Introduction
The magnetic treatment of water has been used more widely for industrial purposes. There is a wide range of anti-scale magnetic devices used in domestic and industrial environments that claims to effectively reduce the amount of CaCO3 precipitation in hot water pipes. However, its effectiveness has been debated until now (Alabi et al., 2015). In agriculture, different types of magnetic devices have been tested for irrigation in crop production, showing an enhancement of early growth and biomass yield, along with optimization of water use, which is of great interest in this field (Amira et al., 2010; Ferrari et al., 2015; Mahmood & Usman, 2014; Sadeghipour & Aghaei, 2013). Nonetheless, the main criticism of this technology is the lack of a plausible physiological mechanism, the absence of a standardized magnetic treatment, and the low reproducibility of the experiments.
Despite the above factors, a critical issue being studied is the use of low-quality water, or limited water supply, to reduce the depletion of the surface and underground water. In this regard, Surendran et al. (2016) found that irrigation with hard and saline water previously treated with a magnet of 180-200 mT (millitesla) led to a significant increase in length and weight of plants of cowpea and eggplant and less deterioration of crop yield. The latter was ascribed to a higher soil moisture profile in the magnetically treated plots and a different pattern of CaCO3 precipitates in the irrigation system.
Furthermore, in a study with red and yellow pepper using brackish water, the application of MTW produced an increase in the nutrient concentration in fruits, fruit yield, water use efficiency, and chlorophyll content (Marei et al., 2014). The latter authors also pointed out that decreasing the volume of irrigated water with MTW would be worthy of research. Accordingly, Ferrari et al. (2015), Ogunlela and Yusuf (2016), Yusuf and Ogunlela (2017) mention that MTW elicited a significant increase in the fresh weight of different crops even with little water or nutrient supply, suggesting that the application of MTW in conditions of limited irrigation results in positive yield effects.
The attempts to explain the mechanisms by which MTW exerts these effects in plants have fallen into two categories: the physiological and the soil-water relations. The physiological hypothesis indicates that once the plant absorbs MTW, it increases the membrane permeability and the ion transport through the cells due to the smaller cluster size of this treated water (Zúñiga et al., 2016). Increased enzymatic activity has also been cited as a possible mechanism in germinating seeds soaked in MTW (Massah et al., 2019), but its evidence in developed plants is scarce (Kunzhen et al., 1994).
On the other hand, the soil-water relation mechanism explains that the wetting pattern in soils of different textures increments when using MTW in drip irrigation systems because water molecules penetrate the soil particles’ micropores and attach to them easily, which results in preventing water from moving to the lower soil depths (Al-Ogaidi et al., 2017). The latter is attributed to the hydrogen bond, and other intermolecular forces weakened in MTW, making water molecules more easily adsorbed to soil particles. Surface tension is another water property likely involved in these mechanisms. Water has high surface tension compared with other substances of similar molecular weight and geometry allowing water to exhibit quite elevated cohesive and adhesive forces with itself and other substances.
The effects of magnetic fields on the surface tension of water have been studied, and the general trend observed is that there is a noticeable reduction in this parameter under different experimental conditions (Cai et al., 2009; Hasaani et al., 2015; Huo et al., 2011; Pang & Bo, 2008). According to Pang and Bo (2008), this phenomenon is a manifestation of the variations in the molecular structure of water influenced by magnetic fields, such as the distribution of molecules and electrons, displacements and polarization of molecules and atoms, the transition of dipole moment and vibrational states of the molecules. Surface tension is an important factor driving water transport in vascular plants (Domec, 2011), finding evidence of its role in xylem transport and embolism (Schenk et al., 2015, 2017).
The restriction of water supply in crops is a practice that optimizes water use for crop biomass accumulation. In Capsicum cultivars, it was observed that reduced irrigation could be a feasible strategy for hot pepper production where the benefit from saving water outweighs the concomitant decrease in total fresh mass of fruit (Dorji et al., 2005). For instance, Pérez- Gutiérrez et al. (2016) found that a suitable combination of different Capsicum chinense genotypes plus a 40 % water supply led to better physiological performance (measured as chlorophyll fluorescence and biomass accumulation regarding water consumption). Other experiments carried out in semi-controlled conditions showed that Tabasco pepper plants increased their fruit yield and mineral content when consistently irrigated with MTW (Ospina-Salazar et al., 2018). In Colombia, Tabasco pepper is often cultivated in areas with high evapotranspiration demand and scarce water availability, like the western inter-Andean valleys (Dagua, Vijes, Guabas in Valle del Cauca) and the northern Caribbean basin (Atlántico and Bolívar).
Hence, a combination of MTW with reduced water supply could be a proper approach to validate the applicability of this technology to a crop of great economic importance such as Tabasco pepper, testing MTW as an alternative to ameliorate losses in biomass yield due to the restricted irrigation. This work aims to evaluate the overall effect of MTW at full (no water deficit) and half (water deficit) irrigation on the physiology and biomass yield of Tabasco pepper (Capsicum frutescens L.).
Materials and methods
Location, plant material, and setup of the experiment
Two experiments were conducted in a 125 m. mesh house at Universidad del Valle experimental station in Cali, Valle del Cauca Province, Colombia. The station is located at 967 m above sea level, with a daily average temperature and relative humidity at the time of 26 °C and 65 %, respectively. The first experiment (from August to December 2016) involved 52 one-year-old Tabasco pepper plants (Capsicum frutescens L.), extant from a previous experiment, in 10 L plastic bags. The plants were cut-off at the base of the stem and then allowed to regrow for three weeks before water treatments to obtain homogeneous and vigorous plants. The soil was a clay loam topsoil previously homogenized and sieved, with a pH of 6.79 and organic matter content of 2.57 %. The second experiment was conducted from June 2017 to May 2018. Fifty-days old seedlings of Tabasco pepper were transplanted in 10 L plastic bags with a clay loam topsoil like that of the first experiment, totaling 48 bags. In both experiments, each plastic bag was connected to a drip irrigation system with emitters of 3 L/h. The water source was the municipal service company, having the same specifications for drinking or tap water. The mineral nutrients were applied to the soil in 50 % Hoagland solution diluted in the irrigation system once a month, whereas pest and disease control was achieved with chemical and mechanical methods.
Magnetic treatment of water and irrigation levels
The water was treated using a Quantum Biotek magnet (Omni Enviro Water Systems, Australia). This device is a cylinder of twelve centimeters long and one inch in internal diameter, generating a variable magnetic field from 0 to 156 mT that increases along the length and around the cylinder circumference. The north-south poles are also switched along the length and the circumference of the cylinder. The device was connected to the irrigation system before derivation to the plants. The MTW was recirculated for five minutes through the magnet at a flow rate of 10 L/minute in a 100 L-cistern. Control (CON) water was also recirculated through a bypass without any magnetic field in the same conditions. The irrigation levels were adjusted to 100 % and 50 % of field capacity (FC) according to the weight of soil in the bags and the soil water retention curve given by the texture (clay loam). It yielded four treatments according to the water type and the irrigation level: MTW at 100 % FC (MTW100), MTW at 50 % FC (MTW50), control water at 100 % FC (CON100), and control water at 50 % FC (CON50).
Physiological assessments
Leaf water potential (Ψw) was measured with a Sholander-type pressure chamber model 1005 (PMS Instrument, USA) at predawn (05:00 to 06:15 hours) and midday (11:30 to 13:15 hours) only in the first experiment. The measurements were taken in fully expanded, sun-exposed, young leaves with a petiole long enough to fit in the chamber. For relative water content (RWC), leaves of the same physiological age were carefully detached from the plants and weighed in the laboratory. Then, the leaves were immersed in distilled water and left in the fridge at 4 °C for six hours, when they were soaked and weighed again. Finally, the leaves were oven-dried for 24 hours at 65 °C and weighed again. RWC was calculated according to equation (1):
The membrane stability through electrolyte leakage (EL) was determined according to Rachmilevitch et al. (2006). Two young leaves per plant were rinsed with distilled water, immersed in 20 mL of deionized water, and shaken at 80 rpm for 24 hours on a shaker table. The conductivity of this shaken solution was measured with a conductivity meter (Jenway model 470 portable conductivity/TDS meter, United Kingdom). The leaves were then destroyed by autoclaving at 120 °C for 20 minutes, measuring the final conductivity of this solution with the processed tissues. Relative electrolyte leakage was calculated using equation (2).
Photosynthesis measurements were only taken in the first experiment. Gas exchange parameters (net photosynthesis, A; transpiration, E; stomatal conductance, gs) were measured with a CI-340 Handheld Photosynthesis System (CID Bio-Science, USA) under natural environment conditions of CO2 concentration, humidity, and light (398 ppm, 70 % and 480 µmol quanta m-2 .-1 respectively). The intake tubing was introduced in an empty plastic bottle at 1 m above the soil to avoid disturbing the intake of CO2 and the water pressure. Water use efficiency (WUE) was calculated as the ratio A/E from the previous measurements. The readings were taken between 11:00 and 15:00 hours on a sunny, clear day, on fully expanded, sun-exposed young leaves. Chlorophyll fluorescence was measured using an OS30p+ Fluorometer (Opti-Sciences Inc., USA) for dark-adapted measurements as Fv/Fm (maximum quantum efficiency of PSII photochemistry). The dark adaptation was achieved by covering the leaves for 30 minutes with suitable clips. These measurements were taken in the first three fully expanded, sun-exposed young leaves once a week in each plant.
Fruit biomass measurements
Once the plants started to produce fruits, successive harvests were gathered as the fruits ripened. In the first experiment, fruits were collected five times from October to December 2016, whereas in the second experiment, fruits were harvested seven times from the fifth until the eleventh month after transplanting (from November 2017 to May 2018). Right after harvesting, the fruits were counted and weighed for fresh weight. Dry weight was measured after drying the fruits in an oven at 70 °C until reaching a constant weight.
Experimental design and data analysis
The experiment was carried out in a completely randomized design. One plant in each plastic bag was considered as a single replicate. In the first experiment, there were thirteen replicates per treatment (52 plants in total). In the second experiment, there were twelve replicates per treatment (48 plants in total). Data were analyzed with IBM SPSS Statistics 22 (IBM Corp., Armonk, NY), first running tests of homogeneity of variances and normality (Levene and Shapiro-Wilk, respectively). As these tests did not lead us to reject the null hypothesis of homoscedasticity and normality, we carried out an ANOVA followed by post hoc comparisons of all treatments by Duncan’s multiple range test. Since fruit biomass yield is the most interesting variable at the agronomical level, a Cohen’s d index was calculated to estimate the effect size of the MTW over control at 100 % FC, 50 % FC, and the combination of both irrigation levels. This index standardizes the difference by transforming the absolute difference into standard deviation units according to the formula:
Where M1 – M2 is the difference between the group means, and S is the standard deviation of either group (Sullivan & Feinn, 2012).
Results and discussion
Physiological assessments
It was particularly evident for Ψw that the most remarkable differences occurred between the two irrigation levels (100 % and 50 % of FC) within both treatments. However, at predawn, the Ψw of MTW at 50 % FC was not statistically different from that of 100 % FC, unlike in control plants, where the gap between irrigation levels was more significant (0.14 MPa, Table 1). The same effect was observed in midday Ψw, where the distance between 100 % FC and 50 % FC was higher in control plants than in treated plants. It might indicate a buffer effect of MTW in leaf water potential, particularly at midday, where the treated plants at 50 % FC significantly differed from those with untreated water (control). However, the overall effect disappeared when combining both irrigation levels within MTW and control. On the other hand, the combination of both treatments within 100 % FC and 50 % FC showed the effect of water limitation on leaf water potential unequivocally, being the distance between both irrigation levels large and significant (Table 1), either at midday or predawn.
Table 1 Midday and predawn leaf water potential (Ψw), relative water content (RWC), and electrolyte leakage (EL) in Tabasco pepper plants, comparison of each treatment, and differences between the two irrigation levels with two water types. Data are means ± standard error of 13 plants in each treatment.
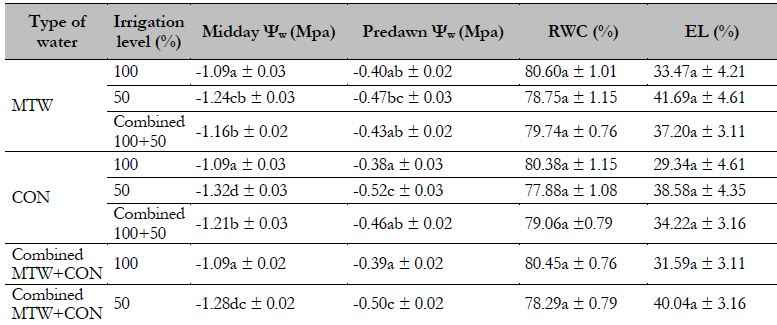
Note.Means followed by distinct letters differ significantly according to Duncan’s test.
Source: Elaborated by the author.
Although there was no significant difference regarding RWC and EL, there was a consistent pattern among plants at 100 % FC and 50 % FC. This pattern shows that plants at full irrigation always had higher RWC and lower EL, which is an indicator of better physiological status. As for RWC, it is remarkable that the difference between all plants at 100 % FC and 50 % FC was not very significant, in contrast to that of Ψw, which might indicate that RWC in leaf tissues is less sensitive to water limitation than leaf water potential. On the other hand, the lower EL of fully irrigated plants (26.7 % lower) means that the cell membrane integrity is higher than in half- irrigated plants, but the absence of significant differences is attributed to the high standard error (table 1). Similar to Ψw, there was a reduction in A, WUE, E, and gs at 50 % FC in both water types (MTW and control). Fv/Fm only decreased in MTW at 50 % FC but not in control plants (table 2).
Table 2 Gas exchange and photosynthesis parameters in Tabasco pepper plants, comparison of each treatment. Data are means ± standard error of 13 plants in each treatment.
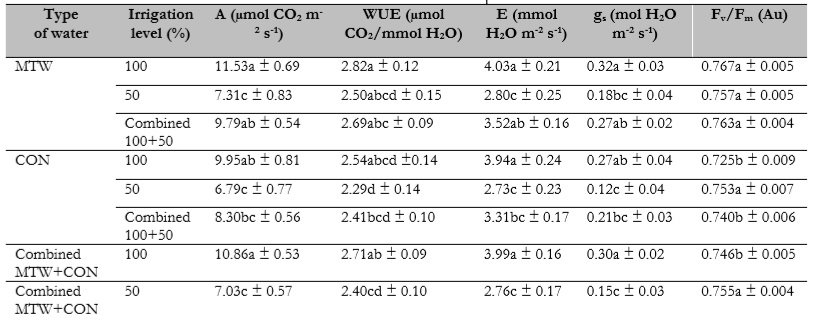
Note.Means followed by distinct letters differ significantly according to Duncan’s test.
Source: Elaborated by the authors
Besides, treated plants always exhibited higher values of these parameters at either 100 % or 50 % FC than their untreated counterparts, although they belong to the same statistical group (sharing at least one letter), except for Fv/Fm at 100 % FC. Nevertheless, it is noticeable that the values of MTW merging both irrigation levels were statistically equal to those of 100 % FC among the four gas exchange parameters tested, whereas the values of control plants did not show this statistical pattern. The latter could be evidence of the amelioration effect of MTW in the gas exchange process and the light reactions (chlorophyll fluorescence) at a low water supply.
Of particular importance is to highlight that water use efficiency (WUE), measured as the amount of CO2 assimilated per unit of H2O released, was consistently higher (but not significantly) in MTW-plants despite the apparent increase in transpiration (E). It is of great relevance at crop level management since MTW could be a strategy for saving water without hindering biomass accumulation. Quantum efficiency of photosystem II, measured as the ratio of variable fluorescence (Fv) between maximum fluorescence (Fm) in dark-adapted leaves, showed a higher and significant value at MTW combining both irrigation levels, which is indicative of less water stress. Comparing both irrigation levels regardless of the type of water yielded significant decreases among all the gas exchange parameters at 50 % FC, except for chlorophyll fluorescence (table 2).
Biomass accumulation
The fruit set in Tabasco pepper plants is shown in table 3. The number of fruits, fresh and dry weight per plant were consistently higher in MTW-plants in both experiments, either at 100 % or 50 % of FC.
Table 3 Fresh weight (FW), dry weight (DW), and the number of fruits in Tabasco pepper plants. Data are means ± standard error of 13 plants per treatment in the first experiment (52 in total) and 12 plants per treatment in the second experiment (48 in total).
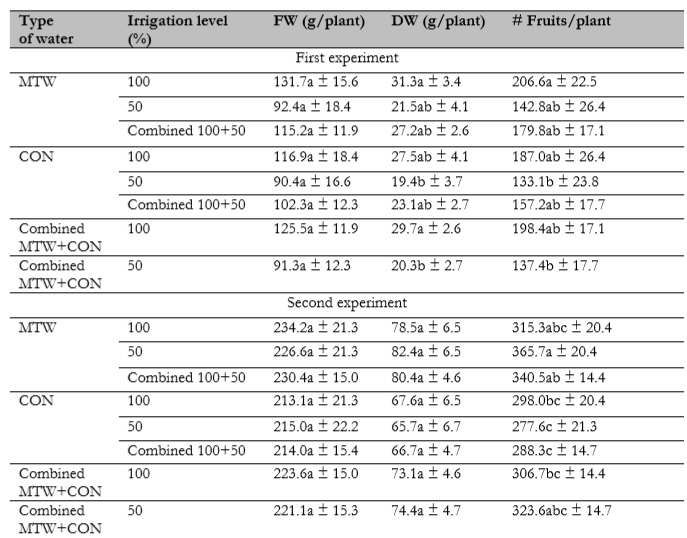
Note.Means followed by distinct letters differ significantly according to Duncan’s test.
Source: Elaborated by the authors
Unexpectedly, the MTW group of the second experiment reported a greater DW and number of fruits in the plants at 50 % FC than in the 100 % FC plants (although not significant). Similarly, the difference between fully irrigated and half irrigated plants in the control group was not as significant as in the first experiment regarding FW and DW. The latter explains why the values of FW, DW, and the number of fruits were almost identical when comparing all 100 % FC plants with 50 % FC plants. The lack of statistical significance between both MTW and control is attributed to the high standard error inherent to the measurements plant by plant since some of them easily outdo the mean yield, whereas others do not. Despite the above mentioned, the percentage increase in FW, DW, and the number of fruits in MTW-plants over control at 100 % FC was 11.3 %, 15.0 %, and 8.1 % on average, respectively (accounting for both experiments, figure 1).
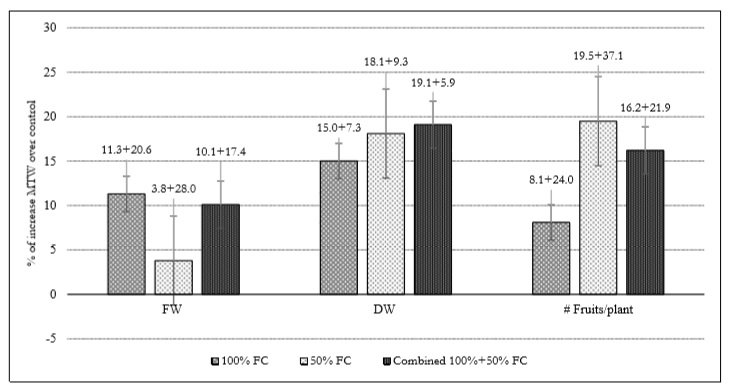
Source: Elaborated by the authors
Figure 1 Percentage increase in fresh weight (FW), dry weight (DW), and the number of fruits in MTW-plants over control plants. Data are the average of the first and second experiments (plus the standard error of the mean difference).
There was an increase of 10.1 %, 19.1 %, and 16.2 % in these three parameters in the treated plants considering the combination of the two irrigation levels. It is noteworthy that, at 50 % FC, the gain in fresh weight was only 3.8 % (not unexpected because of the low water regime imposed), but the gain in dry weight and number of fruits was even more significant than at 100 % FC. It indicates that the plants produced more but smaller fruits, with less water and drier biomass. Cohen’s d index was calculated for each irrigation level in treated and untreated plants to correctly measure the effect size of MTW on fruit yield (table 4).
Both experiments tested a comparison between MTW at 100 % FC and 50 % FC with its respective control counterparts. This index standardizes the difference by transforming the absolute difference (shown in Figure 3 as percentages) into standard deviation units and classifies effect sizes as small (d = 0.2), medium (d = 0.5), and large (d > 0.8) (Sullivan & Fein, 2012).
Table 4 Effect sizes of MTW over control in fruit yield parameters according to Cohen’s d
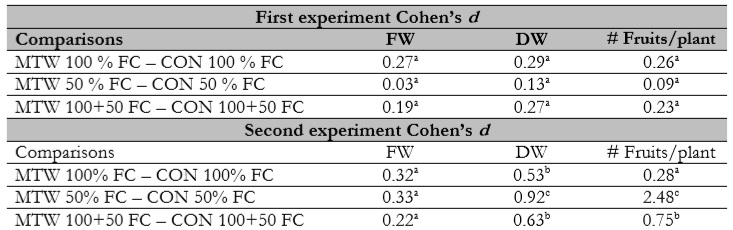
Note.a small; b medium; c large
Source: Elaborated by the authors
Based on these premises, there were large effect sizes in the second experiment, specifically in the number of fruits per plant and dry weight (MTW-50 % FC over CON-50 % FC), and medium effect sizes in dry weight (MTW-100 % FC over CON-100 % FC; MTW 100+50 FC over CON 100+50 FC) and fruits/plant (MTW 100+50 FC over CON 100+50 FC). In general, the smaller effect sizes were detected in fresh weight among both experiments but increased to the small-medium range in dry weight and fruits/plant. Another pattern that emerged from this analysis is that the effect sizes of MTW-50 % FC over its correspondent control were small among the three fruit yield parameters in the first experiment, unlike the second experiment.
In this study, the main question was if MTW can enhance crop yield in Tabasco pepper while reducing water application to a half of field capacity; the results show that it does, but marginally from a statistical point of view. From an effect-size point of view, the outcomes of this work support this hypothesis, and the physiological performance of the tested plants points in that direction.
Regarding water transport in plants, an increase in E and Ψw was observed in MTW-plants, merging both irrigation levels. Even though greater transpiration implicates more water consumption (thus lower water use efficiency), here is essential to point out two things: the first, MTW has been proved to have a higher evaporation rate (Ben-Amor et al., 2017; Guo et al., 2012), and second, that transpiration is the ultimate pulling force of the water column in the xylem, taking place in the substomatal chamber of the leaves. Since this process is closely related to stomatal conductance, a more stomatal opening is needed to release H2O, leading to a higher CO2 intake. The increasing pattern in transpiration and leaf water potential (less negative Ψw) can be a manifestation of the lower molecular energy of MTW (Cai et al., 2009), which leads to more accessible water transport. It is not irrelevant to note that the magnetic treatment reduced the distance in Ψw between fully and half-irrigated plants, particularly at predawn. Since predawn Ψw is generally considered the best representation of water status at the crop level (Améglio et al., 1999), our results indicate that plants grown under MTW develop better conditioning in the soil-plant-atmosphere relation. The latter explanation is consistent with the behavior of MTW in soil experiments since several authors have reported that the wetted radius increases in surface or depth according to the soil texture (Al-Ogaidi et al., 2017) and that average soil moisture at different depths is higher compared to water with no magnetic treatment (Khoshravesh et al., 2011). Although soil humidity parameters were not measured in this experiment, it is likely that the physiological and yield responses observed in the plants under MTW were a consequence of the particular properties of this water in its interaction with the soil particles.
Regarding gs, WUE, and A, there was a significant decline at 50 % FC in both water types. However, the fact that plants with MTW showed the highest values of these three parameters supports that these plants produced more fruit yield even at water deficit. This agrees with Ferrari et al. (2015) and Al-Khazan et al. (2011), whose results suggest that irrigation with MTW, even at low moisture regimes, optimizes the plants’ ability to manage water sustain biomass. Our results support these findings based on a stomatal effect, which explains this ability by augmenting stomatal conductance (gs), which, while leading to higher transpiration, also increases net photosynthesis (A), accounting for the greater WUE found in the MTW-plants. Here is relevant to mention that gs is also sensitive to Ψw (not only to abscisic acid, ABA), so less negative water potentials (as those recorded in this work) could also be related to a bigger stomatal opening (Bunce, 1999; Ripullone et al., 2007). Therefore, the augmentation in CO2 assimilation under MTW leads to an increase in the dry biomass and number of fruits, an outcome found in a previous report (Ospina-Salazar et al., 2018).
Despite a consistent rise in A and fruit yield, significance was lacking in every possible comparison between MTW and control without focusing on the magnitude of the difference between them. According to Cohen’s d index calculated for fruit yield, there are noticeable effect sizes in dry weight and fruits/plant, and less critical effect sizes in fresh weight, but not so small as trivial. In this regard, Phimchan et al. (2012) reported in drought-stressed plants of nine cultivars of C. chinense and C. annuum an average reduction in dry fruit biomass and the number of fruits per plant of 30 %. As seen in this work, the use of MTW increased the dry weight and the number of fruits per plant over control plants substantially in one of the two experiments. The low water supply at 50 % FC produced significant changes in leaf tissue status; this is EL and RWC. EL is an indicator of cell membrane stability, measured as the concentration of electrolytes (ions) released from the cells to the water. Stressing conditions such as drought or salinity will usually impair the toughness of the cell membrane, which could result in higher values of EL in leaves and other tissues (Valentovič et al., 2006).
Moreover, leaf relative water content (RWC), which measures the extent of leaf tissue hydration, is rapidly decreased by low soil water availability in Capsicum species (Phimchan et al., 2012). It was evident that RWC was not significantly affected by the MTW or the low irrigation level. The latter outcome indicates that the magnetic treatment does not affect leaf tissue hydration in Tabasco pepper and that Ψw is more sensitive to water stress than RWC. The latter was also found by Phimchan et al. (2012), whose results show clearly that the decrease in leaf water potential in drought-stressed plants was four-fold to seven-fold, whereas relative water content never fell more than two-fold. As for EL, it is worth noting that MTW increased it and the lowest level of irrigation (no matter the type of water), indicating a decline in the cell membrane stability, an expected outcome in drought conditions, but a particular effect of MTW. It could be attributed to changes in ion selectivity and integrity function of Ca2+ in the membranes, a phenomenon in water solutions after magnetic treatment (Fathi et al., 2006). At any rate, the lower membrane stability in plants subjected to MTW seems to have not affected the general physiological performance, such as gas exchange and fruit yield. The possible physiological mechanism of MTW is likely related to facilitated water transport via more transpiration, which triggers higher stomatal conductance and CO2 assimilation. The soil-water mechanism proposes that increased wetting pattern in the soil delays plant adjustments of their photosynthetic apparatus to a water stress regime. The latter mechanism is consistent with the reduction of surface tension observed in MTW previously (Ospina-Salazar et al., 2021)
Conclusions
The evidence collected in this work suggested that MTW has a positive effect on the fruit yield of Tabasco pepper. The physiological parameters tested follow this behavior, particularly net photosynthesis and quantum yield. Leaf water potential and leaf tissue status (such as relative water content and electrolyte leakage) did not show significant modifications, although the general pattern was that plants under MTW had fewer manifestations of water stress in these parameters. This was observed as significant in leaf water potential of MTW-plants at 50 % FC compared to control plants. Combining complete and limited irrigation in Tabasco pepper with MTW could be a convenient practice to sustain crop yield with low use of water, which may increase the water use efficiency measured as dry biomass per volume of water applied.