Introducción
The coexistence of agro-energy, food security, and environmental protection amidst climate change, environmental degradation, food crisis, and the “biofuels vs. food” contradiction is still a challenge (Suárez et al., 2014; Suárez-Hernández, Quevedo-Benkí, et al., 2018). However, biofuels are also promoted as an environmentally friendly alternative to fossil fuels for reducing greenhouse gases and promoting agricultural development, which is the strength of integrated farming systems. They can produce food, biofuels, and multiple products from biomass, whose total value-added can be greater than that generated by fossil fuels (Fernandez et al., 2018; Koppelmäki et al., 2019).
Agro-wastes could play an essential role in future energy supply, mainly through thermochemical, physicochemical, biochemical transformations, and conventional combustion. In this sense, biogas, biodiesel, and bioethanol are some of the candidate alternative fuels from biochemical transformations receiving wide attention because of their inherent “green” potential (Koryś et al., 2019; Martínez-Gutiérrez, 2018; Nzila et al., 2010). Nevertheless, in terms of technological and social-economic considerations, biogas technology is preferred due to its economic feasibility (Kelebe, 2018), sustainability potential (Guerini Filho et al., 2019), and ability to meet all the Sustainable Development Goals (Clemens et al., 2018; Dada & Mbohwa, 2018; Yasmin & Grundmann, 2020). Also, biogas technology is a mature technology and offers a very appealing route to utilize diverse categories of biomass (Martínez-Gutiérrez, 2018; Theuerl et al., 2019) and the inherent biowaste for meeting energy needs as well as contributing to resource and environmental conservation. Biogas is suitable for covering small scale energy needs, influencing environmental sanitation (Abdul Aziz et al., 2019; Garfí et al., 2016; Silaen et al., 2020), and simple enough to avoid production limitations.
According to the new economic model in Cuba, the energetics policy proposed a change in the energetic matrix for 2030 by using 24 % of renewable energy source contribution (Arrastía-Avila & Glidden, 2017; Korkeakoski, 2021; Vazquez et al., 2018). Nonetheless, it only considers wind, photovoltaic, hydroelectric energy, and biomass combustion in sugar cane factories. Biogas or methane, which has been mainly limited to farm-scale systems for waste treatment until the end of the 20th (Björkman, 2020; Suárez-Hernández, Sosa-Cáceres, et al., 2018), has not been considered an input for this 24 %.
Anaerobic digestion technology was introduced in Cuba in the early 1980s, with the purely environmental objective of treating livestock waste and residuals in sugar factories and their derivatives (Björkman, 2020). There are currently several anaerobic digestion plants (which mainly treat livestock waste, co-digestion of livestock waste, and co-digestion with agricultural biomass) whose purpose is to produce biogas for community use (Cano-Ricardo et al., 2019; Odales et al., 2020; Suárez-Hernández, Quevedo-Benkí, et al., 2018). The most widespread digesters are the Chinese and Indian types, on a small and medium scale, whose common disadvantages are the long hydraulic retention times and low substrate conversion efficiency, resulting in a low volumetric biogas production rate (Barrera-Cardoso et al., 2020; Cano-Ricardo et al., 2020; Hermida García et al., 2020; Suárez-Hernández, Sosa-Cáceres, et al., 2018).
On a larger scale, there are few examples of anaerobic digestion plants in the country. One of these recently set up plants is composed of an anaerobic lagoon covered with a high-density synthetic geomembrane and a capacity of 4,500 m3 , located in the Martí municipality, Matanzas province (Suárez-Hernández, Quevedo-Benkí, et al., 2018). This lagoon is locally known by its project name “Martí II” (financed by the EU and with technology from Aqualimpia Engineering e.K.) and operates with pig slaughterhouse waste and pig manure on a complete mixing tank basis and has a current electricity generation potential of 120 kW. The project foresees the completion of a second covered lagoon (Martí I) by 2021, which would have a capacity of 2,500 m3 and operate on the same principles as the first one (Suárez-Hernández, Quevedo-Benkí, et al., 2018).
Other previous plants are the ones used for vinasse treatment at the Heriberto Duquesne distillery in Villa Clara (created in 2004), using a 3,000 m3 up-flow anaerobic sludge bed reactor (UASB) with a production of 16,000 m3 of biogas for boilers in the distillery and cooking food (Barrera et al., 2020). Another large-scale plant is located at the 100th Street Landfill in Havana (created in 2008), which uses a completed stirred tank reactor (CSTR) to treat solid waste from agro markets and tree pruning, with the possibility of delivering 60 kWh of electrical energy. However, in the last two cases, the inefficient management of the anaerobic process has been verified, preventing the plants from working at full capacity.
With the development of decentralized swine production, anaerobic digestion technology has played a crucial role in treating manure to obtain biogas for energy purposes, fundamentally for cooking (Kelebe, 2018; Nevzorova & Kutcherov, 2019). Nevertheless, despite the experiences in the subject, in the authors’ opinion, several criteria have contributed to the low efficiency of these technologies, which has limited their use for power energy purposes, including a) the use of Chinese and Indian technologies, whose designs do not take into account agitation (intermittent, mechanical or biogas), b) the limited use of inoculums or active sludge (Li et al., 2010), and c) the minimal physical-chemical and microbiological control of the process. The issue constitutes a challenge for Cuban scientists, as it requires anaerobic digestion optimization studies to improve methanogenesis from the different sources of waste available, predominantly agricultural and industrial biomass.
Agricultural biomass, such as straw, has a high carbon content, and its anaerobic degradation for methane production has been studied by several authors (Contreras Velázquez et al., 2019; Kainthola et al., 2019; Moustakas et al., 2020; Yu et al., 2019). Some dry fermentation technologies have been developed to treat solid wastes (Contreraz Velazquez et al., 2012; Momayez et al., 2019; Samer et al., 2020; Stehel et al., 2020; Weiland, 2007), including type reactors designed for fermentation of solids such as corn silage and other energy crops (Herrero García et al., 2019; Nurk et al., 2017; Weiland, 2007). However, these technologies are still too expensive for developing countries like Cuba. In this sense, a CSTR to treat agro-solid waste in co-digestion with slurry would be ideal for the Cuban scenario. It would combine the benefits of the carbon source from biomass with the nutrients (e.g., nitrogen and phosphorus) and microorganisms from slurry. Nonetheless, the experimental biochemical methane potential (BMP) of Cuban biomass has not yet been studied.
This work aims to analyze the agro-waste biogas potential as an alternative for renewable energy production in Cuba. The agro-wastes were biochemically characterized, determining their energy potential using BMP assays. Based on the results, the energy power potential and its economic feasibility were determined as renewable energy sources for the context of the new Cuban economic model.
Materials and methods
Sources of agro-wastes and materials
Agricultural residues (substrates) used in this work (rice straw, cane straw, corn straw, bean straw, banana straw (leaves), coffee husk, and parchment coffee) were collected in farming areas of Sancti Spíritus province, Cuba. The substrates selected for the research are the most representative (biomass) from the provincial and national agro-industry (ONEI, 2019b). In the case of the sugar cane bagasse case, it was collected from the leaf cleaner center at the Melanio Hernández Sugar Cane Factory located in Sancti Spíritus.
Approximate 10 kg of each residue was collected using the quarter methods (NMX-AA-15-1985) described previously by several authors (Filippín et al., 2017; Hernández-Cobián & Rivera-Sasso, 2017), subsequently air-drying them at ambient conditions in a laboratory to prevent the possible growth of fungi. For the chemical analysis and BMP assays, all the agro-wastes were milled and homogenized by a laboratory household blender (Severing Elektrogeräte GmbH). The agrowastes blended were sieved through 5 mm mesh, achieving an average 2-5 mm particle size. Table 1 shows the main agro-waste characteristics.
Table 1. Agro-waste characteristics.
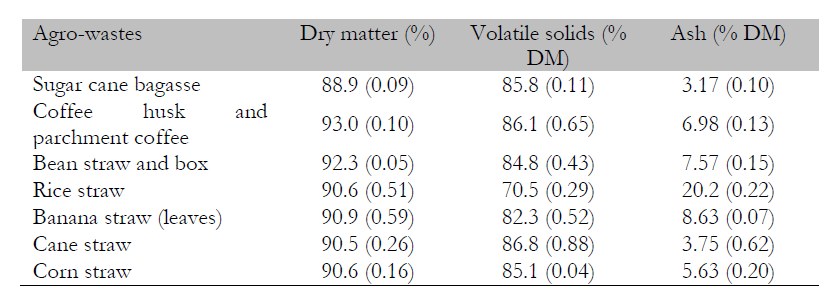
Note.Standard deviations (SD) in parentheses (n = 3).
Source: Elaborated by the authors
Anaerobic sludge from a lab-scale CSTR (10 L) treating pig manure as monosubstrate was used as inoculum for batch assays. The main characteristics of this inoculum were 5.31 % total solids (TS), 3.59 % total volatile solids (VS), and 1.72 % ash.
BMP assays T3
The BMP assays were carried out in 585 ml serological bottles according to German standard methods VDI 4630 (Carabeo-Pérez et al., 2021; VDI 4630, 2016; Weinrich et al., 2018). The bottles were filled with 160 ml of culture media (containing K2HPO4 0.50 g/l; KH2PO4 0.33 g/l; NH4Cl 0.20 g/l; MgCl2·6H2O 0.10 g/l; CaCl2·2H2O 0.15 g/l; NaCl 0.10 g/l; FeSO4·6H2O 0.01 g/l; Na2S·9H2O 0.02 g/l; NaHCO3 3.0 g/l; vitamin solution 10 ml/l; micronutrients solution 10 ml/l; Cysteine and Na2S 1 g/l). The pH was adjusted between 6 and 7 using a phosphate buffer. Then, 40 mL of inoculum (pig manure anaerobic sludge) and an amount of each agro-waste (0.88 g for coffee husk and parchment coffee / 1.19 g for rice straw) were added to each bottle, which represents an organic load of 0.5 g/l of total volatile solids, and a substrate/inoculum ratio of 0.5 according to German standard methods (VDI 4630, 2016). The bottles were sealed immediately using a rubber cap, assaying three replicates per agro-waste typology. All bottles were incubated at mesophilic conditions (35 ± 2 °C) for 30 days. Two bottles filled with inoculum without substrate (B1) were used as control. Gas production was measured manually with syringes, discarding the first gas measurements (taken at 24 hours), as described by Nzila et al. (2010).
Chemical analysis T3
The agro-wastes were characterized in terms of dry matter (DM), VS, ash, pH, total alkalinity, and ammonia nitrogen (N-NH4+), according to the standard methods (Rice et al., 2017). The concentration of volatile fatty acids (VFA) was determined by a gas chromatograph (Focus Thermo Scientific, with flame ionization detector FID, column Restek Stabilwax-DA, and hydrogen as carrier gas). For VFA analysis, 2 ml of each sample were transferred to vials containing 0.5 mL sulfuric acid (50 %), 0.4 g NaCl, 0.5 ml acrylic acid (2,100 mg/l), and 2 ml diethyl ether. The vials were vortexed and centrifuged. The top layer (ether) was transferred to another vial for chromatographic analysis of VFAs. The VFA to alkalinity ratio (VFA/TA ratio) was also determined (VDI 4630, 2016).
Biogas production was measured daily using the water displacement method, considering the pressure and temperature measurements for normalization (Karray et al., 2017; Muske & Venkateswara Rao, 2019). During the digestion period, the reactors were mixed manually every day before measuring gas. The biogas volume (Vbiogas) and the methane volume (𝑉𝐶𝐻4 ) in ml per gram of VS were determined as Nzila et al. (2010) described in their work and adapted to the context of this study, using Equations (1) and (2), respectively.
Where
ΔP = change of pressure in the reactor (kPa) after correcting for temperature changes
Vh = reactor headspace volume (l)
Vmol= molar gas volumen at 308 K (l/mol)
R = universal gas constant (8.31 kPa l/molK)
T = temperature (308 K)
Where
ηCH4= CH4 fraction in biogas
V’biogas = biogas volume produced by the substrate (l)
V“biogas = biogas volume in the blank reactor (l).
Determination of the amount of biomass waste and potential methane T
Waste quantities available were calculated using the national production data from the National Bureau of Statistics and Information database of the Republic of Cuba (ONEI, 2019a).
The available potential residue (𝑅𝐴𝑟𝑒𝑎𝑙) was calculated from the maximum residue output potential (𝑅𝐴𝑚𝑎𝑥) and the profitable (spatial location, the proximity of the plant, the economic feasibility of biomass transport, interest in its use) waste fraction (𝛿) (Equation (3)) (Nzila et al., 2010). The 𝛿 constant was estimated as 50 % for each biomass used (Chandra et al., 2012). Equations (4) and (5) were used to calculate the maximum and available methane potential from the agro-wastes (𝑅𝐴𝐶𝐻4𝑚𝑎𝑥 and 𝑅𝐴𝐶𝐻4𝑟𝑒𝑎𝑙) in m3 CH4/yr., respectively.
Economic and energetic viability analysis of BMPs
Based on the results of the BMP, and assuming that it is used in a combined heat and power (CHP) system, the energetic potential (electricity and heat) per cubic meter of biogas will be determined. Furthermore, its economic feasibility as a renewable energy source in the Cuban context will be assessed. According to the EPA (2019), the overall efficiency of the cogeneration system (CHP) is 75 %, where the conversion efficiency to electricity and thermal energy is 30 and 45 %, respectively (EPA, 2019; Nzila et al., 2010), producing 2.98 kWh of electricity per 1 m3 CH4 and 4.48 kWh of thermal energy (EPA, 2019). The rates used in this study ($0.36 by 1 kWh per peak hour and $0.196 by 1 kWh per 24 hours) are the same rates for the purchase of electricity generated from biofuels according to the time tables established by Unión Eléctrica de Cuba (UNE, for its acronym in Spanish, a Cuban State Enterprise) to its suppliers (mainly entities subordinated to the AZCUBA sugar group) and approved by Resolution MFP No 425/2011. Other information used in the study, such as electricity generation, electricity consumption, and fuel consumption, the petroleum equivalence ratio, and the cost of petroleum in Cuba, was taken from the Cuban National Bureau of Statistics and Information database (ONEI, 2019a).
Results and discussion
BMP of agro-wastes
The agro-wastes characterization showed TS and VS values in the same order of magnitude for sugar cane bagasse, coffee husk and parchment coffee, bean straw and box, rice straw, banana straw (leaves), cane straw, and corn straw (table 1). The VS/DM ratio suggests the biodegradable fraction of the substrates used for biogas production. Therefore, it is expected that because of its lower VS content, rice straw should produce a lower amount of biogas, and in contrast, cane straw should produce a higher amount. However, as Xu et al. (2019) pointed out, this assumption does not consider substrate composition’s possible effect and biodegradability.
The parameters evaluated at the end of the experiments (table 2) were similar among the seven different agro-waste types assayed. The range values of pH (7.19-7.68), alkalinity (3.00-3.86·10-3 mg CaCO3/l), ammonia nitrogen (0.08-0.28·10-3 N-NH4+ mg/l FM), and the VFA/TA ratio (0.27-0.38) showed the proper conditions for agro-waste anaerobic digestion in all cases (Mussoline et al. 2012). The removal of organic matter was achieved in a range from 53 to 63 %. According to the results published by other researchers, the percentages of organic load reduction for the agro-waste studied are in the 52-73 % range (Corro et al., 2014; Darwin et al., 2014; Sajad Hashemi et al., 2019). The higher value corresponds to banana straw and the lower for sugar cane straw at a retention time of 30 days.
Table 2. Parameters evaluated at the end of the experiments
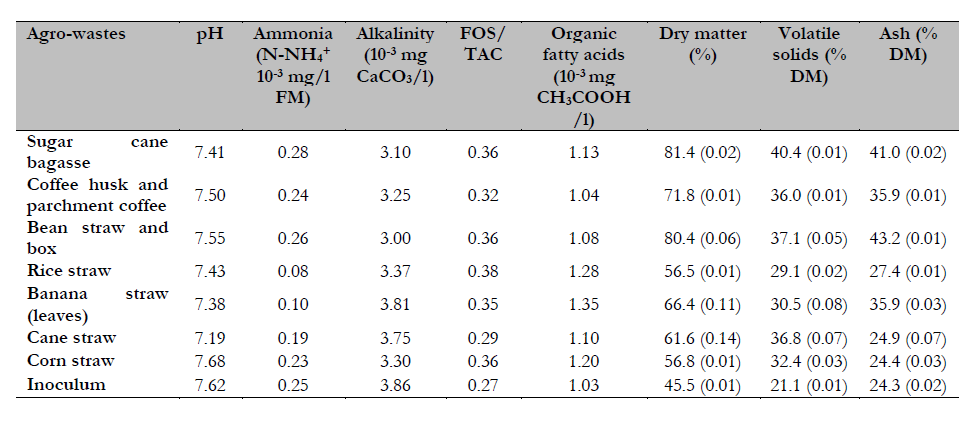
Note.Standard deviations (SD) in parentheses (n = 3).
Source: Elaborated by the authors
The VFA profile differences among agro-wastes were in accordance with the methane yields and VS removal (figure 1). The higher VFA accumulation in the case of rice straw is probably the outcome of its higher lignocellulosic content, which hampers its degradation. Contreraz Velazquez et al. (2012) showed similar results, indicating that the substrate requires longer than 35-day treatment for the microorganism to transform all organic matter present. Other authors point to the need for pre-treatment, either chemical-physical or biological, to increase biodegradability and thus increase methane yields per g of volatile solids (Martínez-Gutiérrez, 2018; Mussoline et al., 2013; Xu et al., 2019).
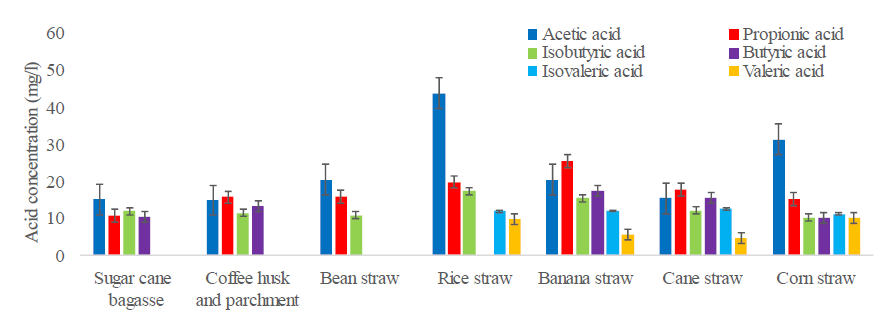
Source: Elaborated by the authors
Figure 1. Cumulative VFAs at the end of experiments. The bars indicate the standard sampling error where three samples were analyzed per substrate. A simple classification variance analysis and the Duncan Multiple Range Test were used for a 5 % error probability level.
The BMP of each agro-waste showed that corn straw rendered the highest yield, with average values of 416.6 ± 14.0 ml CH4/gSV, while coffee husk and parchment coffee showed the lowest yield, with an average of 114.8± 6.2 ml CH4/gSV (figure 2). The BMP from each agro-waste (table 3) is pretty comparable to BMP values of cultures used for energy production in other studies reported at similar conditions and inoculum (Chandra et al., 2012; Corro et al., 2014; Darwin et al., 2014; Kaur et al., 2020; Mustafa et al., 2018; Noonari et al., 2019; Nosratpour et al., 2018; Nurk et al., 2017; Pazmiño-Hernandez et al., 2019; Petersson et al., 2007; Sajad Hashemi et al., 2019; Villa Gomez et al., 2019). Other authors achieved similar values using other inoculum sources (Guerini Filho et al., 2019; Marchetti et al., 2016; Nzila et al., 2010). They also point out the need to pre-treat such biomass to increase biodegradability and biogas yield by scaling up the technology. Therefore, Cuba’s agricultural residues can be used as a source for energy or electricity production, closing the loop in agriculture production.
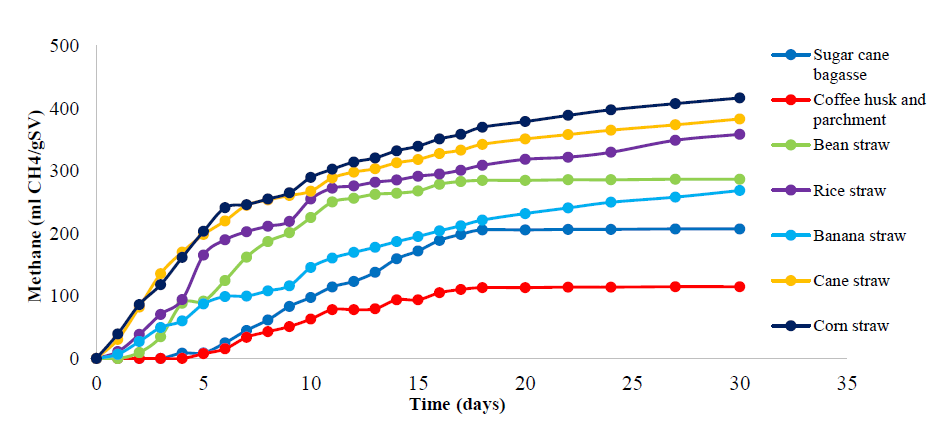
Source: Elaborated by the authors
Figure 2. Cumulative methane production of each agro-waste assayed
Table 3. Comparison of the biochemical methane potential (BMP) values obtained from this study and those reported in the literatura
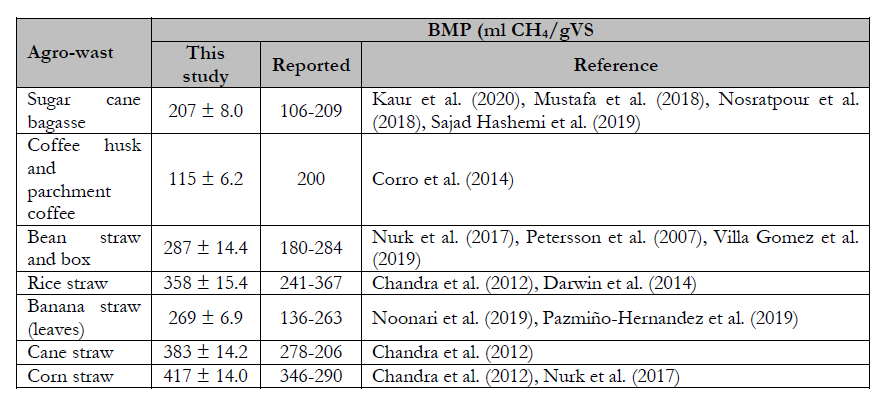
Source: Elaborated by the authors
It should be noted that the lag phase for each agro-waste methane production: (rice straw, sugarcane, corn, beans, and banana) began one day after the initiation of the experiment (figure 2), for sugarcane bagasse four days after, and for coffee husk and parchment coffee five days after. This delay in methane production suggests that the population of methanogens may not have been high enough until day four or five. Also, the structural characteristic of the substrate (lignocellulose raw) may difficult hydrolytic bacteria mass transfer, therefore limiting the hydrolytic step of methanogenesis (Mussoline et al., 2013). An example is the rice straw case; it has one of the highest DM contents (90.6 ± 0.51 %) but the lowest VS content, showing its high fiber (e.g., lignin) content. Methane yield was lower than expected for coffee husk and parchment coffee waste, as it has higher VS % values than corn and rice straw residues (table 3). The methane yield from rice straw waste (lower VS %) was three times greater than coffee husk and parchment coffee waste (figure 2). Other authors (Corro et al., 2014) have reported that liquid coffee waste produced better digestible leachate than solid coffee waste, having a tremendous potential for methane. Coffee solid waste contains inhibitors and methanogenesis substances such as tannins that make it less digestible and is obtained for the corresponding methane production, as Xu et al. (2019) pointed in their study.
These results are consistent with the lower accumulated methane from coffee husk and parchment coffee waste (115 ml CH4/g VS) (figure 2) and average methane biogas (46 %). Therefore, due to the geographical and relief characteristics of the crop, solid coffee waste is less competitive to produce methane and energy compared to other residues investigated in this study. However, a more practical use for solid coffee waste could be the bioremediation of soils contaminated by metals (Corro et al., 2014).
Biogas power energy potential T3
From the agro-waste tested, the potential for electricity and heat obtained per cubic meter of biogas is depicted in table 4 when a CHP system is used. Compared to conventional electricity and thermal energy production, CHP offers efficiency, environmental, economic, and reliability benefits (EPA, 2019).
Table 4. Energy power potential of biogas using the agro-wastes evaluated as feedstock
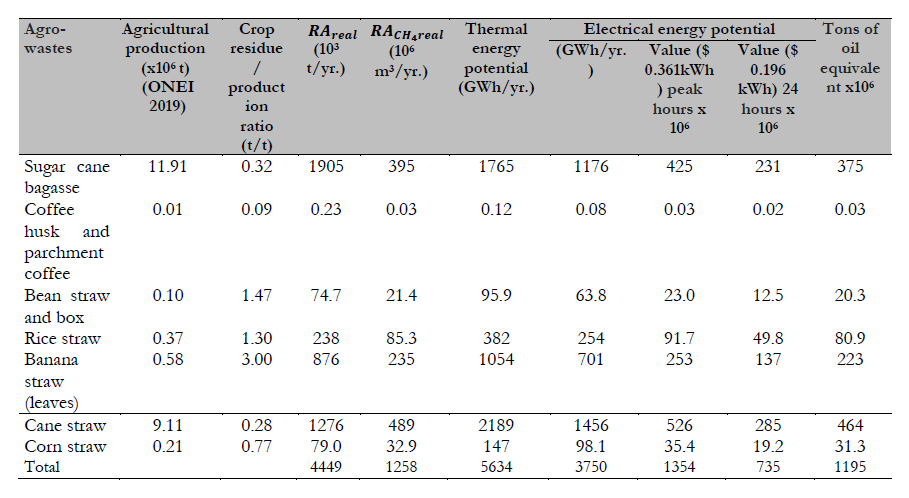
Source: Elaborated by the authors
The total electricity generation in 2018 in Cuba remained at 20,837 GWh (ONEI, 2019a), with 98.5 % coming from fossil fuels. Hence, the potential annual electricity from the selected agricultural residue (3749.61 GWh) is equivalent to about 18.0 % of Cuba’s total annual electricity generation. The electric potential of the waste evaluated in this study could replace 40.5 % of the electricity consumed by households (9,257 GWh/yr., table 5) (ONEI, 2019a). Furthermore, decentralizing power generation would decrease power losses by up to 3,290 GWh/yr. for transmission (ONEI, 2019a).
When using a residual factor of 50 %, the potential annual generation of electricity from agricultural residues was 4.3 times greater than that produced by self-producers (sugar and nickel industries) and 12 times the electrical energy generated by renewable sources currently exploited (ONEI, 2019a). The methane obtained as a renewable energy source alternative in the new Cuban economy model could replace 23 % of tons of petroleum, corresponding to 538·109 USD (ONEI, 2019a) and representing more than 100 % of the gas (natural and manufactured) used in 2018 (equivalent to 1007·109 USD) (ONEI, 2019a) (table 5). Using crop residues for energy production would positively impact the economy through income generation and savings resulting from reductions of imports, employment creation, improved living conditions, and development. In addition, the electricity generated from methane would be a renewable energy source in response to the new Cuban policies.
Conclusions
This study demonstrates the potential of using anaerobic digestion for biogas production from solid agro-wastes: sugarcane straw, rice straw, corn straw, banana straw, bean straw, coffee husk, and sugarcane bagasse generated in Cuba. Solid agro-wastes can be degraded under mesophilic conditions by co-digestion using adapted pig manure sludge as inoculum. The adapted pig manure inoculum analyzed in this study showed better biogas production, probably due to the improvement in the balance of nutrients and their availability at the temperature of digestion. It is vital to determine the biodegradability of biomass because the content of volatile solids alone is not enough to predict biodegradability. BMP values for the types of waste studied ranged from 0.03 million m3 /yr. to 489 m3 /yr.
Moreover, waste from corn straw, sugarcane straw, and rice straw showed better qualities of digestibility than coffee husk, parchment coffee, and sugarcane bagasse, with the bean straw and banana straw demonstrating average digestibility. These residues can be used to supplement or co-digest with manure in biogas reactors, especially for intensive agricultural regions in Cuba. The study’s authors consider that biogas production in Cuba should be considered more than a small-scale generation or a simple treatment system. Based on the results, they determined the excellent energy potential and the economic viability as a renewable energy resource that biogas production has in the context of the new Cuban economic model. The total electricity generation from this waste (3750 GWh/yr.) can replace 18.0 % of the energy consumed, 23 % of crude oil purchased (equivalent to 100 % of the gas used. The results of this research are encouraging and can be used in system decision-making to support this technology on a larger scale for energy purposes. Cuban biogas production can and should be included in the group of renewable energy generation sources to achieve the goal of replacing 24 % of fossil fuel use in electric energy production by 2030.