doctorado Ciencias Agrarias y Agroindustriales, Universidad del Cauca, Investigador Grupo Gibna. Popayán, Colombia.
2Docente, Universidad del Cauca, Investigador Grupo Nutrición Agropecuaria. Popayán, Colombia.
3Docente, Universidad Pedagógica y Tecnológica de Colombia, Facultad Ciencias Agropecuaria. Tunja, Boyacá.
Nitrogen economy is a subject that is growing in popularity at the global level, especially in relation to the agricultural activity, due to nutrient leaching caused by fertilization, as it contributes to the eutrophication of water. As a result, the population of algae increases, and thus, oxygen availability is reduced by accelerating the denitrification process in which N2O is produced, known mostly for its effect on global warming. Likewise, part of the fertilizer is emitted into the atmosphere by volatilization. This has led to the creation of techniques that allow quantifying the nitrogen used by plants and the one that is fixated in the soil through micro-organisms to make more efficient the use of nitrogen in agricultural systems. However, the most significant limitations are their sensitivity, specificity, cost, and the technology that is required to apply them. This has also led to the innovation of procedures and the creation of techniques that have a very low error rate. The aim of this work was to describe the main techniques used to quantify nitrogen fixation with emphasis on the background, procedures, mathematical expressions that are used and future scenarios. The information is described from the analysis of available trials included in the Scopus database. This work consolidates the techniques that continue to be used to quantify nitrogen and facilitates their use over time with prediction models, as well as their importance, advantages, and disadvantages.
Keywords eutrophication; fertilizer application; forecasting; isotopes; nitrogen fixation
La economía del nitrógeno es un tema que viene creciendo a nivel mundial, especialmente en la actividad agrícola cuando por mal manejo de la fertilización ocurren pérdidas por lixiviación que contribuyen a la eutrofización del agua, lo que incrementa la población de algas y, como efecto, reduce la disponibilidad de oxígeno, acelerando el proceso de desnitrificación en el que se produce N2O, conocido por su efecto en el calentamiento global. Igualmente, parte del fertilizante es emitido a la atmósfera por volatización. Esto ha impulsado la creación de técnicas que permiten cuantificar el nitrógeno utilizado por las plantas y el fijado en el suelo a través de microrganismos para hacer más eficiente el empleo del nitrógeno en los sistemas agrícolas. Sin embargo, dentro de las mayores limitantes se encuentra su sensibilidad, especificidad, costo y tecnología que se requiere para aplicarlas. Esto ha conducido a la innovación de procedimientos y a la creación de técnicas que tienen una tasa de error muy bajo. El objetivo de este trabajo fue realizar la descripción de las principales técnicas utilizadas para cuantificar la fijación del nitrógeno con énfasis en los antecedentes, procedimientos, expresiones matemáticas que se usan, y escenarios futuros. La información se describe a partir del análisis de ensayos disponibles en la base de datos Scopus. Este trabajo consolida las técnicas que continúan vigentes para cuantificar el nitrógeno y facilita entender su uso a través del tiempo con modelos de predicción, así como su importancia, ventajas y desventajas.
Palabras clave aplicación de abonos; eutrofización; fijación del nitrógeno; isótopos; técnicas de predicción
Introduction
The nitrogen molecules that are important in plant nutrition are nitrate and ammonium, whose availability is related to the application of chemical and organic fertilizers, as well as to the transformation of nitrogen in the soil due to physical, chemical, and biological effects. The fixation of this element (N) is performed by a group of symbiotic bacteria (such as root nodule-forming rhizobia) and non-symbiotic (free-living) diazotrophs (Angel et al., 2018). However, it is possible that the efficiency in its fixation is lost due to environmental factors, management practices, integrity and number of plant nodules, and by the type of microorganisms associated with the root (Chalk & Craswell, 2018). Therefore, the need to analyze the dynamics of this element from its fixation, for the design and implementation of strategies that allow taking advantage of the biological process and reduce the application of fertilizers without sacrificing the productive yield of the crops arises. However, there are several limitations to quantify nitrogen fixation, e.g., the degree of complexity of the process, costs and sensitivity of the techniques, high-tech equipment, skilled labor, and reagent availability, among others.
Among the methods used, the latest generation techniques such as nifH gene identification and 16S rRNA (Rampadarath, Bandhoa, Puchooa, Jeewon, & Bal, 2017) stand out; furthermore, dilution methods of nitrogen isotopes and the use of leaf/ canopy reflectance spectra to identify 15N are also found. Moreover, less precise techniques include difference of total nitrogen, N balance in the system, difference of total N in plants, nodular mass, supply of carbohydrates to the root, ureide content, reduction of acetylene, modifications of the Kjeldahl method (Yan et al., 2017), leghemoglobin content in nodules and the GlnLux assay using sensors that detect glutamine (Thilakarathna & Raizada, 2018). However, in the field, a practical way to relate the efficient absorption of nitrogen is with dry matter production (Mondal, Datta, & Mondal, 2017), number of leaves/stems (Bilal, Ayub, Tariq, Tahir, & Nadeem, 2017), and the production of green biomass (Bionda, Babini, Martino, Salas, & Lajmanovich, 2018; Dutta, Datta, & Mandal, 2017).
The interest in the analysis of the nitrogen cycle and the agents involved in its emission and fixation by using laboratory techniques, is related to the high cost of urea and fertilizers used in the field for planting, and the loss of nitrogen molecules both by leaching as well as by volatilization, which contribute to atmospheric and water pollution. This has prompted the study of more efficient procedures for the analysis of nitrogen inflows and outflows in agricultural systems, although they have also been used in other economic sectors, mainly in the industry. The aim of this work was to elaborate a review of the main techniques used to quantify nitrogen fixation with emphasis on the background, procedures, mathematical expressions used, and future scenarios.
Materials and methods
Search in databases
A systematic search of articles, books, series of books and conferences published in the Scopus database was carried at the global level, using the search path TITLE-ABS-KEY (techniques fixation of nitrogen and bacteria) that includes documents in which the descriptors of interest separated by the Boolean connectors "of " and "and" appear in the title, abstract and keywords.
Data processing and analysis
The number of publications was organized in Microsoft Office Excel® per year, and the main countries and areas in which the techniques had been used from 1967 to 2018 were analyzed. The evaluation of the worldwide publication trend per year was carried out with the software SigmaPlot12®, by predicting non-linear regression curves, in which the independent variable corresponded to time, and the dependent variable was the number of publications. The models used were the following: sigmoidal 3-4 parameters, logistics 4, Gompertz 3, and hill 3-4 parameters with a p-value < 0.05. Data independence was corroborated with the Durbin Watson statistic and normality with the Shapiro- Wilk test. Only articles published in the period between 2016 and 2018 were considered to establish the techniques that remain in force and are a trend worldwide, based on the methodology of systematic reviews (Pai et al., 2004). In this methodology, the inclusion criteria of the articles were the following: published articles not older than three years in an indexed journal with the description and generic name of the technique; the exclusion criteria were non-indexed literature and general methods used. In total, 50 articles were analyzed.
Results and discussion
There are 1,016 documents published from 1967 to 2018 in the Scopus database, including articles (967), books (22), series of books (22), and conferences (5). Nitrogen fixation techniques have been applied since 1967 with a regular trend, and their maximum use was in the year 2017. The relevance of these lies in their usefulness in analyzing nitrogen use efficiency employing different emitters and collectors in the ecosystem, as a strategy to make production and business systems more efficient from an environmental point of view. Therefore, these are part of a technology that grows adjusting to a sigmoidal model and whose decline is estimated towards the year 2053 (R2 = 0.99; p < 0.01) for being the turning point with the highest adjustment (R2), and whose Durbin Watson value was closer to 2; however, this may occur before or after that date, due to the small difference between the R2 values, since there is a complexity in the evolution dynamics of any technology as a result of the rapid maturity or development of innovations (table 1).
Table 1 Nonlinear regression models for the equation “techniques fixation of nitrogen and bacteria” used since 1967
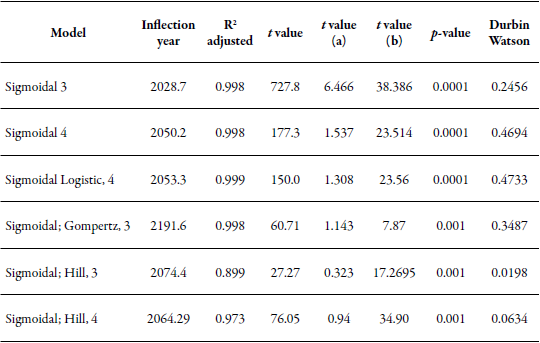
Note: the t statistic and the a and b values belong to the different logistic models.
Elaborated by the authors
This variation in the point of decline that tends to extend indicates that these techniques are in an innovation phase. This phase is characterized by a curve in which most of the data are grouped at the beginning of the model according to the interpretation given to the S curve as a tool for the analysis of the life cycle of a product. For that reason, the efforts of companies must be aimed at strengthening their techniques, making massive investments to conserve the market, and maintain their position vis-à-vis new competitors (Aguilar et al., 2012) (figure 1). The techniques used to quantify nitrogen fixation have not yet reached an equilibrium point over time, and the dissemination of their procedures is carried out from developed countries that hold their property. However, the techniques most applied in less developed countries correspond to the most economical and simple ones due to the lower availability of resources.
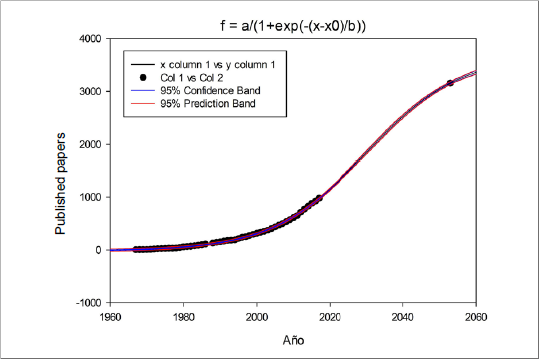
Elaborated by the authors
Figure 1. Publication prediction curve elaborated with the Sigmaplot program (SigmoidalLogistic4).
The prediction model in S-curves shows rapid growth, i.e., the techniques used to quantify nitrogen fixation are in full development, and new competitors may emerge with developments in the most economical and easily adopted processes (Aguilar et al., 2012). This occurs because the most significant limitation in its application is the high cost and low direct adaptability in the field. Thus, the countries that most frequently use the latest generation techniques are developed countries, while in less developed countries, such as those located in Latin America, the oldest techniques continue to be implemented because their application is limited by technology (table 2); for example, Colombia has a participation of only 0.68 % at the global level.
Table 2. Countries highlighted by publishing the most significant amount of research in which nitrogen fixation quantification techniques have been applied
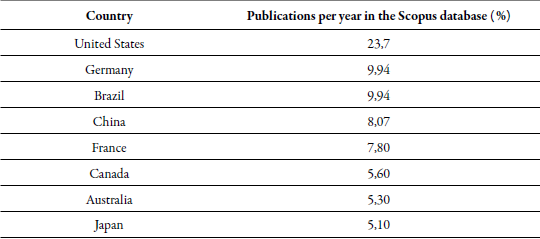
Elaborated by the authors
However, its use is not limited only to the area of agriculture and biological sciences (32.02 %); these techniques have also been used in immunology and microbiology (24.60 %), chemistry and genetics (19.20 %), environmental sciences (9.79 %), medicine (5.01 %), and earth sciences (2.02 %), among others (7.26 %). This demonstrates the growing interest in nitrogen economy and in establishing the most efficient agents in its fixation to replace the use of chemical products and innovate with non-destructive techniques. It is estimated that from the year 2029 (predicted by the Sigmoidal 3 model), which is the time closest to the period of analysis of the publications (1967-2018), a maturity process in its development can be achieved by the amount of advances and products in the market according to the life cycle of the technologies that are summarized in the initial dissemination, early growth, late growth and public knowledge stages (Aguilar et al., 2012).
Techniques used to estimate nitrogen fixation
The oldest methods to verify nitrogen use efficiency in crops are related to the estimation of dry matter production and nitrogen fixation in different parts of the plant. Over time, studies have focused on the specific analysis of the agents involved in the nitrogen cycle, particularly in soil (different depth layers), plants (Rhizobium analysis, nodules, ureide content, and leghemoglobin measurement) (Sainju, 2017), and microorganisms, because the fixation process is directly related to the biological action (free-living diazotrophs and symbiosis). The main techniques are summarized below.
15N isotopic dilution technique
It is based on the detection of isotopes in the sources of nitrogen available for plant growth (N-soil, N-fertilizer, and nitrogen), based on the difference between naturally occurring isotopes (15N) or that have been added or impoverished in the soil or the substrate. This allows identifying in the plant, the nitrogen coming from the different sources (marked and not marked with the 15N tracer) (Braun et al., 2018).
The 15N isotopic dilution technique was reported by McAuliffe to estimate the proportion of nitrogen fixed in legumes by biological action. This is carried out by comparison with a reference plant that does not fix nitrogen, from the difference in the concentration of isotopic atoms when they grow in a soil that has been enriched with 15N isotopes (Chalk & Craswell, 2018). These are added in solutions of 1 to 10 % excess to the soil or the fertilizer (kg N/ha) (15NH4 and 15NO3), prior to planting in the same amount for the plant that fixes nitrogen and the one that does not fix nitrogen (Braun et al., 2018; Wambeke et al., 2018). So, in the classical method, the following equation is used to calculate the atmospheric nitrogen that has been fixed:
Where E represents the percentage of 15N isotopic atoms that have been added in excess (Chalk & Craswell, 2018). This technique is expensive as mass spectrometers have to be used to obtain the gases and the identification of the isomers. For this, it is common to use coupled systems consisting of a spectrophotometer connected to a combustion system (oven) (Hamawaki & Kantartzi, 2018). However, as an alternative, the use of leaf/canopy reflectance spectra has been chosen to identify the 15N isomer (Thilakarathna, Moroz, & Raizada, 2017). The method is summarized in the application of solutions with 1 to 10 % excess of 15N atoms to the soil (Braun et al., 2018; Wambeke et al., 2018) or in the fertilizer (kg N/ha) before planting. Further, also in the ratio of isotopes in the plants, in the soil, in microorganisms, and in the air, is estimated. In plants, 15N is quantified in samples that have been dried, e.g., soybean roots, nodules, and seeds; these have been placed at 70 °C for 72 hours (Hamawaki & Kantartzi, 2018). 15N isotope detection is possible due to its higher weight (atomic mass of 15) compared to the nitrogen atoms that are in the atmosphere (atomic mass of 14), which allows them to separate during the process and, subsequently, be collected and quantified in the spectrophotometer. The following equation can be used to estimate the percentage of isotopes, which consider the contribution of isotopes in the air (0.3663 % of 15N atoms) (Hamawaki & Kantartzi, 2018):
On the other hand, for the evaluation of the microbial mass, isolations have been made, and mathematical models have been established (Hamawaki & Kantartzi, 2018). It is also possible to carry out an in situ incubation for roots and soils in parafilm, from the injection of gas enriched with 15N. Amounts of 4.5 to 20 mL of 15N gas have been reported regarding air in the environment (Ritchie & Raina, 2016), and at the end of the incubation, the samples are dried, grounded, and weighed, and then, their 15N concentration is measured. However, it is possible to overestimate fixation if potential changes in nitrogen concentration are not considered as a result of denitrification, and if the commercial form of isotopes used is contaminated with other gases that can be absorbed by plants and microorganisms (Roley et al., 2018). Although in a study, in which a 15N-NH4 + marker with an amount of 10 % atoms in the atmosphere was used to corroborate this hypothesis, no relationship was found. On the other hand, studies showed that it must not be ruled out that the amount applied was mild (Braun et., 2018).
Some disadvantages of the technique are the following:
1. Leaching of the 15N tracer in the enriched solutions that are applied to the soil, so their daily reinstatement is needed, increasing labor costs.
2. In closed systems, leaks may occur.
3. The homogeneous distribution of the enriched material (15N) on the ground is difficult.
4. The asynchrony in the capture of mineral nitrogen by the legume and the reference plant, contribute to the achievement of differences in results (Chalk & Craswell, 2018).
5. The cost of media enriched with 15N isotopes and equipment is high.
6. Consumption of the 15NN-NH4 tracer in soil samples due to the effect of fixation on clay minerals and humic substances, or by microbial absorption, may alter the results (Braun et al., 2018).
7. Variation in the content of 15N in the plant during different growth stages (Hamawaki & Kantartzi, 2018), and easy contamination of artificial media such as hydroponic solutions and its support material (sand, perlite or vermiculite or mixtures thereof, e.g., 70 % peat, 20 % perlite and 10 % vermiculite) (Hamawaki & Kantartzi, 2018).
However, this is the recommended method to analyze nitrogen dynamics in the soil, so that according to the study focus, the target product is marked in the analysis. In the case of mineralization, it is NH4 with an enriched tracer (15N–NH4) (Braun et al., 2018).
Natural abundance technique (δ 15N)
This technique is based on the quantification of naturally available 15N in plants and soil derived from atmospheric fixation (De Carvalho et al., 2017), because the 15N and organic nitrogen fractioning are observed during nitrification (Chalk & Craswell, 2018); that is, due to low nitrate absorption there must be a higher concentration of isotopes in the soil. For this, the difference in the percentage of 15N atoms between the material evaluated and the natural abundance in the atmosphere is established (0.3663 % of 15N atoms) (Fuertes-Mendizábal et al., 2018). The following equation is used to estimate the proportion of nitrogen fixed by a legume derived from the atmosphere (Patm) (Chalk & Craswell, 2018):
Where B represents the abundance of isotopes (15N) in the legume, dependent solely on the nitrogen fixation process; 15N ref is the abundance of the 15N isotope in the reference plant, and 15N leg is the abundance of the 15N isotope in the legume (Van Vugt, Franke, & Giller, 2018). Shoots are the samples commonly used; these are dried at 65 °C for 72 hours or until a constant weight is reached. After drying, the 15N is weighed, grounded and quantified in a mass spectrophotometer (De Carvalho et al., 2017); furthermore, the use of grain tissue (2.5 mg) has also been validated, so that the fixed nitrogen efficiency can be estimated as kilogram of grain produced per kilogram of nitrogen absorption from growing shoots (Polania, Poschenrieder, Rao, & Beebe, 2016). Some nitrogen-fixing plants have a reference value (B) as follows: for soybean grains, a value of -2 ‰ has been reported (Van Vugt et al., 2018); further, -3.09 ‰ for soybean in sprout, and -2.44 ‰ in grain for genotypes with growth stage habit II (Polania et al., 2016); however, a value of -0.80 has also been observed (Van Vugt et al., 2018). In grasses, a value of 0 (zero) is usually used (De Carvalho et al., 2017). Differences observed in the same species occur because, within the plant, there is discrimination in the distribution of 15N in its organs (Polania et al., 2016). The natural abundance method only works if the 15N of the legume is between the ‘B’ value and the 15N of the reference plant (Van Vugt et al., 2018). Besides, it is possible to calculate the amount of nitrogen accumulated by plants by multiplying the total nitrogen by its biomass, respectively (De Carvalho et al., 2017).
On the other hand, it is also possible to establish the amount of nitrogen fixed by biological action —for which hydroponic means, sand or other sterile support that does not contain nitrogen are used— in controlled tests to establish the abundance of 15N isotopes, which are fixed and do not have a different origin. Open field trials have also been carried out in unmarked plots and marked with the 15N tracer, to establish the concentration of isotopes in plants concerning their availability in the air (Van Vugt et al., 2018). The 15N fixed by microorganisms varies in legumes (Chalk & Craswell, 2018), due to the different species of Rhizobium and the support used that can contribute to the degree of error, by the presence of molecules that contribute with ammonium ions. In general, the technique has several drawbacks:
1. A reference plant must be used to establish the availability of the 15N isotope derived from the soil to make adequate estimates.
2. It is necessary to determine the B value, since it differs by species and by the type of strain associated with the legume. Hence, assuming reference values does not guarantee the accuracy of the results.
3. The type of plant and the sample used are also factors that influence this technique (Chalk & Craswell, 2018)
4. The abundance of the 15N isotope in the soil is variable, since factors such as rain, influence its concentration because it is a reincorporation mechanism of this element to the ground (Polania et al., 2016).
Therefore, when applied, it is ideal to use other techniques such as isotopic dilution and variations such as tracking the 15N isotope in microbial RNA using SIP-Raman microspectroscopy, from samples incubated for three days with diazotrophic bacteria and 15N. Subsequently, the identification of the microorganisms that fix nitrogen in their biomass is done in a highly sensitive way in diverse environments. Although this technique does not have the same sensitivity in all microbial strains that fix nitrogen in symbiosis and as free-living organisms (Angel et al., 2018), it has the advantage of having high precision to estimate isotopes in the material evaluated by the sensitivity of the equipment. Furthermore, since the application of isotope-labeled fertilizers is not necessary, it is relatively cheaper than the isotopic dilution technique.
ARA method (acetylene reduction assay)
From the discovery of Schoöllhorn, Burris, and Dilworth in 1966 (Dilworth, 1966; Schoöllhorn & Burris, 1967) that nitrogenase reduces acetylene (C2H2) to ethylene (C2H4), the authors suggested that the acetylene reduction rate can be used as a nitrogen fixation rate index (Stewart, Fitzgerald, Burris, & Nov, 1967). Since then, it is used comprehensively; in this technique, a gas chromatograph is required and 10-20 % air volume (Chauhan et al., 2017) is replaced by acetylene in an incubation chamber that is left at 28-30 °C for 2 to 5 days (Chauhan et al., 2017; Das & De, 2018; Yong et al., 2018). Likewise, entire plants, soil, nodules, and bacteria samples can be analyzed (Camelo- Rusinque, Moreno-Galván, Romero-Perdomo, & Bonilla-Buitrago, 2017). When nodules are used, nitrogen fixation is calculated using the following equation (mL/h/plant) (Yong et al., 2018):
When the sample corresponds to bacteria, the strains are cultured in semisolid media (Nfb) in vials (García et al., 2017) for 5 days at 28 °C and are exposed to atmospheres with 10 % acetylene for 2 to 5 days; subsequently, a sample of 1 mL is extracted and analyzed by gas chromatography (Chauhan et al., 2017). One of the major limitations in this technique is related to the integrity of the plant nodules, so the nitrogenase activity is not altered (Chalk & Craswell, 2018). Even acetylene is considered toxic for some microorganisms (Angel et al., 2018), and a large amount of plant material and soil are required to increase the reliability of the analysis. However, the following aspects stand out among the advantages offered by this technique. 1) it is cheaper than other methods such as isotopic dilution and natural abundance; 2) a gas chromatograph is a less complex equipment than a mass spectrophotometer, and 3) faster results can be obtained with reductions in acetylene to ethylene being reported after five seconds to 30 minutes (Stewart et al., 1967). Nevertheless, the following disadvantages are found: 1) it is less sensitive, and it is not recommended for samples where diazotrophic activity is low or sporadic, and 2) gas leakage may also occur during incubation (Angel et al., 2018).
Microbial bioassay
This technique was developed as an alternative to the acetylene reduction technique by Das and De (2018), who applied a direct approach to the quantification of fixed nitrogen from the culture of diazotrophic bacteria in Jensen medium (composition: sucrose 20 g/L, dipotassium phosphate 1 g/L, magnesium sulfate 0.5 g/L, sodium chloride 0.5 g/L, ferrous sulfate 0.1 g/L, sodium molybdate 0.005 g/L, and calcium carbonate 2 g/L, agar 15 g/L).
For this technique, soil samples of the first 5 cm in the area to be analyzed are taken and transported under refrigeration conditions; then, these are screened and a part is dried in an oven until obtaining a constant weight, and 10 g of a representative sample is washed with isotonic phosphate buffer solution and filtered. From this suspension, a serial dilution of up to 10-4 is obtained, and 1 mL is inoculated in Jensen medium and incubated for two weeks at 30 °C. This procedure is repeated 10 times to ensure the attainment of pure colonies; subsequently, the amount of nitrogen in the microbial cells is calculated, employing the hightemperature catalytic oxidation method (HTCO), and the nitrogen fixation rate is estimated with the following equation:
Where the total bacterial cells are calculated from the multiplication between the number of colonies and the amount of bacteria found (Das & De, 2018). The authors indicate that there is a positive correlation between this method and the ARA test, but they also observed that being a direct technique, the quantification of fixed nitrogen is higher compared to ARA, although further trials are required to corroborate its sensitivity. Other advantages of this technique are the following: 1) lower cost, since no complex equipment is used, and 2) it serves to analyze any soil (Das & De, 2018). However, the most significant limitation is that it is time-consuming, since the results are obtained after approximately 140 days, and contamination problems may occur. Besides, it is possible that not all of the nitrogenfixing microbial mass can be recovered in this medium (Das & De, 2018).
Ureide content
In ureide exporting legumes, the nitrogen fixed by the rhizobia within the root nodules is assimilated into the glutamine amino acid (Gln) and is metabolized into the ureides, i.e., allantoin and allantoic acid, whose import into the xylem is made from ureide permease (UPS1) (Carter & Tegeder, 2016; Santos et al., 2018). Then, these are distributed in the plant, but they accumulate in higher proportion in stems (Carter & Tegeder, 2016; Thilakarathna & Raizada, 2018), especially in the vegetative state of legumes, although during the reproductive state, they are transferred from the stems to pods and seeds (Yong et al., 2018); therefore, the development state of the plant must be considered when sampling.
This technique is an indirect way to evaluate biological nitrogen fixation, because a reduction in ureide content indicates less fixation of nitrogen compounds (Santos et al., 2018); therefore, as this technique does not offer exact nitrogen fixation results, it has been disused in the short and mediumterm (Thilakarathna & Raizada, 2018). The samples generally used correspond to leaves, stems, pods, and grains. A sample of 0.4 g of the material to be evaluated is weighed and mixed with phosphate buffer (80 °C) for five minutes; subsequently, it is centrifuged (5,000 rpm) twice as long, and the supernatant is collected and stored (-20 °C). Then, 1.5 mL of this solution is extracted and exposed to NaOH (0.5 mL), HCl (0.5 mL) and phenylhydrazine hydrochloride (0.5 mL) (Tai-wen et al., 2018) to break the tissues and then measure the amount of ureides in a spectrophotometer (Kataria & Baghel, 2016). This method has the following advantages: 1) it is cheaper compared to techniques such as isotopic dilution, natural abundance, and reduction of acetylene, and 2) it is relatively fast, although a high number of samples are required to increase the reliability of the results.
GlnLux trial
Glutamine (Gln) is a primary transport form of nitrogen once it is absorbed by the root (Thilakarathna et al., 2017). In the GlnLux assay, glutamine is detected by biosensors that are entire cells that emit light when they come in contact with this amino acid. These cells correspond to strains of Escherichia coli that are auxotrophs for Gln and express a constitutive lux operon (Thilakarathna et al., 2017); therefore, nitrogen fixation is associated with the cost of light that can be measured using a luminometer. In general, the test is based on measuring Gln output using a GlnLux biosensor (Thilakarathna et al., 2017). Its sensitivity has been verified to be comparable with studies carried out with high-performance liquid chromatography (HPLC) according to a study in which corn (Zea mays L.) leaves were analyzed.
The procedure consists in taking samples from the first to the third leaf in order of emergence with a manual perforation disc for leaf tissue (6.35 mm in diameter) and immediately storing them in liquid nitrogen; then, these are homogenized by adding sterile sand and 20 μL of protease (0.1 %), and then centrifuging. The supernatant is diluted 100 times and refrigerated (-20 ° C); subsequently, 10 μL of plant extract is mixed with 10 μL of GlnLux cells and 80 μL of M9 medium, and incubated for two hours for biosensor activation; afterward, the Gln output is quantified with the luminometer (Thilakarathna et al., 2017); however, the mentioned proportions may change.
The biggest limitation of this method is that it only reports the relative amount of Gln and not the absolute amount (Thilakarathna et al., 2017). However, it has the following advantages. 1) it is a fast technique in which high quantity of samples (young leaves) are not required; 2) the GlnLux biosensor has high sensitivity: 3) it allows the processing of several samples, and 4) it facilitates the analysis of nitrogen absorption, assimilation and redistribution dynamics in the plant from the detection of Gln (Thilakarathna et al., 2017). PCR techniques to identify nifH genes fH PCR techniques have been used to identify diazotrophic bacteria that are isolated from roots (Castanheira et al., 2017), soils, leaves, and stems. In general, between 0.25 g and 0.5 g of sample are taken for testing, and the DNA extraction protocol is performed. In these, solvents are used to lyse the cell wall to liberate the DNA that precipitates and is preserved in vials under refrigeration conditions. Subsequently, the DNA quality is corroborated by agarose gel electrophoresis, and DNA amplification is continued by PCR in a thermal cycler with primers specific for the nifH gene (e.g., ATGCCTGCAGGTCGACTCTAG (direct primer) and CCTTGTACTTGGTCGTGTTTGAC) (Ritchie & Raina, 2016; Roley et al., 2018; Tchuisseu, Berger, Patz, Fankem, & Ruppel, 2018). The nifH genes are diversified, and their transcription is controlled by RNA polymerase, which is an alternative holoenzyme that also needs a nifA activating protein (Satyanarayana, Krishna, Pavan, & Jeereddy, 2018). Other genes identified by PCR are 16S rRNA (Akemi et al., 2017; Jaiswal, Msimbira, & Dakora, 2017; Tahon & Willems, 2017), because it allows a more detailed phylogenetic classification at the species level (Osei, Abaidoo, Ahiabor, & Boddey, 2018; Ribeiro et al., 2018), and rec A, atpD, gln II and nod C genes with multilocus sequence analysis (MLSA) (Leite et al., 2017; Mwenda, Hara, De Meyer, Howieson, & Terpolilli, 2018; Rigonato et al., 2017). With this technique, it has been possible to identify the nifH gene in genera such as Paenibacillus, Enterobacter, Klebsiella and Pantoea (Ndungu et al., 2018), that are not recognized for fixing nitrogen. Therefore, it is possible that they have obtained the capacity to fix nitrogen through lateral gene transfer. However, the evolutionary lateral gene transfer forces are not well known (Khalifa & AlMalki, 2018).
Although it is a specific technique, it is necessary that when it is used, the morphological and biochemical characterization of the microorganisms must be carried out (Liu et al., 2017). It has the following advantages: 1) it allows the extended characterization of microorganisms from the identification of the nifH gene; 2) it is a rapid test and DNA extraction kits can be used to speed-up the procedure, and 3) it is highly sensitive in the detection of nifH gel (Leite et al., 2017). However, some limitations of the technique are the following. 1) high cost to carry out the procedure; 2) the quality of the primer template establishes the sensitivity and reliable amplification of the DNA, so the state of the genetic material has to be corroborated by electrophoresis, and 3) it does not admit any degree of contamination both in the procedure as well as in the reagents (Tahon & Willems, 2017).
Nodular mass
Nodular mass is an indirect method in which the number of nodules, their mass (Arshi, 2017), distribution in the root system (Thilakarathna & Raizada, 2018) and structural characteristics (Akemi et al., 2017), are related to the ability of the plant to fix nitrogen, since the nodules develop as a result of the symbiotic association between plant and bacteria. Therefore, high nitrogenase activity is associated with a good nodular state. In this technique, samples of entire plants or only the root system are collected in airtight bags and transported under refrigeration conditions (4 °C). Subsequently, a physical analysis of the nodule structure is carried out, which includes color, integrity, size and total number per plant; then, the nodules are separated from the root, washed with distilled water, disinfected with hydrogen peroxide (30 %) for three minutes and washed again with sterilized water. Finally, these are dried at 70 °C until they reach a constant weight, and the final weight is registered (Argaw & Mnalku, 2017; Yong et al., 2018). Nevertheless, it should be noted that the number and mass of the nodules changes according to the age of the legumes and factors, such as drought (Hashem et al., 2018), soil management and salinity, which in turn, influence symbiosis functions (De Andrade Albenísio, Bonifacio, Cerqueira, & Barreto, 2018), and modify the nodular development. Thus, the technique tends to be inaccurate as samples are required in all plant states and kept under the same conditions to reduce the sampling error. This technique has the following advantages: 1) it is an economical method that does not require complex equipment; 2) highly qualified personnel is not required; 3) rapid results are obtained, and 4) it is an indirect way to estimate the effective symbiosis of Rhizobium bacteria that are inoculated in the cultures (De Andrade et al., 2018).
Leghemoglobin measurement
The biological nitrogen fixation process is catalyzed by the nitrogenase enzyme complex that is highly susceptible to the presence of oxygen. Therefore, a protective mechanism to prevent its inactivation is the expression of the leghemoglobin protein that is inside the nodules and captures the oxygen that enters the cell. Thus, this hemoprotein (Santos et al., 2018) prevents oxidation of nitrogenase and guarantees efficient nitrogen fixation (Kataria & Baghel, 2016). The procedure consists of taking between 1.25 g and 2 g of fresh root nodules, which are washed and mixed homogeneously with five volumes of phosphate buffer solution (6.8 mol/L; 5 °C; pH 6.8). The samples are centrifuged at 100 rpm for 15 min at 15 °C; subsequently, the sediment is discarded, and the sample is centrifuged again at 39,000 rpm for 20 min at 15 °C, and the red sediment is measured with a spectrophotometer (Yong et al., 2018). It should be noted that there is a close relationship between the content of SeO in the soil and the concentration of leghemoglobin in the nodules. This was verified in a trial in which 0.1 and 1 mg/kg of soil was added as Na2SeO4 for the cultivation of soybean. This compound acts as an antioxidant that reduces lipid peroxidation, so that supplied in low concentrations, stimulates growth, photosynthesis, and production of leghemoglobin in nodules (Kataria & Baghel, 2016).
Some advantages of this technique are the following: 1) it generates rapid results that allow the nitrogenase activity to be analyzed indirectly, and 2) it has a lower cost since the only equipment required is a spectrophotometer (Yong et al., 2018). However, it is not accurate, and lacerations in the nodules can affect the results. Therefore, sampling should be performed with caution, and the time between collection and processing should not take too long (Kataria & Baghel, 2016).
Techniques used in different production systems
Crops in which more studies have been carried out to identify nitrogen fixating microorganisms that make symbiosis, those that are free-living and others that are endophytes, correspond mostly to species that are economically important as food and livestock resources (grasses and legumes) as well as for forestry use (e.g., in eucalyptus, the identification of endophytes with the nifH gene) (Fonseca et al., 2017) (table 3). In these systems, the application of synthetic fertilizers is usually high to guarantee the production that is demanded worldwide. This favors the tendency to carry out studies focused on the efficient use of nitrogen due to the high cost of fertilizers, and because their indiscriminate application can alter the biological quality of the crops and the yield, as well as contaminate the water table (Bilal et al., 2017; Yong et al., 2018). These studies are carried out for the design of strategies that allow reducing their dosage and application frequency, as well as making production systems more efficient from an economic and environmental perspective.
The preference between one technique and another depends on the sensitivity they may have when detecting different concentrations of nitrogen in the samples, the cost, the speed to obtain results, access to the equipment, and the complexity of the procedure. Typically, rhizosphere and plant root samples are used to isolate associated microorganisms in symbiosis, but when it comes to free-living nitrogenfixing organisms, soil samples are used (Angel et al., 2018). The sensitivity of the techniques is related to nitrogen availability in the sample, which in turn, depends on the nitrogen-fixing capacity of the microorganism present in association with the plant or if it is a free-living organism. Therefore, if it is high, it is easily detectable, as observed when using acetylene reduction and 15N isotopic dilution (Angel et al., 2018). On the other hand, it may differ by evaluated crop, e.g., with the natural abundance technique, a different percentage of nitrogen derived from the atmosphere has been observed, with values ranging from 12.8 % to 19.4 % for sugarcane, 22.4 % for corn, 20.9 % for Guinea grass, and from 24.7 % to 31.2 % for sorghum (De Carvalho et al., 2017). Concerning legumes such as soybean with values up to 65 % in local species and 53 % in improved varieties (Van Vugt et al., 2018), these results are due to the high nitrogenase activity in species that nodulate. The same has been observed in trials with common beans, highlighting differences in the percentage of nitrogen fixed in roots and grain samples by genotype (Polania et al., 2016).
These techniques, just like the nifH gene detection test, are used to detect diazotrophs and analyze their activity in the soil associated with permanent and transient cultures (table 3). However, ARA is not as sensitive in samples where the diazotrophic activity is low or sporadic, compared to the isotopic dilution method (Angel et al., 2018). Due to this variability, it is common to confirm the diazotrophic capacity by detection of the nifH gene, independent of the culture origin of the samples or their nature with a complementary morphological characterization.
Of course, plant characteristics such as the ability to produce ureides and the presence of nodules only in legumes, limit the application of some techniques for plants that produce them or failing to make modifications that consist in using much more aggressive reagents, and increase or decrease their concentration or exposure time. Thus, in permanent crops such as eucalyptus that is characterized by having a higher hardness in the roots due to its productive cycle, modification in the root sample treatment for analysis of endophytic microorganisms has been reported (Fonseca et al., 2017).
Such modification includes exposure to UV light for 5 min, washing with 70 % ethanol for 1 min and 2 % sodium hypochlorite for 2 min, to prepare root samples before isolating endophytic microorganisms (Fonseca et al., 2017); this test was adapted from a citrus sample trial; on the other hand, on root samples of grasses such as switchgrass (Panicum virgatum L.) only surface sterilization with 1.5 % sodium hypochlorite is stated (Roley et al., 2018). This indicates that the sample preparation is a decisive step that is usually adapted according to the characteristics of the material evaluated.
Conclusions
Since 1967, the use of several techniques to estimate the nitrogen fixation rate in agricultural research has increased with at least one publication per year, adjusting to a sigmoidal growth model with a point of decline towards the year 2053. In this way, this technology is in a rapid growth phase characterized by the appearance of modifications, innovations, and new methods, such as microbial bioassay, GlnLux test, and the latest generation molecular techniques, to analyze the dynamics of nitrogen in agricultural systems and the factors involved in the cycle. This reflects the economic effort to strengthen its market and dissemination from developed countries, which holds the property of these techniques, as well as to achieve the recognition of its products from its adaptation to the needs of research due to the disadvantages that have arisen during its application.
The procedures and sensitivities of the techniques used to determine the nitrogen fixation rate in the soil differ, which is related to their rusticity and detection capacity, but it also influences the type of sample and the procedure of its collection. In general, indirect techniques such as the number of nodules and nodular mass are unreliable, and nitrogen fixation can be overestimated or underestimated because the detection rate is low; however, there is a close relationship between the nodular mass and the fixed nitrogen content. Therefore, the most sensitive method at present, is isotopic dilution, because, with this method, it is possible to identify low rates of 15N, which are not achieved with the ARA or nodular mass methods. Despite this, it is difficult to control all external factors and agents involved in the nitrogen cycle, since it is usually episodic-like any biogeochemical process, so the action of nitrogen-fixing microorganisms can occur only when they have sufficient humidity and mineral elements.
All this explains the results of the maximum agronomic response of crops that are established under good fertility conditions. Therefore, there is a probability of error in all techniques, and perhaps, the most reliable is the identification of genes; however, the most significant limitation is the cost and the technology necessary for its application, although due to competition in the market, these tests have reduced its commercial price. Nevertheless, and despite their specificity, it is necessary that they are used jointly with some of the mentioned tests in this study.
As future scenarios, it is expected that technologies that are easy to use in the field will be used more frequently since the tests carried out under controlled conditions do not always reflect natural dynamics, because nitrogen varies in the crop and the soil due to edaphoclimatic and management factors. We propose the implementation of techniques that allow establishing the transformations of nitrogen after its application depending on the soil characteristics, the climate and the crop for the efficient use of nitrogen; this, together with cuttingedge technologies that use software to predict soil fertility from the analysis of historical results of nitrogen dynamics in the agricultural system, its modeling and estimation of the amount of fertilizer to be applied. However, older techniques will continue to be used due to its extensive dissemination in developing countries, and especially those that are cheaper.
Acknowledgments
The authors express their gratitude to the general royalty system [Sistema General de Regalías], the government of the department of Boyacá, Colciencias, and Universidad del Cauca. This document was elaborated within the development framework call number 779 of 2017.
REFERENCES
Aguilar, S., Ávalos, A. F., Giraldo, D. P., Quintero, S., Zartha, J. W., & Cortés, F. B. (2012). La curva en S como herramienta para la medición de los ciclos de vida de productos. Journal of Technology Management & Innovation, 7(1), 238-249. doi:10.1016/j.bjm.2017.03.007. [ Links ]
Akemi, M., Soares, T., Carvalho, D., Azarias, A., Martins, E., … Moreira, D. S. (2017). Tripartite symbiosis of Sophora tomentosa, rhizobia and arbuscular mycorrhizal fungi. Brazilian Journal of Microbiology, 48(4), 680-688. doi:10.1016/j.bjm.2017.03.007. [ Links ]
Angel, R., Panhölzl, C., Gabriel, R., Herbold, C., Wanek, W., … Woebken, D. (2018). Application of stable-isotope labelling techniques for the detection of active diazotrophs. Environmental Microbiology, 20(1), 44-61. doi:10.1111 /1462-2920.13954. [ Links ]
Argaw, A., & Mnalku, A. (2017). Symbiotic effectiveness of Rhizobium leguminosarum bv. vicieae isolated from major highland pulses on field pea (Pisum sativum L.) in soil with abundant rhizobial population. Annals of Agrarian Science, 15(3), 410-419. doi:10.1016/j.aasci.2017.04.005. [ Links ]
Arshi, A. (2017). Reclamation of coalmine overburden dump through environmental friendly method. Saudi Journal of Biological Sciences, 24(2), 371-378. doi:10.1016/j.sjbs.2015.09.009. [ Links ]
Bilal, M., Ayub, M., Tariq, M., Tahir, M., & Nadeem, M. A. (2017). Dry matter yield and forage quality traits of oat (Avena sativa L.) under integrative use of microbial and synthetic source of nitrogen. Journal of the Saudi Society of Agricultural Sciences, 16(3), 236-241. doi:10.1016/j.jssas.2015.08.002. [ Links ]
Bionda, C., Babini, S., Martino, A. L., Salas, N. E., & Lajmanovich, R. C. (2018). Impact assessment of agriculture and livestock over age, longevity and growth of populations of common toad Rhinella arenarum (Anura: Bufonidae), central area of Argentina. Global Ecology and Conservation, 14, 1-12. doi:10.1016/j.gecco.2018.e00398. [ Links ]
Braun, J., Mooshammer, M., Wanek, W., Prommer, J., Walker, T. W. N., … Richter, A. (2018). Full 15 N tracer accounting to revisit major assumptions of dilution approaches for gross nitrogen mineralization N isotope pool. Soil Biology and Biochemistry, 117, 16-26. doi:10.1016/j.soilbio.2017.11.005. [ Links ]
Camelo-Rusinque, M., Moreno-Galván, A., Romero-Perdomo, F., & Bonilla-Buitrago, R. (2017). Desarrollo de un sistema de fermentación líquida y de enquistamiento para una bacteria fijadora de nitrógeno con potencial como biofertilizante. Revista Argentina de Microbiología, 49(3), 289-296. doi:10.1016/j.ram.2016.06.005. [ Links ]
Carter, A. M., & Tegeder, M. (2016). Increasing nitrogen fixation and seed development in soybean requires complex adjustments of nodule nitrogen metabolism and partitioning processes. Current Biology, 26(15), 2044-2051. doi:10.1016/j.cub.2016.06.003. [ Links ]
Castanheira, N. L., Dourado, A. C., Pais, I., Semedo, J., Scotti-Campos, P., Borges, N., … Fareleira, P. (2017). Colonization and beneficial effects on annual ryegrass by mixed inoculation with plant growth promoting bacteria. Microbiological Research, 198, 47-55. doi:10.1016/j.micres.2017.01.009. [ Links ]
Chalk, P. M., & Craswell, E. T. (2018). An overview of the role and significance of 15 N methodologies in quantifying biological N 2 fixation (BNF) and BNF dynamics in agro-ecosystems. Simbiosis, 75(1), 1-16. doi:10.1007/s13199-017-0526-z. [ Links ]
Chauhan, A., Guleria, S., Balgir, P. P., Walia, A., Mahajan, R., … Shirkot, C. K. (2017). Tricalcium phosphate solubilization and nitrogen fixation by newly isolated Aneurinibacillus aneurinilyticus CKMV1 from rhizosphere of Valeriana jatamansi and its growth promotional effect. Brazilian Journal of Microbiology, 48(2), 294-304. doi:10.1016/j.bjm.2016.12.001. [ Links ]
Das, S., & De, T. K. (2018). Microbial assay of N2 fixation rate, a simple alternate for acetylene reduction assay. MethodsX, 5, 909-914. doi:10.1016/j.mex.2017.11.010. [ Links ]
De Andrade, A., Albenísio, J., Bonifacio, A., Cerqueira, A., & Barreto, V. (2018). Antioxidant response of cowpea co-inoculated with plant growth-promoting bacteria under salt. Brazilian Journal of Microbiology, 49(3), 513-521. doi:10.1016/j.bjm.2017.12.003. [ Links ]
De Carvalho, E. X., Sim.es, R., Menezes, C., Dolores, A., De Freitas, S., … De Queiroz, R. O. (2017). The 15N natural abundance technique to assess the potential of biological (BNF) in some important C4 grasses. Australian Journal of Crop Science, 11(12), 1559-1564. doi:10.21475/ajcs.17.11.12.pne729. [ Links ]
Dilworth, M. J. (1966). Acetylene reduction by nitrogenfixing preparations from Clostridium pasteurianum. Biochim Biophys Acta, 127(2), 285-294 [ Links ]
Dutta, S., Datta, J. K., & Mandal, N. C. (2017). Evaluation of indigenous rhizobacterial strains with reduced dose of chemical fertilizer towards growth and yield of mustard (Brassica campestris) under old alluvial soil zone of West Bengal, India. Annals of Agrarian Science, 15(4), 447-452. doi:10.1016/j.aasci.2017.02.015. [ Links ]
Fonseca, S., Peixoto, R. S., Rosado, A. S., Balieiro, D. C., Tiedje, J. M., ... Rachid, C. (2017). The microbiome of eucalyptus roots under different management conditions and its potential for biological nitrogen fixation. Microbial Ecology, 75(1), 183-191. doi:10.1007/s00248-017-1014-y. [ Links ]
Fuertes-Mendizábal, T., Estavillo, J. M., Duñabeitia, M. K., Huérfano, X., Castellón, A., … González-Moro, M. B. (2018). 15 N natural abundance evidences a better use of N sources by late nitrogen application in bread wheat. Frontiers in Plant Science, 9(853), 1-11. doi:10.3389/fpls.2018.00853. [ Links ]
García, J. E., Maroniche, G., Creus, C., Suárez-Rodríguez, R., Ramirez-Trujillo, J. A., & Groppa, M. D. (2017). In vitro PGPR properties and osmotic tolerance of different Azospirillum native strains and their effects on growth of maize under drought stress. Microbiological Research, 202, 21-29. doi:10.1016/j.micres.2017.04.007. [ Links ]
Hamawaki, R. L., & Kantartzi, S. K. (2018). Fixaç.o de nitrogênio na fase inicial e final de desenvolvimento da soja. Acta Scientiarum - Agronomy, 40(1), 1-10. doi:10.4025/actasciagron.v40i1.36372. [ Links ]
Hashem, A., Kumar, A., Al-Dbass, A. M., Alqarawi, A. A., Al-Arjani, A. F., Singh, G., … Allah, A. (2018). Arbuscular mycorrhizal fungi and biochar improves drought tolerance in chickpea. Saudi Journal of Biological Sciences, 26(3), 614-624 doi:10.1016/j.sjbs.2018.11.005. [ Links ]
Jaiswal, S. K., Msimbira, L. A., & Dakora, F. D. (2017). Phylogenetically diverse group of native bacterial symbionts isolated from root nodules of groundnut (Arachis hypogaea L.) in South Africa. Systematic and Applied Microbiology, 40(4), 215-226. doi:10.1016/j.syapm.2017.02.002. [ Links ]
Kataria, S., & Baghel, L. (2016). Influence of UV exclusion and Selenium on Carbon fixation, Nitrogen fixation and yield of soybean variety JS-335. South African Journal of Botany, 103, 126-134. doi:10.1016/j.sajb.2015.09.003. [ Links ]
Khalifa, A. Y. Z., & AlMalki, M. (2018). Polyphasic characterization of Delftia acidovorans ESM-1, a facultative methylotrophic bacterium isolated from rhizosphere of Eruca sativa. Saudi Journal of Biological Sciences, 1-6. doi:10.1016/j.sjbs.2018.05.015. [ Links ]
Leite, J., Ribeiro, S., Sim.es-Araújo, J. L., Gouvêa, N., Ribeiro, G., & Édson, J. (2017). Genomic identification and characterization of the elite strains Bradyrhizobium yuanmingense BR 3267 and Bradyrhizobium pachyrhizi BR 3262 recommended for cowpea inoculation in Brazil. Brazilian Journal of Microbiology, 49(4), 703-713. doi:10.1016/j.bjm.2017.01.007. [ Links ]
Liu, H., Zhang, L., Meng, A., Zhang, J., Xie, M., … Qiu, L. (2017). Isolation and molecular identification of endophytic diazotrophs from seeds and stems of three cereal crops. PLoS ONE, 12(10), 1-11. doi:10.1371/journal. pone.0187383. [ Links ]
Mondal, T., Datta, J. K., & Mondal, N. K. (2017). Chemical fertilizer in conjunction with biofertilizer and vermicompost induced changes in morpho-physiological and bio-chemical traits of mustard crop. Journal of the Saudi Society of Agricultural Sciences, 16(2), 135-144. doi:10.1016/j.jssas.2015.05.001. [ Links ]
Mwenda, G. M., Hara, G. W. O., De Meyer, S. E., Howieson, J. G., & Terpolilli, J. J. (2018). Genetic diversity and symbiotic effectiveness of Phaseolus vulgaris -Nodulating rhizobia in Kenya. Systematic and Applied Microbiology, 41(4), 291- 299. doi:10.1016/j.syapm.2018.02.001. [ Links ]
Ndungu, S. M., Messmer, M. M., Ziegler, D., Gamper, H. A., Mészáros, É., … Thonar, C. (2018). Cowpea (Vigna unguiculata L. Walp) hosts several widespread bradyrhizobial root nodule symbionts across contrasting agro-ecological production areas in Kenya. Agriculture, Ecosystems and Environment, 261, 161-171. doi:10.1016/j.agee.2017.12.014. [ Links ]
Osei, O., Abaidoo, R. C., Ahiabor, B. D. K., & Boddey, R. M. (2018). Bacteria related to Bradyrhizobium yuanmingense from Ghana are effective groundnut micro-symbionts. Applied Soil Ecology, 127, 41-50. doi:10.1016/j.apsoil.2018.03.003. [ Links ]
Pai, M., Mcculloch, M., Gorman, J. D., Pai, N., Enanoria, W., Kennedy, G., … Colford, J. M. (2004). Systematic reviews and meta-analyses : an illustrated, step-by-step guide. Clinical Research Methods, 17(2), 86-95. [ Links ]
Polania, J., Poschenrieder, C., Rao, I., & Beebe, S. (2016). Estimation of phenotypic variability in symbiotic nitrogen fixation ability of common bean under drought stress using 15 N natural abundance in grain. European Journal of Agronomy, 79, 66-73. doi:10.1016/j.eja.2016.05.014. [ Links ]
Rampadarath, S., Bandhoa, K., Puchooa, D., Jeewon, R., & Bal, S. (2017). Early bacterial biofilm colonizers in the coastal waters of Mauritius. Electronic Journal of Biotechnology, 29, 13-21. doi:10.1016/j.ejbt.2017.06.006. [ Links ]
Ribeiro, D., Ferreira, A., Idaline, M., Cavalcanti, P., Elena, I., … Fernandes-júnior, P. I. (2018). Phenotypic, genetic and symbiotic characterization of Erythrina velutina rhizobia from Caatinga dry forest. Brazilian Journal of Microbiology, 49(3), 503-512. doi:10.1016/j.bjm.2017.09.007. [ Links ]
Rigonato, J., Kent, A. D., Gumiere, T., Henrique, L., Branco, Z., … Paulo, S. (2017). Temporal assessment of microbial communities in soils of two contrasting mangroves. Brazilian Journal of Microbiology, 49(1), 87-96. doi:10.1016/j.bjm.2017.04.008. [ Links ]
Ritchie, M. E., & Raina, R. (2016). Effects of herbivores on nitrogen fixation by grass endophytes, legume symbionts and free-living soil surface bacteria in the Serengeti. Pedobiologia, 59, 233-241. doi:10.1016/j.pedobi.2016.09.001. [ Links ]
Roley, S. S., Xue, C., Hamilton, S. K., Tiedje, J. M., & Philip, G. (2018). Isotopic evidence for episodic nitrogen fixation in switchgrass (Panicum virgatum L.). Soil Biology and Biochemistry, 129, 99-98. doi:10.1016/j.soilbio.2018.11.006. [ Links ]
Sainju, U. M. (2017). Determination of nitrogen balance in agroecosystems. MethodsX, 4, 199-208. doi:10.1016/j.mex.2017.06.001. [ Links ]
Santos, A. A., Silveira, J. A. G., Guilherme, E. A., Bonifacio, A., Rodrigues, A. C., & Figueiredo, M. D. V. B. (2018). Changes induced by co-inoculation in nitrogen–carbon metabolism in cowpea under salinity stress. Brazilian Journal of Microbiology, 49(4), 685-694. doi:10.1016/j.bjm.2018.01.007. [ Links ]
Satyanarayana, S. D. V, Krishna, M. S. R., Pavan, P., & Jeereddy, S. (2018). In silico structural homology modeling of nif a protein of rhizobial strains in selective legume plants. Journal of Genetic Engineering and Biotechnology, 16(2), 731-737. doi:10.1016/j.jgeb.2018.06.006. [ Links ]
Schoὂllhorn, R. & Burris, R. H. (1967). Acetylene as a competitive inhibitor of N2 fixation, Proceedings of the National Academy of Sciences, 58, 213-216 [ Links ]
Stewart, W. D., Fitzgerald, G. P., Burris, R. H., & Nov, N. (1967). In situ studies on N2 fixation using the acetylene reduction. Proceedings of the National Academy of Sciences of the United States of America, 58(5), 2071-2078. [ Links ]
Tahon, G., & Willems, A. (2017). Isolation and characterization of aerobic anoxygenic phototrophs from exposed soils from the Sør Rondane Mountains, East Antarctica. Systematic and Applied Microbiology, 40(6), 357-369. doi:10.1016/j.syapm.2017.05.007. [ Links ]
Tai-wen, Y., Ping, C., Quian, D., Quing, D., Feng, Y., Xiao-chun, W., Wei-guo, L., & Wen-yu, Y. (2018). Optimized nitrogen application methods to improve nitrogen use efficiency and nodule nitrogen fixation in a maize-soybean relay intercropping system. Journal of Integrative Agriculture, 17(3), 664-676. doi: 10.1016/S2095-3119(17)61836-7. [ Links ]
Tchuisseu Tchakounté, G. V., Berger, B., Patz, S., Fankem, H., & Ruppel, S. (2018). Community structure and plant growth-promoting potential of cultivable bacteria isolated from Cameroon soil. Microbiological Research, 214, 47-59. doi:10.1016/j.micres.2018.05.008. [ Links ]
Thilakarathna, M. S., Moroz, N., & Raizada, M. N. (2017). A biosensor-based leaf punch assay for glutamine correlates to symbiotic nitrogen fixation measurements in legumes to permit rapid screening of rhizobia inoculants under controlled conditions. Frontiers in Plant Science, 8, 1-11. doi:10.3389/fpls.2017.01714. [ Links ]
Thilakarathna, M. S., & Raizada, M. N. (2018). Challenges in using precision agriculture to optimize symbiotic nitrogen fixation in legumes: progress, limitations , and future improvements needed in diagnostic testing. Agronomy, 8(5), 1-21. doi:10.3390/agronomy8050078. [ Links ]
Van Vugt, D., Franke, A. C., & Giller, K. E. (2018). Understanding variability in the benefits of N2-fixation in soybean-maize rotations on smallholder farmers’ fields in Malawi. Agriculture, Ecosystems and Environment, 261(36), 241-250. doi:10.1016/j.agee.2017.05.008. [ Links ]
Wambeke, F. Van, Gimenez, A., Duhamel, S., Dupouy, C., & Lefevre, D. (2018). Dynamics and controls of heterotrophic prokaryotic production in the western tropical south Pacific ocean: links with diazotrophic and photosynthetic activity. Biogeosciences, 15(9), 2669-2689. doi:10.5194/bg-15-2669-2018. [ Links ]
Yan, K., Ranjitkar, S., Zhai, D., Li, Y., Xu, J., … Lu, Y. (2017). Current re-vegetation patterns and restoration issues in degraded geological phosphorus-rich mountain areas: a synthetic analysis of central Yunnan, SW China. Plant Diversity, 39(3), 140-148. doi:10.1016/j.pld.2017.04.003. [ Links ]
Yong, T. W., Chen, P., Dong, Q., Du, Q., Yang, F., Wang, X. C., … Yang, W. Y. (2018). Optimized nitrogen application methods to improve nitrogen use efficiency and nodule nitrogen fixation in a maize-soybean relay intercropping system. Journal of Integrative Agriculture, 17(3), 664-676. [ Links ]
Received: April 03, 2019; Accepted: September 04, 2019