Serviços Personalizados
Journal
Artigo
Indicadores
-
Citado por SciELO
-
Acessos
Links relacionados
-
Citado por Google
-
Similares em SciELO
-
Similares em Google
Compartilhar
Boletín de Investigaciones Marinas y Costeras - INVEMAR
versão impressa ISSN 0122-9761
Bol. Invest. Mar. Cost. vol.46 no.2 Santa Marta jul./dez. 2017
https://doi.org/10.25268/bimc.invemar.2017.46.2.725
Research Articles
Epiphytic dinoflagellates of Thalassia testudinum in two coastal systems of the Colombian Caribbean
1 Universidad Nacional de Colombia sede Caribe - Cecimar e Instituto de Investigaciones Marinas y Costeras - Invemar . Calle 25 No. 2-55, Playa Salguero. Rodadero, Santa Marta, Colombia. natalia.arbelaez@invemar.org.co
2 Universidad Nacional de Colombia sede Bogotá, Carrera 45 No 26-85, Bogotá D.C. Colombia. jemancerap@unal.edu.co
3 Instituto Español de Oceanografía (IEO) , Centro Oceanográfico de Vigo , Subida a Radio Faro 50, 36390 Vigo, España. beatriz.reguera@vi.ieo.es
Studies on potentially toxic epibenthic dinoflagellates have been increased in the last years due to growing number of harmful events attributed to some of these microalgae. These events represent a risk to human health, as well as to diverse economic activities. With the aim of confirming the presence of these dinoflagellates in Tayrona National Natural Park, Colombian Caribbean, monthly samples of the seagrass Thalassia testudinum were collected between January 2014 and December 2015 in two coastal systems (Bay and Lagoon) located in Chengue Bay (11°20´ N y 74°07´W). Grass leaves were manually collected, placed in plastic bags with seawater and transferred to laboratory for detachment of epibenthic microalgae by vigorous shaking. To identify the species, three types of microscopy were used (optical, inverted with epifluorescence and scanning electron microscopy). Fourteen species of potentially toxic epiphytic dinoflagellates, distributed in four genera (Gambierdiscus, Coolia, Ostreopsis and Prorocentrum) were identified. Prorocentrum was the most representative genus. Ostreopsis cf. ovata, P. hoffmannianum and P. lima were the most frequent species in the Bay, whereas Prorocentrum sp. 1 and P. rhathymum were the most frequent in the Lagoon. This study depicts the main features of the observed species, considering that precise identification at species level is required for any ecological study that seeks to provide elements for risk management against the toxic or harmful effects caused by epibenthic dinoflagellates.
Keywords: Toxic dinoflagelates; Colombian Caribbean; Benthic HABs; Seagrass
Los estudios sobre dinoflagelados epibentónicos potencialmente tóxicos han incrementado en los últimos años debido al aumento en el número de eventos perjudiciales atribuidos a algunas de estas microalgas. Tales eventos constituyen un riesgo para la salud humana y para diversas actividades económicas. Con el objetivo de confirmar la presencia de estos dinoflagelados en el Parque Nacional Natural Tayrona (PNNT), Caribe colombiano, se recolectaron mensualmente muestras del pasto marino Thalassia testudinum entre enero de 2014 y diciembre de 2015 en dos sistemas costeros (bahía y laguna) ubicados en la Bahía de Chengue (11 ° 20' N y 74 ° 07' W). Las hojas del pasto marino se tomaron manualmente, se depositaron en bolsas plásticas y se trasladaron al laboratorio para el desprendimiento de los dinoflagelados epibentónicos, mediante agitación vigorosa. Para identificar las especies observadas, se utilizaron tres tipos de microscopía (óptica, invertida con epifluorescencia y microscopía electrónica de barrido). Se observaron 14 especies de dinoflagelados epifitos potencialmente tóxicos, distribuidos en cuatro géneros (Gambierdiscus, Coolia, Ostreopsis y Prorocentrum). Prorocentrum fue el género más representativo, siendo Ostreopsis cf. ovata, P. hoffmannianum y P. lima las especies más frecuentes en la bahía, mientras que en la laguna fueron Prorocentrum sp. 1 y P. rhathymum. Este estudio describe las características principales de las especies observadas, considerando que la identificación precisa a nivel de especie es requerida para cualquier estudio ecológico que busque proporcionar elementos para la gestión de riesgos contra los efectos tóxicos o perjudiciales causados por dinoflagelados epibentónicos.
Palabras clave: Dinoflagelados tóxicos; Caribe colombiano; FANs bentónicas; Pastos marinos
INTRODUCTION
In recent decades, an apparent increase has occurred in human intoxication and death from organisms associated with the intensification of microalgal proliferation worldwide (Hallegraeff et al., 2004; Mancera-Pineda et al., 2009). The main agents of these episodes are planktonic and epibenthic dinoflagellates (associated with marine phanerogams, macroalgae, sediments and other substrates) that produce potent toxins (Hallegraeff et al., 2004). Unlike phytoplankton cell proliferation, which can cause water coloration (red tides), even high concentrations of epibenthic dinoflagellates may go unnoticed. However, their toxins accumulate in various marine organisms (fish or mollusks), generating public health risk if consumed by humans (Alvarez, 1997, 1999; Gaitán, 2007).
Among the major toxin-producing epibenthic dinoflagellates are species belonging to the genera Ostreopsis, which is a producer of ovatoxins, palytoxin and their analogs (Lenoir et al., 2004; Brissard et al., 2014) and is responsible for dermal and airway irritation (Gallitelli et al., 2005; Tubaro et al., 2011; Del Favero et al., 2012; Ciminiello et al., 2014), Coolia, which is producer of cooliatoxin (Holmes et al., 1995; Rhodes et al., 2000), Prorocentrum, which causes diarrhetic shellfish poisoning (DSP), and Gambierdiscus. The latter genus has some of the highest toxic potential because some species are able to produce gambiertoxins and maitotoxins, which accumulate in reef fish (e.g., Sphyraena barracuda) and cause the toxic syndrome called "ciguatera" (Alvarez, 1997, 1999, Gaitán, 2007). This disease generates recurrent gastrointestinal, neurological and cardiac problems (Arcila-Herrera et al., 2001, Vila et al., 2001, Faust, 2009, Mancera-Pineda et al., 2009).
The study of epibenthic dinoflagellates is important because although not all species of this group are producers of toxins, the species are frequently misidentified due to their wide morphological variability. The accurate and detailed identification of species is essential, which is not an easy task using low resolution optical methods. The present study describes the main species of potentially toxic dinoflagellates present in the seagrass beds of Thalassia testudinum in two coastal systems of the Colombian Caribbean as a contribution to the knowledge of these organisms in the region.
STUDY AREA
Between January 2014 and December 2015, samples were collected monthly from two coastal systems in Chengue Bay, Tayrona National Natural Park (PNNT) (11° 20' N and 74° 08' W), which is located 14 km northeast of Santa Marta city in the Colombian Caribbean. The first sampling point was established in the interior of the bay (11° 19'07" N and 74° 07'42" W) on the abundant T. testudinum bed, and the second was established in the central part of a lagoon located in the southern part of the bay (11° 19'01" N and 74° 07'42" W) (Figure 1). The lagoon, which is permanently connected with the bay by a small channel, is surrounded by a wide margin of mangrove swamp and has some patches of macrophytes in its interior (Álvarez-León et al., 1995; Rodríguez-Ramírez et al., 2004).
MATERIALS AND METHODS
At each station, leaves of the phanerogam Thalassia testudinum (80 to 100 g) were removed manually at a depth of less than 1 m and over an area of less than 10 m2. The leaves were pulled gently to prevent the release of the attached organisms and placed in sealed bags (Ziploc®). Care was taken to not allow large amounts of surrounding sea water into the bags. Once in the laboratory, pre-filtered sea water was added to the bags and vigorously shaked for two minutes to remove the dinoflagellates attached to the phanerogam. Then, the leaves were extracted, and all the water present was filtered through a 200-μm sieve to remove large residues. Each sample was placed in a 1000-ml beaker and refiltered through a 20-μm sieve. The material contained in the last sieve (200 to 20 μm fraction) was washed with filtered sea water with a wash bottle and stored in a labeled plastic bottle to which formaldehyde was added at a final concentration of 4% to fix the sample (Reguera et al., 2011). The identification and measurement of dinoflagellates were conducted using a Carl Zeiss Primo Star light microscope with an AxioCam ERc 5s camera and the AxioVision 4.8.2 image analysis program. The cells were mounted on slides, and a drop of hypochlorite was applied to clean and separate their thecal plates by exerting some pressure on the coverslip. The epifluorescence images were obtained with a Nikon Eclipse TS100 inverted microscope after applying a drop of calcofluor to dye the plates (Fritz and Trimer, 1985), and the samples were observed under a UV light filter (Reguera et al., 2011).
Micromorphological analysis of the cells was performed using the Jeol JSM 6360LV scanning electron microscope (SEM). To prepare the samples, three to five drops of this concentrate were deposited onto a 5-μm Whatman polycarbonate filter. Salt was removed by successive washes with filtered sea water diluted with distilled water in different concentrations (10, 20, 30, 50, 80, 90 and 100%) through the filter with an interval of 10 min between washes. The cells were dehydrated using diluted ethanol to reach the maximum concentration (5, 15, 30, 50, 70 and 95%) with 10 min pauses between successive concentrations. The filters with the organisms were mounted on small metal aluminum specimen holders that were placed in an oven at 50 °C for 24 h to remove the humidity. Finally, the samples were metallized with gold- palladium in a Sputtering Polaron E5000 metallizer for 5 min under an argon gas atmosphere and a metallization stream of 20 mÅ. The gold-palladium layer did not exceed 250 Å.
The documents of Fukuyo (1981), Besada et al. (1982), Faust et al. (1999), Penna et al. (2005), Mohammad- Noor et al. (2007), Litaker et al. (2009), Hoppenrath et al. (2014) and Almazán-Becerril et al. (2015) were used to confirm the species identities. Following the recommendations of Fraga et al. (2011), the modified Kofoid tabulation system described by Besada et al. (1982) was used to name the plates of genera Coolia, Gambierdiscus and Ostreopsis using the thecal formula Po, 4’, 6’’, 5’’’, 2’’’’ in the three cases. This system is based on homologous characteristics and represents the affinities with other genera by considering the cellular fission and the superposition of some of the plates. For genus Prorocentrum, the terms suggested by Hoppenrath et al. (2013) were used to refer to the cellular orientation and the nomenclature of its morphological characteristics.
It must be noted that the toxicity of the organisms observed in the present study was not determined. However, most of the described species were recorded to produce toxins in other areas that affected several organisms, including humans (Yasumoto et al., 1987; Lee et al., 1989; Holmes et al., 1991, 1995; Morton and Bomber, 1994; Rhodes et al., 2000; Delgado, 2005; Delgado et al., 2005; An et al., 2010; Tichadou et al., 2010, Ciminiello et al., 2011, 2014; Tubaro et al., 2011; Gorbi et al., 2012; Nascimento et al., 2012; Holland et al., 2013; Brissard et al., 2014; Lewis et al., 2016).
RESULTS AND DISCUSSION
SPECIES DESCRIPTION
A total of 14 species of potentially toxic dinoflagellates associated with the leaves of T. testudinum were observed; these species belonged to two orders, three families and four genera. Among the genera present, Prorocentrum was the most representative during sampling, with a contribution of 50% of the species, which coincided with previous studies in other parts of the Caribbean (Valerio and Díaz, 2008; Almazán-Becerril et al., 2015), followed by Ostreopsis with 21%. The numbers of species were similar between the two systems, with a difference of two more species in the bay. However, these differences were more evident in terms of composition. For instance, Coolia cf. malayensis, Coolia sp., Ostreopsis cf. lenticularis and Prorocentrum emarginatum were only observed in the bay, whereas Gambierdiscus sp. and Prorocentrum sp. 2 were found only in the lagoon (Table 1). In the bay, Prorocentrum lima, P. hoffmannianum and Ostreopsis cf. ovata were considered frequent species based on their appearances in all samples collected during both years, whereas Coolia sp., Gambierdiscus cf. caribaeus, Prorocentrum rhathymum and Prorocentrum sp. 1 were observed occasionally. In contrast, Prorocentrum sp. 1 was the most frequent species (79.17%) in the lagoon, followed by P. rhathymum (70.83%), whereas Gambierdiscus sp. And P. cf. concavum were found sporadically (Table 1). The species found are described below.
Gambierdiscus cf. caribaeus Vandersea, Litaker, Faust, Kibler, Holland and Tester, 2009.
Description: Large cell (dorsoventral length: 82.7 ± 4.3 μm; transdiameter: 81.2 ± 6.7 μm; n = 4). The cell has a semicircular shape in both the apical and antapical views with a noticeable sulcus (Figure 2A, B and F). The U-shaped sulcus in the antapical view is deep and narrow (Figure 2B). The body is slightly compressed in the anteroposterior direction (Figure 2E). The surface is dense with small pores (Figure 2G). Plate 2’ is rectangular (length: 47 μm, width: 20-25 μm, Figure 2C), and plate 2’’’’ is pentagonal and wide (length: 50 μm, width: 37 μm, Figure 2B and D). Based on the observed structures, especially the shapes of plates 2’ and 2’’’’, and the descriptions by Litaker et al. (2009), Jeong et al. (2012) and Hoppenrath et al. (2014), the characteristics of the species coincide with those of G. caribaeus, although the cells of the present study were somewhat larger than those described by Litaker et al. (2009).
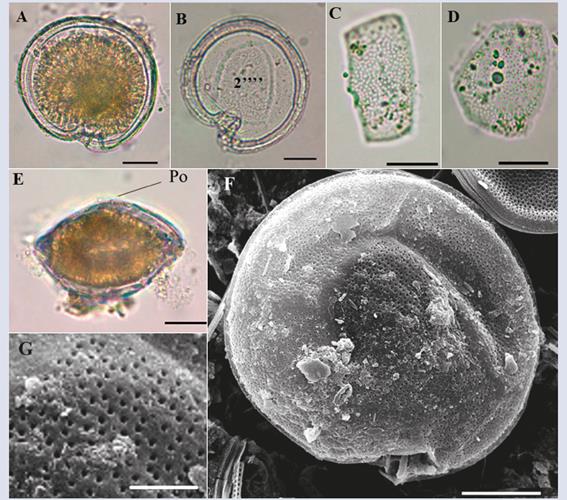
Figure 2 Micrographs of Gambierdiscus sp1 with optical microscope (A-E) and scanning electron microscope (SEM) (F-G). A-B. Cell in antapical view. C. Second apical plate (2’). D. Second antapical plate (2’’’’). E. lateral view. F. Antapical view in SEM. G. Detail of the pores that cover the cell. Po: apical pore. Scale bar (A-F) = 20 μm, (G) = 5 μm.
Habitat: Usually circumtropical, commonly benthic, associated with various substrates including macroalgae, seagrass, sand and dead coral in protected areas of the waves and away from continental water inputs (Faust, 1995; Hallegraeff et al., 2004; Tester et al., 2014). In case of disturbances, the organisms can swim by moving short distances (Hallegraeff et al., 2004).
Toxicity: Some species of the Gambierdiscus genus are the main producers of gambiertoxin and maitotoxin, which are toxins that cause ciguatera (Litaker et al., 2010, Parsons et al., 2012, Fraga et al., 2016). The toxicity of this species has not been confirmed in Chengue; however, several cases of ciguatera were reported previously in the Colombian Caribbean, particularly in the departments of Magdalena, Bolívar and San Andres Island (Álvarez, 1997, 1999; Gaitán, 2007; Celis and Mancera, 2015).
Gambierdiscus sp.
Description: Large cell (dorsoventral length: 76 μm; transdiameter: 70 μm; n = 1) that is compressed in the anteroposterior direction (Figure 3F). In apical view, the shape is more oblong than Gambierdiscus cf. Caribaeus, and the dorsal end of the cell is slightly acute (Figure 3B). A deep and wide U-shaped sulcus is evident in the antapical view (Figure 3B and D). The plate surface is covered with small pores (Figure 3H). The apical pore plate (Po) is displaced towards the ventral portion (sulcus). Plate 2’ is asymmetrical in the shape of an axe, and plate 2’’’’ is pentagonal, similar to the description by Litaker et al. (2009) and Hoppenrath et al. (2014) for G. polynesiensis. However, in the description of the authors, the dorsal end of the cell is not acute, and the dorsoventral length is shorter than the length observed in the present study. Additionally, there is no evidence of the presence of this species in the Caribbean.
Coolia cf. malayensis Leaw, P.-T. Lim and Usup, 2001.
Description: Small form (dorsoventral length: 29.9 ± 2.6 μm; transdiameter: 28.0 ± 2.4 μm; n = 18) that is rounded and slightly compressed in the anteroposterior direction with a lenticular shape (Figure 4D). In the lateral view, the epitheca shifts towards the dorsal portion, and the hypotheca is closer to the ventral area (Figure 4D) similar to the observations of Gómez et al. (2016). The apical pore plate (Po) is visible under a light microscope and is located between plates 2’ and 4’ in the upper left apical position of the cell, with an approximate size of 6.7 μm (Figure 4A). The Po size is larger than the one reported by Leaw et al. (2010) and Karafas et al. (2015) (5.0 and 5.9 μm, respectively). Plate 4’ is oblong with a hexagonal shape and is located towards the center of the cell; its right edge makes contact with plate 5’’, which tends to be the largest of the epitheca (Figure 4A and G); this pattern is similar to the pattern observed in genus Ostreopsis. Plate 3’ is pentagonal and is located between plates 4’ and 3’’. Plate 3’’’ is rectangular, centered and wide and occupies much of the hypotheca (Figure 4C and H). The surfaces of the plates are covered with small pores (Figure 4G and H). The sulcus is narrow, with two membranes projecting out on each side (Figure 4B and C). The species resembles C. palmyrensis and C. monotis based on the shape and arrangement of plates 4’, 5’’ and 3’’’. However, C. palmyrensis is usually smaller in size, and its thecal plates have a lower pore density (Karafas et al., 2015). For C. monotis, the cells have a deep sulcus expanding to occupy almost a quarter of the cell, whereas in the organism observed here, the sulcus is short with an opening towards the base (Figure 4H). Coinciding with Leaw et al. (2010), plate 3’’’ is the largest of the hypotheca in C. malayensis, whereas in C. monotis plates 3’’’ and 4’’’ have similar sizes. However, other authors have observed similarity in plate sizes in both species (Jeong et al., 2012; Gómez et al., 2016).
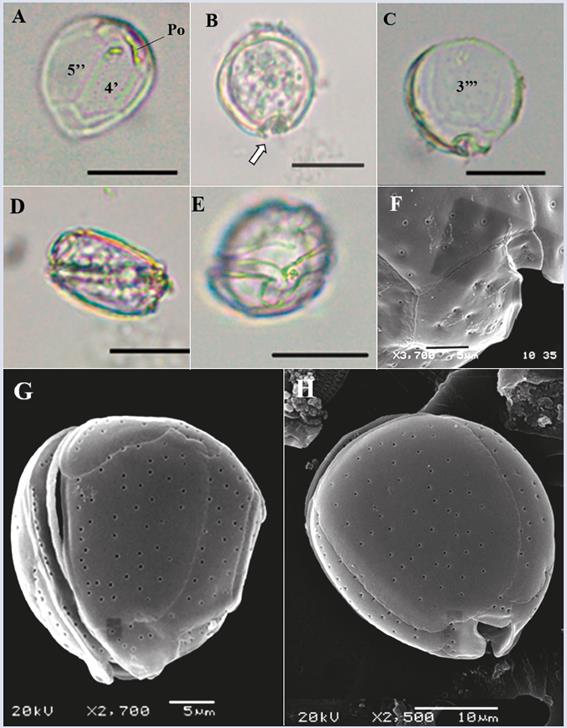
Figure 4 Micrographs of Coolia cf. malayensis under optical microscope (A-E) and SEM (F-H). A. Apical view. B-C. Antapical view (arrow indicates one of the sulcal membranes). D. Lateral view. E-F. Ventral view and sulcus details. G. Apical view In SEM. H. Antapical view. Po: Apical pore. Scale bar (A-E) = 20 μm, (F-G) = 5 μm, (H) = 10 μm.
Habitat: Marine genus, subtropical and temperate waters. Coolia cf. malayensis has been associated with macroalgae, coral fragments and sand (Leaw et al., 2010). The most studied species (C. monotis) has been observed in planktonic samples, brackish habitats, coastal lagoons and mangrove areas in shallow waters (Faust, 1992; Steidinger and Tangen, 1996); however, its distribution is often limited to temperate zones.
Toxicity: Not confirmed in the study area; however, some species of the genus are potentially toxic. A few strains produce cooliatoxin, which is a potent neurotoxin that may lead to respiratory difficulties, and blockage of some nerves essential for the functioning of the nervous system has been confirmed in some mammals (Holmes et al., 1995; Rhodes et al., 2000; Fraga et al, 2008). To date, the negative effect of this toxin has not been reported in humans.
Coolia sp.
Description: The cell is rounded and globular, and the epitheca is slightly compressed towards one end. Dimensions of single organism observed were as follows: dorsoventral length 38.89 μm and transdiameter 40.1 μm. The sulcus is even narrower than in Coolia cf. malayensis, with the presence of two small membranes projecting out on each side (Figure 5A). Unlike Coolia cf. malayensis, in the lateral view the epitheca and the hypotheca do not shift towards the ends (Figure 5B). We could not provide a more detailed description of the plates because only one cell was found in the samples. The specimen has an affinity with C. tropicalis and C. canariensis, mainly due to its size, which is larger than the other species of this genus and coincides with the sizes described by Fraga et al. (2008), Mohammad-Noo et al. (2013) and Karafas et al. (2015).
Ostreopsis cf. ovata Fukuyo, 1981.
Description: The cells are oval, elongated and acute at their ventral ends, with a tear shape (Figure 6A- C). The cells are the smallest form of the genus among those observed in the samples, with a dorsoventral length of 66.2 ± 3.5 μm and a transdiameter of 39.9 ± 3.3 μm (n = 35). The anteroposterior axis is approximately equal to the width of the cell. The apical pore plate (Po) is narrow, with a length of approximately 9.7 μm, and is noticeably shifted dorso-laterally to the left of the apical zone, which increases the difficulty of observing plate 2’ (Figure 6B and D). The sutures between the plates are soft and poorly visible. The shape is similar to O. ovata; however, according to the descriptions by Penna et al. (2005), the specimen differs from O. ovata in the shapes of plates 4’, 3’ and 2’’’’, which are similar to the plates observed in O. heptagona except for the suture indicated with the arrow in Figure 6D. In O. heptagona, the suture makes direct contact with plate 4’, whereas in the observed species, the suture makes contact with plate 3’. Both the sizes of the cells observed in the present study and the lengths of the Po are greater than those reported by Faust et al. (1996) and Faust (1999) for O. ovata. The morphological variability of this species is quite broad, and its taxonomic classification needs to be validated using the information available in genetic databases (Penna et al., 2005).
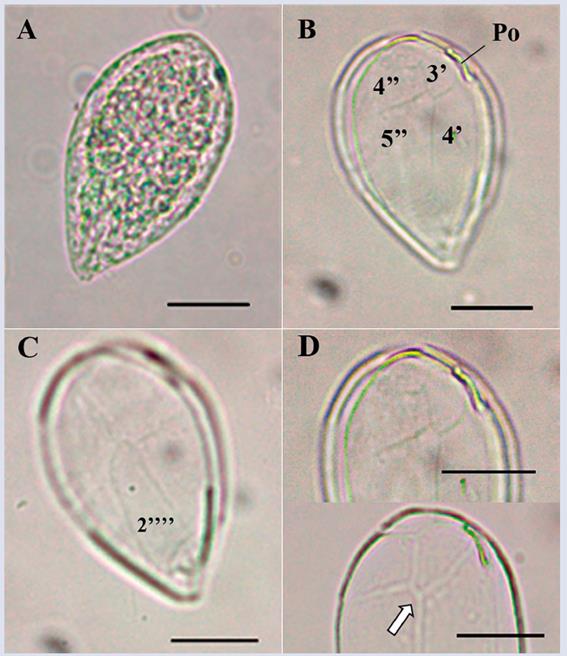
Figure 6 Micrographs of Ostreopsis cf. ovata observed under optical microscope. A. Apical view. B. Detail of the apical plates. C. Antapical view and plates details. D. Details of the plates sutures and of the apical pore complex (arrow indicates the suture between plates 3’ y 5’’ that separate 4’ y 4’’). Po: apical pore. Scale bar = 20 μm.
Habitat: Ostreopsis ovata is a marine benthic species that may be attached to macrophytes and other substrates. This species is common in tropical, subtropical and temperate zones. However, O. ovata is often more frequent in warm waters (Parsons et al., 2012). Ostreopsis sp. have occasionally been observed in planktonic samples.
Toxicity: The species has been recorded as a producer of various toxins, including ostreotoxins and palytoxins (Granéli et al., 2011), which cause gastrointestinal, respiratory and dermatological problems in humans (Parsons et al., 2012). However, its toxicity has not been confirmed in the study area.
Ostreopsis sp.
Description: The cells are ovoid and somewhat pointed towards the ventral portion and wide towards the dorsal end in the apical view. Dimensions: dorsoventral length: 84.8 ± 5.7 μm; transdiameter: 64.6 ± 5.5 μm (n = 37). Compressed dorso-ventrally, the thecal plates exhibit undulations that are evident in lateral view (Figure 7E) similar to the description by Fukuyo (1981) for O. siamensis. The thecal plates are covered by a single type of pores, which are located somewhat distant from each other and form a contiguous line in the outer margin of the precingular plates (Figure 7F). The apical pore plate (Po) is narrow and elongated (19.7 μm) with a curvature parallel to the upper left edge of the cell (Figure 7B, C and F). Considering the size of the cells observed, the length of the Po and the shape and arrangement of the thecal plates, the species could be O. labens (Faust et al., 1996; Hoppenrath et al., 2014); however, according to the descriptions reported by some authors, the species does not present undulations in the plates. Due to their variability in size and shape, the cells observed may be part of a complex composed of more than one species. However, the taxonomic description of this genus is not very clear, and most of the characteristics overlap between species, making their differentiation very complex. Penna et al. (2005) indicated that high morphological variability could be observed between cells of the same species, and Almazán- Becerril et al. (2015) mentioned that the taxonomy of this genus was so ambiguous that differentiation between species was impossible.
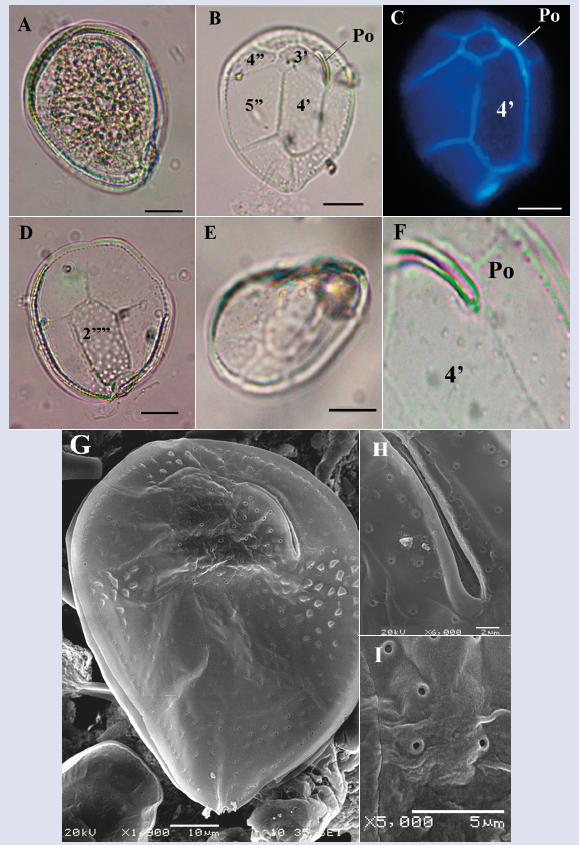
Figure 7 Micrographs of Ostreopsis sp. under optical microscope (A-F) and SEM (G-I). A. Cell in antapical view. B. Detail of the apical plates. C. Apicales plates stained with calcofluor and view by epifluorescence. D-E. Internal view of the antapical plates. F. Apical pore (Po) and pores. G. Apical view in SEM. H. Detail of the apical poro plate (Po). I. Pores that cover the cell. Scale bar (A-E) = 20 μm, (G) = 10 μm), (H) = 2 μm, (I) = 5 μm.
Ostreopsis cf. lenticularis Fukuyo 1981.
Description: The cell is rounded in the apical view (Figure 8A-C), lenticular and slightly pointed towards the sulcus. The cell is antero-posteriorly compressed and has larger size than the other species of this genus observed in the samples. Dorsal-ventral length: 102.1 ± 7.0 μm; transdiameter: 83.8 ± 6.4 μm (n = 24). The thecal plates have two types of pores (small and more abundant and thicker); both pore types are visible under a light microscope (Figure 8D). The Po is narrow and long (Figure 8A), with an approximate length of 22 μm. This specimen has the same pattern of plates as Ostreopsis sp.; however, apart from the size and presence of the two types of pores, O. cf. lenticularis is rounded and lacks thecal undulations, which coincides with the description by Hallegraeff et al. (2004). Faust (1996) indicated that the presence of both types of pores occurred in O. siamensis, although Fukuyo (1981) and Parsons et al. (2012) agreed that this characteristic was typical of O. lenticularis. Additionally, the cell size and the length of the Po coincide with some of the characteristics described by Faust (1999) for O. marinus; however, the author indicated that the thecal surface of this species had small pores and not two types of pores, as was observed in the cells from Chengue.
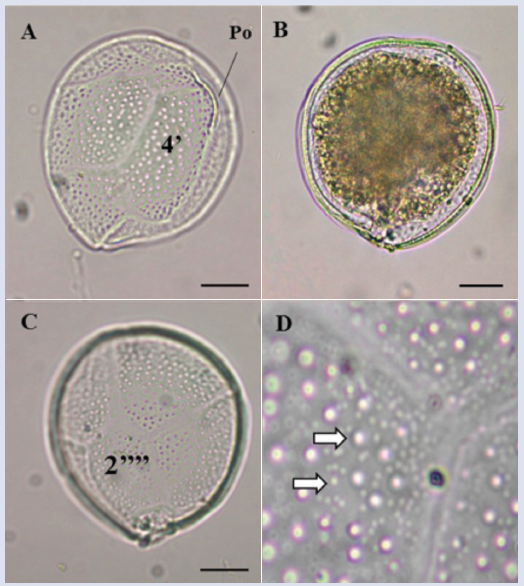
Figure 8 Micrographs of Ostreopsis cf. lenticularis under optical microscope. A. View of apical region indicating the plates and the apical pore (Po). B-C. Internal view of the plates. D. Detail of the pores that cover the cell (arrows indicate the two different thecal pores). Scale bar (A-C) = 20 μm.
Habitat: Benthic species attached to rocks and other substrates or epiphytes of macrophytes in shallow circumtropical waters (Hallegraeff et al., 2004).
Toxicity: The species has been recorded as a potentially toxic producer of palytoxins and other analogous toxins (Tosteson et al., 1989; Ashton et al., 2003; Granéli et al., 2011) that are harmful to some organisms, including humans. The toxicity of the species in the region has not been confirmed.
Prorocentrum hoffmannianum Faust, 1990.
Synonym: Exuviaella hoffmanniana (Faust) McLachlan, Boalch et Jahn1997. Exuviaella hoffmannianum (Faust) McLachlan et Boalch (Hoppenrath et al., 2014).
Description: The cell has a broad, tear-like, oval shape that is somewhat narrower towards the apical area, with a flattening towards the center of the cell where the pyrenoids are located (Figure 9A). Dorsal-ventral length:
50.6 ± 3.1 μm transdiameter: 37.3 ± 2.4 μm (n = 38). The theca surface is reticulated-excavated (Hoppenrath et al., 2013), and reniform pores are dispersed on the plates (Figure 9G) but absent in the center. The V-shaped periflagellar area has eight plates (1-8) (Figure 9J and K) and a wide adjacent apical collar (Figure 9C and F). Periflagellar plate 1 has three slits (Figure 9J and K) that coincide with the description by Hoppenrath et al. (2013). The morphology of these cells presents variations in shape and size, even some of them with a slightly reduced apical collar were observed. The species is easily confused with P. sabulosum, P. tropicalis and P. belizeanum, but Herrera-Sepúlveda et al. (2015) confirmed by molecular analysis that there was no difference between P. hoffmannianum and P. belizeanum. Regarding the other species, Hoppenrath et al. (2014) suggested that a more detailed analysis was needed to establish the boundaries that separated them.
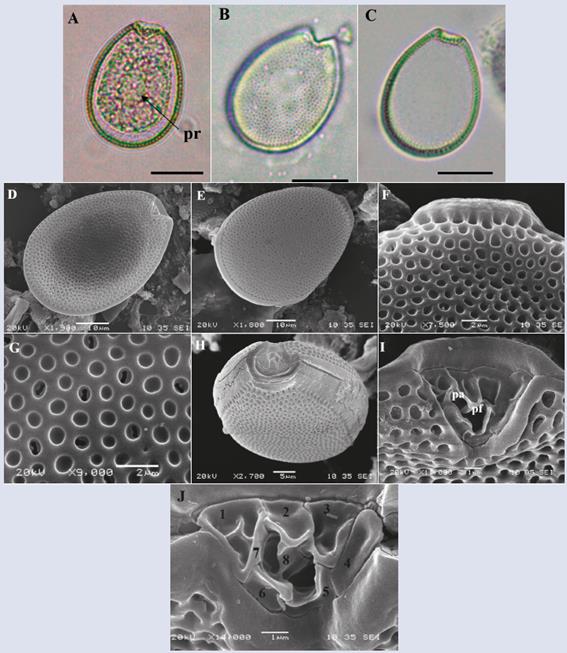
Figure 9 Micrographs of Prorocentrum hoffmannianum under optical microscope (A-C) and SEM (D-J). A. View of the right plate. B. Right plate with pore detail. C. Left plate. D. Cell in ventral view. E. Dorsal view. F. Detail of the apical collar. G. Detail of pores and areoles covering the cell. H-J. Periflagelar área (apical). J. Periflagelares plates, numbered followingHoppenrath et al. (2013). Pr: pirenoid, pa: accessory pore, pf: flagelar pore. Scale bar (A-C) = 20 μm, (D-E) = 10 μm, (F-G) = 2 μm, (H) = 10 μm, (I) = 5 μm, (J-K) = 1 μm.
Habitat: Marine species, benthic, widely distributed in tropical and subtropical areas, usually found on various substrates, such as sand, rocks or phanerogams (Chomérat et al., 2010).
Toxicity: A potentially toxic species that is a producer of okadaic acid and its analogues, which generate DSP (Faust et al., 1999; Hoppenrath et al., 2013).
Prorocentrum lima Stein, 1878.
Synonym: Cryptomonas lima Ehrenberg 1860.
Homotypic synonym: Exuviaella lima (Ehrenberg) Bütschli 1885, E. marina var. lima (Ehrenberg) Schiller 1931 (Guiry and Guiry, 2016).
Heterotypic synonyms: Exuviaella marina Cienkowski 1881, Dinopyxis laevis Stein 1883, E. laevis (Stein) Schroder 1900, E. cincta Schiller 1918, E. ostenfeldi Schiller 1933, E. caspica Kiselev 1940, P. marinum Dodge et Bibby 1973 and Prorocentrum arenarium Faust 1994 (Guiry and Guiry, 2016).
Description: The cell is ovoid, oval and oblong. Dorsoventral length: 40.4 ± 2.9 μm; transdiameter: 25.0 ± 2.0 μm (n = 42). In valve view, the cell is narrower towards the periflagellar area, ellipsoidal lenticulate in lateral view and slightly flattened towards the central portion, where prominent pyrenoids are internally located (Figure 10A), visible under the light microscope. The thecal surface is soft with small dispersed oval pores that form a marginal row and surround the periflagellar area (Figure 10C-F); the central portion of both thecal plates are devoid of pores (Figure 10B-D). The V-shaped periflagellar area with eight (1-8) plates adjacent to the flagellar pores (Figure 10G and H) coincides with the description of Hoppenrath et al. (2013). The species presents a wide variability of forms and sizes, which sometimes generates misidentifications.
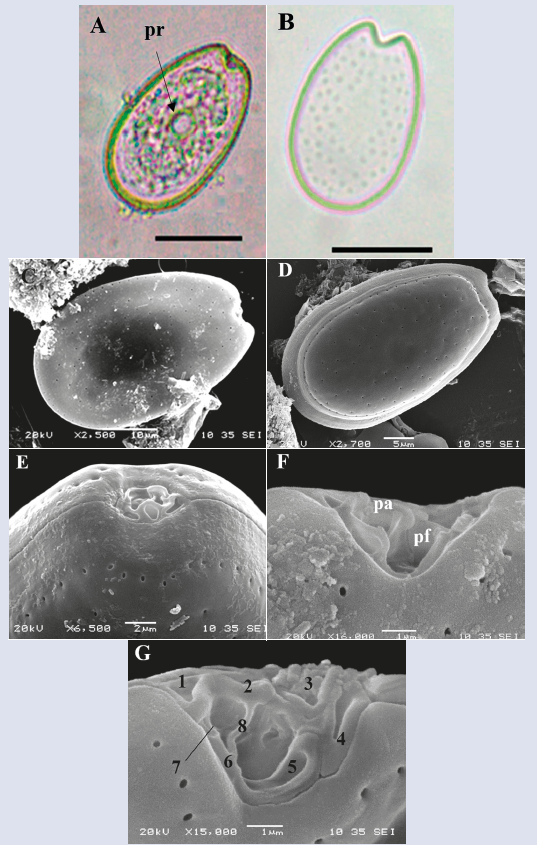
Figure 10 Micrographs of Prorocentrum lima under optical microscope (A-B) and SEM (C-G). A. Right theca. B. Right theca with pores. C. Right theca. D. Left theca. E-G. Periflagelar area (apical). G. Periflagelar plates, numbered followingHoppenrath et al. (2013). Pr: pirenoid, pa: accessory pore, pf: flagelar pore. flagelar. Scale bar (A-B) = 20 μm, (C and E) = 10 μm, (D) = 5 μm, (F) = 2 μm, (G-H) = 1 μm.
Habitat: Marine species, epibenthic, embedded in sandorattachedtovarioussubstrates, includingmacrophytes. Widely distributed in tropical waters (Hoppenrath et al., 2013, 2014). Fukuyo (1981) observed when performing cultures of this species that it normally adhered to the walls of the vessel, and cells were rarely found swimming freely. However, the species has been observed sporadically and in low densities in planktonic samples (Vila et al., 2001, Levasseur et al., 2003).
Toxicity: No toxicity has been confirmed in the study area. However, the species is considered potentially toxic and produces okadaic acid and other congeners that may cause diarrheic shellfish poisoning (DSP) (Murakami et al., 1982; Lee et al., 1989; Torigoe et al., 1988).
Prorocentrum emarginatum Fukuyo, 1981.
Description: The cell is asymmetric, rounded, slightly oval and slightly less broad towards the apical end (Figure 11A-D and F-G). In the lateral view, the thecal plates are slightly compressed dorsoventrally. Dorsoventral length: 36.7 ± 2.0 μm; transdiameter: 32.0 ± 1.5 μm (n = 8). The thecal texture is smooth with two types of pores in rows (Figure 11E) that are arranged radially from the periphery towards the center of the cell and are not completely present in the central portion of the theca (Figure 11C, D and F). A strongly marked V-shaped periflagellar area is observed (Figure 11B-C and F-G). In ventral view, it can be observed that the two apical shoulders are unequal, with the dorsal blunt and the ventral long and pointed (Figure 11 B-C and F), coinciding with the description of Hoppenrath et al. (2013, 2014). In the periflagellar area, a wing or spine-shaped structure protrudes from periflagellar plate 1 (Figure 11F and G). The species may be confused with Prorocentrum fukuyoi and P. scuptile; however, unlike P. fukuyoi, the cells observed do not have small pores in the center of the theca or possess a reticulated- excavated thecal surface as described for P. scuptile, which coincides with the description of P. emarginatum by Hoppenrath et al. (2013). However, a more detailed review at both the morphological and molecular levels is required to establish a clear difference between these three species and to understand the variability of the different characteristics.
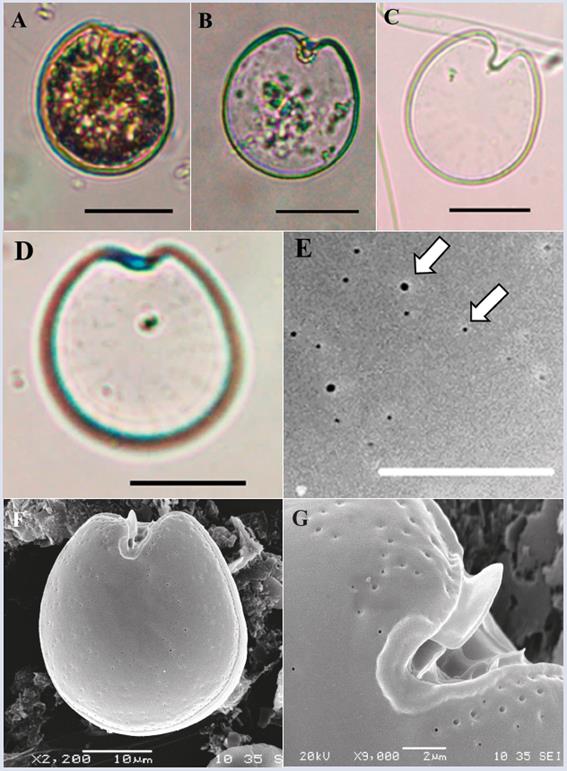
Figure 11 Micrographs of Prorocentrum emarginatum under optical microscope (A-D) and SEM (E-G). A-B, Right theca. C. Right theca slightly showing the pore pattern. D. Left theca. E. Detail of the two types of thecal pores (white arrows). F. Ventral view. G. Detail of the periflagelar area and of the apical wing. Scale bar (A-D) = 20 μm, (E) = 5 μm, (F) = 10 μm, (G) = 2 μm.
Habitat: Epibenthic marine species common in Caribbean waters. Faust et al. (1999) recorded this species in low densities on sediment, such as rocks or dead coral, attached to macrophytes and even on floating material.
Toxicity: The toxicity of this species has not been confirmed, although its presence has been associated with other benthic toxin-producing dinoflagellates (Faust et al., 1999).
Prorocentrum rhathymum Loeblich III, Sherley and Schmidt, 1979.
Description: Cell with asymmetric shape, oval and oblong. Dorsoventral length: 32.1 ± 3.8 μm; transdiameter: 22.4 ± 2.0 μm (n = 5), with pyrenoids present (Figure 12A). The texture of the thecal surface is soft and presents a characteristic pore pattern with a row of apical pores and some rows of radial pores towards the posterior end (Figure 12C and D). The central portion of the theca is devoid of pores (Figure 12C). A narrow U-shaped periflagellar area is observed; one end of periflagellar plate 1 extends as a spine projecting towards the right apical end of the cell (Figure 12A-B and D). There is controversy surrounding the taxonomy of this species because in some studies, Prorocentrum rhathymum has been considered synonymous with P. mexicanum (Faust, 1990, Steidinger and Tangen, 1996, Faust and Gulledge 2002), whereas in other studies it has been defined as a separate species (Cortés-Altamirano and Sierra-Beltrán, 2003). However, in a recent study based on morphological and molecular analyses, Gómez et al. (2017) confirmed that these were the same specie.
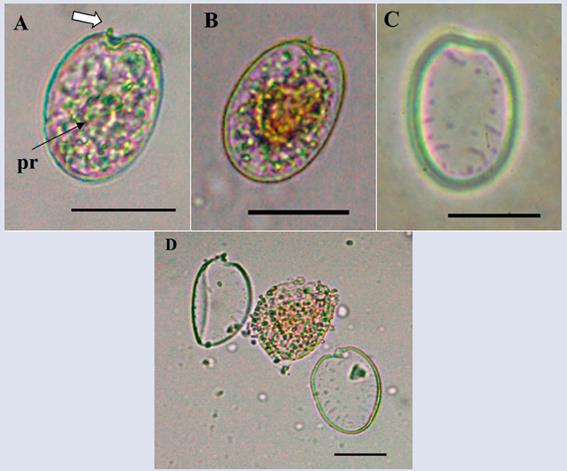
Figura 12 Micrographs of Prorocentrum rhathymum under optical microscope. A-B. Right theca view (arrows indicate the apical spine). C. Left theca view and pore patern. D. Separation of the two thecas, cytoplasmic material in the center. Pr: pirenoid. Scale bar (A-D) = 20 μm.
Habitat: Marine species, epibenthic, common on macroalgae and other substrates. This species is widely distributed in tropical and temperate waters (Hallegraeff et al., 2004).
Toxicity: Potential toxin-producer. Bomber and Aikman (1989) recorded the presence of hemolytic toxins in cultures of the species. No toxicity has been confirmed in the sampling area.
Prorocentrum cf. concavum Fukuyo, 1981
Description: The cell is wide with a rounded ovoid shape that is somewhat narrow towards the apical portion (Figure 13). Dorsoventral length: 44.9 ± 0.6 μm; transdiameter: 39.3 ± 1.3 μm; (n = 3), with the presence of pyrenoids (Figure 13A and B). The thecal surface is reticulated-excavated with pores, absent in the center of the thecal plates (Figure 13D) and become denser towards the margins of the cell. The periflagellar area is noticeably pronounced towards the V-shaped lateral ends (Figure 13A and B). It was not possible to perform a more detailed study of the organism to confirm the species because only one pair of cells was observed in the samples.
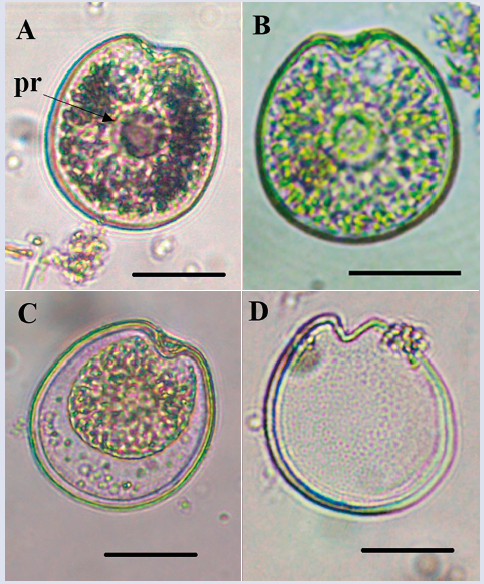
Figure 13 Micrographs of Prorocentrum cf. concavum under optical microscope. A-C. Right theca. D. Right theca with thecal pores. Pr: pirenoid. Scale bar = 20 μm.
Habitat: Marine organism, often associated with macroalgae and sediment (Faust et al., 1999) both in the Pacific (Fukuyo, 1981) and in the Caribbean (Valerio and Díaz, 2007; Almazán-Becerril et al., 2015).
Toxicity: P. concavum has been shown to be capable of producing potent toxins, including okadaic acid and some undefined ichthyotoxic compounds (Bomber and Aikman, 1989; Dickey et al., 1990; Faust et al., 1999), but the toxicity of this organism has not been verified in the sampling area.
Prorocentrum sp. 1
Description: The cell is symmetric, oblong oval and occasionally rounded. Dorsoventral length: 41.0 μm ± 2.3; transdiameter: 33.8 μm ± 2.4 (n = 24), with pyrenoids present (Figure 14). The texture of the plate surface is smooth, with small pores arranged irregularly that are difficult to observe under a light microscope (Figure 14D- F). The U-shaped periflagellar area is not very pronounced. Similar to P. cf. concavum, but differs in the cells size, pores patern (Figure 14D-F) and in the periflagellar area, which is wider in P. cf. concavum. A more detailed study of the organism is necessary to determine which species it belongs to.
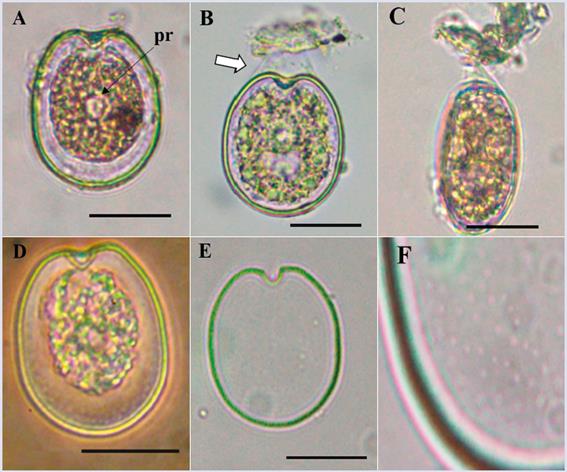
Figure 14 Micrographs of Prorocentrum sp1 under optical microscope. A. Right theca. B. Detail of a cell attached to a particle (arrow indicating mucus). C. Lateral view of a cell attached to detritus. D. Ventral view. E. Right plate. F. Detail of the thecal surface with small pores. Pr: pirenoid. Scale bar = 20 μm.
Prorocentrum sp. 2
Description: The cell is asymmetric with an oval oblong shape. Dorsoventral length: 47.0 μm ± 1.0; transdiameter: 32.0 μm ± 1.6 (n = 8), bean-shaped. The right margin is rounded, and the left margin shows a curvature towards the center of the cell. The thecal surface is soft-textured, with several rows of radial pores towards the posterior end of the cell. The V-shaped shallow periflagellar area has an elongated protruding apical spine that is not always visible in all cells because it appears to lie horizontally over the periflagellar area (Figure 15A and E). The species presents characteristics similar to those of P. rhathymum and P. shikokuense but differs from these species in their shapes and sizes; the cell is much larger than P. rhathymum, which does not have the curvature to the left end of the cell. Compared to P. shikokuense, the difference in the pore pattern is clear. In the cells observed in the present study, the wide striated intercalary band described by Su-Myat and Koike (2013) was not detected. A more detailed study of the organism is necessary for identification at the species level. We could not obtain further information due to its low frequency in the samples during the sampling period.
BIODIVERSITY
In general terms, the species richness in the study area (14 species) remained within the range reported by other authors. Previous research conducted in French Polynesia, New Caledonia, Japan, Cuba and Mexico recorded less than 25 species forming epiphytic/benthic dinoflagellate assemblies on various substrates (Fukuyo, 1981; Vila et al., 2001; Delgado et al., 2006; Okolodkov et al., 2007, 2014; Peraza and Moreira, 2012; Almazán-Becerril et al., 2015; Martínez-Cruz et al., 2015), with a species composition similar to that observed in Chengue. However, the number of species in the present study was higher than that recorded by Rodríguez et al. (2010), who quantified a total of eight epiphytic species on T. testudinum on the island of San Andres, Colombian Caribbean. Notably, the sampling performed by these authors was for a shorter period of time (two months), which could explain this difference.
Morphological variability (shape and size) was evident in some species in both the descriptions and the images obtained, which made identification at the species level using traditional light microscopy methods complex and even confusing. Different strategies need to be implemented for correct identification, such as establishing cultures to obtain sufficient cells for observation under a scanning electron microscope and analysis from different angles. Additionally, the optical analysis must be complemented with a genetic analysis by applying molecular techniques to support the results. Correct identification of the species is fundamental for any study that intends to provide elements for risk management against toxic or harmful effects of benthic dinoflagellates.
ACKNOWLEDGEMENTS
The authors are most grateful to Marine and Coastal Research Institute “Jose Benito Vives de Andreis”. INVEMAR (Contribution N°1162), to the Ministry of Environment and Suitable Development (MADS) and the International Anatomic Energy Agency (IAEA), co-funders of the project “Establishment of an observation net in the Caribbean to evaluate the acidification of the ocean and its impact bloosoming of harmful algae through the use of nuclear and isotropic techniques”, wich provided basic information to furge the present study. Special thanks are expressed to the Universidad Nacional de Colombia, the Marine Science Institute - Cecimar (Caribbean office) and the Biology Postgraduated program - Marine Biology Line (N°461) Bogotá office, HERMES project 28292. The Banco Español de Algas de Gran Canarias, specially Mr. E. Soler Onis and the Botanical Garden of Gran Canarias “Viera y Clavijo” are warmly thanked for their support in methodology and in the use of MEB. Thanks are also expressed to Mr. E. Arteaga for his support in the laboratory and in the field, and to Mr. S. Fraga for his collaboration in confirming the identification of several species. This document is part of the Master´s thesis of the first author.
REFERENCES
Almazán-Becerril. A., S. Escobar-Morales, G. Rosiles-González and F. Valadez. 2015. Benthic-epiphytic dinoflagellates from the northern portion of the Mesoamerican Reef System. Bot. Mar., 58(2): 115-128. [ Links ]
Álvarez, R. 1997. Primer caso de ciguatera en el Caribe colombiano por Seriola zonata (Mitchill, 1815) (Pisces: Carangidae). Biomédica, 17: 67-68. [ Links ]
Álvarez, R. 1999. Tres brotes de ictiosarcotoxicosis por ingestión de Sphyraena barracuda (Walbaum) y Seriola zonata (Mitchill) en el Caribe de Colombia. Biomédica , 9:35-38. [ Links ]
Álvarez-León, R., G. Ramírez-Triana y E. Wedler. 1995. Registro de las condiciones ambientales de la laguna sur (bahía de Chengue, Caribe colombiano) entre 1981-1984. Rev. Acad. Colomb. Cienc., 19 (74): 509-525. [ Links ]
An, T., J. Winshell, G. Scorzetti, J.W. Fell and K.S. Rein. 2010. Identification of okadaic acid production in the marine dinoflagellate Prorocentrum rhathymum from Florida Bay. Toxicon, 55(2-3): 653-657. [ Links ]
Arcila-Herrera, H., J. Mendoza-Ayora, F. González-Franco, L. Montero-Cervantes y A. Castelo-Navarrete. 2001. Revisión de una enfermedad poco conocida: la ciguatera. Rev Biomed., 12:27-34. [ Links ]
Ashton, M., T. Tosteson and C. Tosteson. 2003. The effect of elevated temperature on the toxicity of the laboratory cultured dinoflagellate Ostreopsis lenticularis (Dinophyceae). Rev. Biol. Trop., 4:1-6. [ Links ]
Besada, E.G., L.A. Loeblich and A.R. Loeblich. 1982. Observations on tropical, benthic dinoflagellates from ciguatera-endemic areas: Coolla, Gambierdiscus, and Ostreopsis. Bull. Mar. Sci., 32(3): 723-735. [ Links ]
Bomber, J. W. and K. E. Aikman. 1989. The ciguatera dinoflagellates. Biol. Oceanogr., 6: 291-311. [ Links ]
Brissard, C., C. Herrenknecht, V. Séchet, F. Hervé, F. Pisapia, J. Harcouet, R. Lémée, N. Chomérat, P. Hess and Z. Amzil. 2014. Complex toxin profile of French Mediterranean Ostreopsis cf. ovata strains, seafood accumulation and ovatoxins prepurification. Mar. Drugs, 12: 2851-2876. [ Links ]
Celis, J.S. y J.E. Mancera-Pineda. 2015. Análisis histórico de la incidencia de ciguatera en las islas del Caribe durante 31 años: 1980-2010. Bol. Invest. Mar. Cost., 44: 7-32. [ Links ]
Chomérat, N., D.Y. Sellos, F. Zentz and E. Nézan. 2010. Morphology and molecular phylogeny of Prorocentrum consutum sp nov. (Dinophyceae), a new benthic dinoflagellate from south Brittany (northwestern France). J. Phycol., 46(1): 183-194. [ Links ]
Ciminiello, P., C. Dell’Aversano, E. Fattorusso, M. Forino, L. Tartaglione, C. Grillo and N. Melchiorre. 2011. Putative palytoxin and its new analogue, ovatoxin-a, in Ostreopsis ovata collected along the Ligurian coasts during the 2006 toxic outbreak. J. Am. Soc. Mass. Spect., 19: 111-120. [ Links ]
Ciminiello, P., C. Dell’Aversano, E. Dello Iacovo, E. Fattorusso, M. Forino, L. Tartaglione, G. Benedettini, M. Onorari, F. Serena, C. Battocchi, S. Casabianca and A. Penna. 2014. First finding of Ostreopsis cf. ovata toxins in marine aerosols. Environ. Sci. Technol., 48(6): 3532-3540. [ Links ]
Cortés-Altamirano, R. and A.P. Sierra-Beltrán. 2003. Morphology and taxonomy of Prorocentrum mexicanum and reinstatement of Prorocentrum rhathymum (Dinophyceae). J. Phycol., 39: 221-225. [ Links ]
Del Favero, G., S. Silva, M. Pelin, E. D’Orlando, C. Florio, P. Lorenzon, M.Poli and A. Tubaro . 2012. Sanitary problems related to the presence of Ostreopsis spp. in the Mediterranean Sea: a multidisciplinary scientific approach. Ann. Ist. Super. Ann. Ist. Super. Sanità, 48(4): 407-414. [ Links ]
Delgado, G. 2005. Dinoflagelados bentónicos tóxicos asociados a la ciguatera: abundancia, toxicidad y relación con los factores ambientales en un área del litoral noroccidental de Cuba. Tesis doctorado Ciencias del Mar, Centro Invest. Biol. Noroeste S.C. La Paz, México. 72 p. [ Links ]
Delgado, G., G. Popowski, C. García, N. Lagos and C.H. Lechuga-Devéz. 2005. Presence of DSP-toxins in Prorocentrum lima (Ehrenberg) Dodge in Cuba. Rev. Invest. Mar., 26(3): 229-234. [ Links ]
Delgado, G., C.H. Lechuga-Devéze, G. Popowski, L. Troccoli and C.A. Salinas. 2006. Epiphytic dinoflagellates associated with ciguatera in the northwestern coast of Cuba. Rev. Biol. Trop., 54(2): 299-310. [ Links ]
Dickey, R.W., S.C. Bobzin, D.J. Faulkner, F.A. Bencsath and D. Andrzejewski. 1990. Identification of okadaic acid from a Caribbean dinoflagellate, Prorocentrum concavum. Toxicon, 28(4): 371-377. [ Links ]
Faust, M. A. 1990. Morphologic details of six benthic species of Prorocentrum (Pyrrhophyta) from a mangrove island, Twin Cays, Belize, including two new species. J. Phycol., 26: 548-558. [ Links ]
Faust, M.A. 1992. Observations on the morphology and sexual reproduction of Coolia monotis (Dinophyceae). J. Phycol., 28: 94-104. [ Links ]
Faust, M.A. 1995. Observation of sand-dwelling toxic dinoflagellates (Dinophyceae) from widely differing sites, including two new species. J. Phycol., 31:996-1003. [ Links ]
Faust, M.A. 1999. Three new Ostreopsis species (Dinophyceae): O. marinus sp. nov., O. belizeanus sp. nov., and O. caribbeanus sp. nov. Phycologia, 38(2):92-99. [ Links ]
Faust, M.A. 2009. Ciguatera-causing dinoflagellates in a coral-reef-mangrove ecosystem, Belize. Atoll Res. Bull., 569: 1-32. [ Links ]
Faust, M.A. and R.A. Gulledge. 2002. Identifying harmful marine dinoflagellates. Smithson. Contr. U.S Nat. Herb., 42:1-144. [ Links ]
Faust, M.A., S.L. Morton and J.P. Quod. 1996. Further SEM study of marine dinoflagellates: The genus Ostreopsis (Dinophyceae). J. Phycol., 32: 1053-1065. [ Links ]
Faust, M.A., J. Larsen and Ø. Moestrup. 1999. Potentially toxic phytoplankton. 3. Genus Prorocentrum (Dinophyceae). ICES Identification Leaflets for Plankton. Ed. J.A. Lindley. Natural Environment Research Council. Copenhaguen. 24 p. [ Links ]
Fraga, S., A. Penna, I. Bianconi, B. Paz and M. Zapata. 2008. Coolia canariensis sp nov. (Dinophyceae), a new nontoxic epiphytic benthic dinoflagellate from the Canary Islands. J. Phycol., 44: 1060-1070. [ Links ]
Fraga, S., F. Rodríguez, A. Caillaud, J. Diogène, N. Raho and M. Zapata. 2011. Gambierdiscus excentricus sp. nov. (Dinophyceae), a benthic toxic dinoflagellate from the Canary Islands (NE Atlantic Ocean). Harmful Algae, 11: 10-22. [ Links ]
Fraga, S., F. Rodríguez, P. Riobó and I. Bravo. 2016. Gambierdiscus balechii sp. nov (Dinophyceae), a new benthic toxic dinoflagellate from the Celebes Sea (SW Pacific Ocean). Harmful Algae, 58: 93-105. [ Links ]
Fritz L. and R.E. Trimer. 1985. A rapid and simple technique utilizing calcofluor white MR2 for visualization of dinoflagellate technology. J. Phycol., 21:662-64. [ Links ]
Fukuyo, Y. 1981. Taxonomical study on benthic dinoflagellates collected in coral reefs. Bull. Jpn. Soc. Sci. Fish., 47:967-78. [ Links ]
Gaitán, J.D. 2007. Ictiotoxismo por consumo de barracuda (Sphyraena barracuda) y morena manchada (Gymnothorax moringa) en la comunidad de pescadores artesanales de Tasajera, Caribe colombiano. Duazary, 4(2): 160-167. [ Links ]
Gallitelli, M., N. Ungaro, L.M. Addante, V. Procacci, N.G. Silveri and C. Sabbá. 2005. Respiratory illness as a reaction to tropical algal blooms occurring ina temperate climate. JAMA, 293(21): 2599-2600. [ Links ]
Gómez, F., D. Qiu, E. Otero-Morales, R.M. Lopes and S. Lin. 2016. Circumtropical distribution of the epiphytic dinoflagellate Coolia malayensis (Dinophyceae): Morphology and molecular phylogeny from Puerto Rico and Brazil. Phycol. Res., 64: 194-199. [ Links ]
Gómez, F., D. Qiu and S. Lin. 2017. The synonymy of the toxic dinoflagellates Prorocentrum mexicanum and P. rhathymum and the description of P. steidingerae sp. nov. (Prorocentrales, Dinophyceae). J. Eukaryot. Microbiol., 0: 1-10. [ Links ]
Gorbi, S., R. Bocchetti, A. Binelli, S. Bacchiocchi, R. Orletti, L. Nanetti, F. Raffaelli, A. Vignini, S. Accoroni, C. Totti and F. Regoli. 2012. Biological effects of palytoxin-like compounds from Ostreopsis cf. ovata: A multibiomarkers approach with mussels Mytilus galloprovincialis. Chemosphere, 89:623-632. [ Links ]
Granéli, E., N.K. Vidyarathna, E. Funari, P.R.T. Cumaranatunga and R. Scenati. 2011. Can increases in temperature stimulate blooms of the toxic benthic dinoflagellate Ostreopsis ovata?. Harmful Algae, 10: 165-172. [ Links ]
Guiry, M.D. and G.M 2016. AlgaeBase. World-wide electronic publication, National University of Ireland, Galway. http://www.algaebase.org ; Consultado 15/01/2016. [ Links ]
Hallegraeff, G.M., D.M. Anderson and A.D. Cembella. 2004. Manual on harmful marine microalgae. Monographs on Oceanographic Methodology, UNESCO. París. 793 P. [ Links ]
Herrera-Sepúlveda, A., L. Medlin, G. Murugan, A.P. Sierra-Beltrán, A.A. Cruz-Villacorta and N.Y. Hernández-Saavedra. 2015. Are Prorocentrum hoffmannianum and Prorocentrum belizeanum (Dinophyceae, Prorocentrales) the same species? An integration of morphological and molecular data. J. Phycol., 51: 173-188. [ Links ]
Holland, W., W. Litaker, C.R. Tomas, S.R. Kibler, A.R. Place, E.D. Davenport and P.A. Tester. 2013. Differences in the toxicity of six Gambierdiscus (Dinophyceae) species measured using an in vitro human erythrocyte lysis assay. Toxicon, 65: 15-33. [ Links ]
Holmes M., R.J. Lewis, M.A. Poli and N.C. Gillespie. 1991. Strain dependent production of ciguatoxin precursors (gambiertoxins) by Gambierdiscus toxicus (Dinophyceae) in culture. Toxicon, 29(6): 761-75. [ Links ]
Holmes, M., R. Lewis, A. Jones and A. Wong. 1995. Cooliatoxin, the first toxin from Coolia monotis (Dinophyceae). Nat. Toxins, 3:355-362. [ Links ]
Hoppenrath, M., N. Chomérat, T. Horiguchi, M. Schweinkert, Y. Nagahama and S. Murray. 2013. Taxonomy and phylogeny of the benthic Prorocentrum species (Dinophyceae) - A proposal and review. Harmful Algae, 27: 1-28. [ Links ]
Hoppenrath, M., S. A. Murray, N. Chomérat and T. Horiguchi. 2014. Marine benthic dinoflagellates - unveiling their worldwide biodiversity. Ed. Senckenberg. Stuttgart. 276 p. [ Links ]
Jeong, H.J., A.S. Lim, S.H. Hang, W.O. Yih, N.S. Kang, S.Y Lee, Y.D. Yoo and H.S Kim. 2012. First report of the epiphytic dinoflagellate Gambierdiscus caribaeus in the temperate waters off Jeju Island, Korea: morphology and molecular characterization. J. Eukaryot. Microbiol., 59(6): 637-650. [ Links ]
Karafas, S., R. York and C. Tomas. 2015. Morphological and genetic analysis of the Coolia monotis species complex with the introduction of two new species, Coolia santacroce sp. nov. and Coolia palmyrensis sp. nov. (Dinophyceae). Harmful Algae, 46: 18-33. [ Links ]
Leaw, C-P., P-T. Lim, K-W. Cheng, B-K. Ng and G. Usup. 2010. Morphology and molecular characterization of a new species of thecate benthic dinoflagellate, Coolia malayensis sp. nov (Dinophyceae). J. Phycol., 46: 162-171. [ Links ]
Lee, J.S., T. Igarashi, S. Fraga, E. Dahl, P. Hovgaard and T. Yasumoto. 1989. Determination of diarrhetic shellfish toxins in various dinoflagellate species. J. Appl. Phycol., 1: 147-152. [ Links ]
Lenoir, S., L. Ten-Hage, T. Turquet, J.P. Quod, C. Bernard and M.C. Hennion. 2004. First evidence of palytoxin analogues from an Ostreopsis mascarenensis (Dinophyceae) benthic bloom in southwestern Indian Ocean. J. Phycol., 40: 1042-1051. [ Links ]
Levasseur, M., J. Couture, A.M. Weise, S. Michaud, M. Elbrächter, G. Sauvé and E. Bonneau. 2003. Pelagic and epiphytic summer distributions of Prorocentrum lima and P. mexicanum at two mussel farms in the Gulf of St. Lawrence, Canada. Aquat. Microb. Ecol., 30: 283-293. [ Links ]
Lewis, R.J., M. Inserra, I. Vetter, W.C. Holland, D.R. Hardison, P.A. Tester and R.W. Litaker. 2016. Rapid extraction and identification of maitotoxin and ciguatoxin-like toxins from Caribbean and Pacific Gambierdiscus using a new functional bioassay. PLoS One, 11(7): e0160006. [ Links ]
Litaker, R.W., M. Vandersea, M. Faust, S. Kibler, M. Chinain, M. Holmes, W. Holland and P. Tester . 2009. Taxonomy of Gambierdiscus including four new species, Gambierdiscus caribaeus, Gambierdiscus carolinianus, Gambierdiscus carpenteri and Gambierdiscus ruetzleri (Gonyaulacales, Dinophyceae). Phycologia, 48(5): 344-390. [ Links ]
Litaker, R.W., M. Vandersea, M. Faust, S. Kibler, A.W. Nau, W. Holland, M. Chinain, M. Holmes and P.A. Tester. 2010. Global distribution of ciguatera causing dinoflagellates in the genus Gambierdiscus. Toxicon, 56: 711-730. [ Links ]
Mancera-Pineda, J.E., B. Gavio y G. Arencibia. 2009. Floraciones algales nocivas, intoxicación por microalgas e impactos en el desarrollo regional: el caso de San Andrés isla, Caribe colombiano, 46-62. En: Mancera-Pineda, J.E., O. Sierra y S. Pérez. (Eds.) 2009. Cuadernos del Caribe, 13, 78 p. [ Links ]
Martínez-Cruz, M.E., Y. Okolodkov, A.C. Aguilar-Trujillo and J.A. Herrera-Silveira. 2015. Epiphytic dinoflagellates on the seagrass Thalassia testudinum at Dzilam, southeastern Gulf of Mexico. Cymbella, 3: 2-9. [ Links ]
Mohammad-Noor, N., N. Daugbjerg, Ø. Moestrup and A. Anton. 2007. Marine epibenthic dinoflagellates from Malaysia - a study of live cultures and preserved samples based on light and scanning electron microscopy. Nord. J. Bot., 24(6): 629-690. [ Links ]
Mohammad-Noor, N., Ø. Moestrup, N. Lundholm, S. Fraga, A. Adam, M.J. Holmes and E. Saleh. 2013. Autecology and phylogeny of Coolia tropicalis and Coolia malayensis (Dinophyceae), with emphasis on taxonomy of C. tropicalis based on light microscopy, scanning electron microscopy and LSU rDNA. J. Phycol., 49: 536-545. [ Links ]
Morton, S.L. and J.W. Bomber. 1994. Maximizing okadaic acid content from Prorocentrum hoffmannianum Faust. J. Appl. Phycol., 6: 41-44. [ Links ]
Murakami, Y., Y. Oshima and T. Yasumoto. 1982. Identification of okadaic acid as a toxic component of a marine dinoflagellate Prorocentrum lima. Nippon Suisan Gakk., 48: 69-72. [ Links ]
Nascimento, S., E.V. Corrêa, M. Menezes, D. Varela, J. Paredes and S. Morris. 2012. Growth and toxin profile of Ostreopsis cf. ovata (Dinophyta) from Río de Janeiro, Brazil. Harmful Algae, 13: 1-9. [ Links ]
Okolodkov, Y., F. Merino-Virgilio, J. Aké- Castillo, A. Aguilar-Trujillo, S. Espinosa-Matías and J. Herrera-Silveira. 2014. Seasonal changes in epiphytic dinoflagellate assemblages near the northern coast of the Yucatan Peninsula, Gulf of Mexico. Acta Bot. Mex., 107: 121-151. [ Links ]
Parsons, M.L., K. Aligizaki, M.-Y. Dechraoui Bottein, S. Fraga, S.L. Morton, A. Penna and L. Rhodes. 2012. Gambierdiscus and Ostreopsis: Reassessment of the state of knowledge of their taxonomy, geography, ecophysiology, and toxicology. Harmful Algae, 14: 107-129. [ Links ]
Penna, A., M. Vila, S. Fraga, M.G. Giacobbe, F. Andreoni, P. Riobó and C. Vernesi. 2005. Characterization of Ostreopsis and Coolia (Dinophyceae) isolates in the western Mediterranean Sea based on morphology, toxicity and internal transcribed spacer 5.8s rDNA sequences. J. Phycol., 41: 212-225. [ Links ]
Peraza, R. y Á. Moreira. 2012. Composición y abundancia de dinoflagelados epibentónicos tecados potencialmente tóxicos de la Ensenada Guajimico, región Centro-Sur de Cuba. Rev. Cub. Invest. Pesq., 29: 59-66. [ Links ]
Reguera, B., R. Alonso, A. Moreira y S. Méndez. 2011. Guía para el diseño y puesta en marcha de un plan de seguimiento de microalgas productoras de toxinas. IOC Manuals and Guides 59, COI de UNESCO y OIEA, París y Viena, 46 p + Anexos. [ Links ]
Rhodes, L., J. Adams, T. Suzuki, L. Briggs and I. Garthwaite. 2000. Toxic marine epiphytic dinoflagellates, Ostreopsis siamensis and Coolia monotis (Dinophyceae), in New Zealand. N. Z. J. Mar. Fresh., 34: 371-383. [ Links ]
Rodríguez, A., J.E. Mancera-Pineda and B. Gavio. 2010. Survey of benthic dinoflagellates associated to beds of Thalassia testudinum in San Andrés Island, Seaflower Biosphere Reserve, Caribbean Colombian. Acta Biol. Col., 15(2): 231-248. [ Links ]
Rodríguez-Ramírez, A., J. Nivia-Ruíz y J. Garzón-Ferreira. 2004. Características estructurales y funcionales del manglar de Avicennia germinans en la Bahía de Chengue (Caribe colombiano). Bol. Invest. Mar. Cost., 33: 223-244. [ Links ]
Steidinger, K. and K. Tangen. 1996. Dinoflagellates. 387-598. In: C.R. Tomas (Ed.). 1996. Identifying Marine Diatoms and Dinoflagellates. Academic Press, New York. [ Links ]
Su-Myat and K. Koike. 2013. A red tide off the Myanmar coast: Morphological and genetic identification of the dinoflagellate composition. Harmful Algae, 27(7):149-158. [ Links ]
Tester, P.A., S. Kibler, W. Holland, G. Usup, M. Vandersea, C.P. Leaw, L.P. Teen, J. Larsen, N. Mohammad-Noor, M.A. Faust and R.W. Litaker. 2014. Sampling harmful benthic dinoflagellates: Comparison of artificial and natural substrate methods. Harmful Algae, 39: 8-25. [ Links ]
Tichadou, L., M. Glaizal, A. Armengaud, H. Grossel, R. Lemée, R. Kantin, J.L. Lasalle, G. Drouet, L. Rambaud, P. Malfait and L. De Haro. 2010. Health impact of unicellular algae of the Ostreopsis genus blooms in the Mediterranean Sea: experience of the French Mediterranean coast surveillance network from 2006 to 2009. Clin. Toxicol., 48(8): 839-844. [ Links ]
Torigoe, K., M. Murata and T. Yasumoto. 1988. Prorocentrolide, a toxic nitrogenous macrocycle from a marine dinoflagellate, Prorocentrum lima. J. Am. Chem. Soc., 110: 7876-7877. [ Links ]
Tosteson, T.R., D.L. Ballantine, C.G. Tosteson, V. Hensley and A.T. Bardales. 1989. Associated bacterial flora, growth, and toxicity of cultured benthic dinoflagellates Ostreopsis lenticularis and Gambierdiscus toxicus. Appl. Environ. Microbiol., 55(1): 137-41. [ Links ]
Tubaro A., P. Durando, G. Del Favero, F. Ansaldi, G. Icardi, J.R. Deeds and S. Sosa. 2011. Case definitions for human poisonings postulated to palytoxins exposure. Toxicon, 57(3): 478-495. [ Links ]
Valerio, L. y J. Díaz. 2007. Dinoflagelados epifitos potencialmente tóxicos presentes en praderas de Thalassia testudinum en las costas nororientales de Venezuela. Rev. Fac. Agron., 24(1): 39-43. [ Links ]
Valerio, L. y J. Díaz. 2008. Distribución de dinoflagelados epifitos potencialmente tóxicos asociados a praderas de Thalassia testudinum en la isla la Tortuga, la bahía de Mochima y golfo de Cariaco, Venezuela. Bol. Inst. Oceanogr., 47(1): 47-58. [ Links ]
Vila, M., E. Garcés and M. Masó. 2001. Potentially toxic epiphytic dinoflagellate assemblages on macroalgae in the NW Mediterranean. Aquat. Microb. Ecol., 26: 51-60. [ Links ]
Yasumoto, T., N. Seino, Y. Murakami and M. Murata. 1987. Toxins produced by benthic dinoflagellates. Biol. Bull., 172: 128-131. [ Links ]
Received: October 24, 2016; Accepted: July 08, 2017