Servicios Personalizados
Revista
Articulo
Indicadores
-
Citado por SciELO
-
Accesos
Links relacionados
-
Citado por Google
-
Similares en SciELO
-
Similares en Google
Compartir
Boletín de Investigaciones Marinas y Costeras - INVEMAR
versión impresa ISSN 0122-9761
Bol. Invest. Mar. Cost. vol.46 no.2 Santa Marta jul,/dic. 2017
https://doi.org/10.25268/bimc.invemar.2017.46.2.726
Research Articles
Coral reef bioerosion in the Colombian Pacific: Diadema mexicanum (Echinoidea: Diadematidae) study case
1Universidad del Valle, Departamento de Biología, Grupo de Investigación en Ecosistemas Rocosos Intermareales y Submareales Someros-LITHOS, Apartado aéreo 25360, Cali, Colombia. levy.obonaga@correounivalle.edu.co
2Universidad del Valle, Departamento de Biología, Grupo de Investigación en Ecosistemas Rocosos Intermareales y Submareales Someros-LITHOS, Apartado aéreo 25360, Cali, Colombia. maurozucconi@correounivalle.edu.co
3Universidad del Valle, Departamento de Biología, Grupo de Investigación en Ecosistemas Rocosos Intermareales y Submareales Someros-LITHOS, Apartado aéreo 25360, Cali, Colombia. edgardo.londono@correounivalle.edu.co
Echinoids are an important component of benthic communities; they can directly modify the distribution and relative abundance of algae and corals as well as be an agent in the process of bioerosion. Despite research on echinoids in the Colombian Pacific, the bioerosion rate due to grazing by Diadema mexicanum on the coral reefs of Gorgona Island remains unknown. Therefore, considering the relative high abundance of this echinoid and its potential negative effects on coral reefs, the main objective of this study was to determine the rate of bioerosion. To accomplish this, 1-m2 quadrats were randomly established in each of three reef zones (at low tide, Backreef: ±1 m depth, Reef plain: ±0.5 m and Reef front: ±3.0 m) of La Azufrada fringing reef (Gorgona Island National Natural Park). Sea urchins were counted and measured (test diameter) inside the quadrats, and 30 urchins per zone (90 in total) were collected to estimate the bioerosion rate. The average sea urchin density and size (±SD) were 8.28±11.65 ind/m2 and 19.62±5.02 mm, respectively. The average bioerosion rate for the reef was 0.083 kg CaCO3/m2/yr, and it was significantly affected by echinoid size (p<0.001) and reef zone (p=0.0002). Additionally, a direct relationship was observed between intestinal calcium carbonate (CaCO3) and depth (p=0.043) and size (p<0.001). Finally, although large sea urchins may have important effects, it is unlikely that the bioerosion caused by D. mexicanum presents a threat to La Azufrada fringing reef due to its low relative abundance; this is reflected by the relatively low bioerosion rate relative to other reefs in the Tropical Eastern Pacific.
Keywords: Bioerosion; Eastern Pacific; Sea urchin; Coral reefs; Gorgona Island
Los equinoideos son un componente importante de las comunidades bentónicas y pueden llegar a modificar directamente la distribución y abundancia relativa de algas y corales, y ser un agente importante en el proceso de bioerosión. A pesar de los estudios realizados en el Pacífico colombiano sobre equinoideos, se desconoce la tasa de bioerosión por ramoneo del equinodermo Diadema mexicanum en los arrecifes de la isla Gorgona; considerando las abundancias de este equinoideo y su potencial efecto sobre el ecosistema coralino, en este trabajo se calculó la tasa de bioerosión. Para esto, se efectuaron muestreos aleatorios con cuadrantes de 1 m2 en las tres zonas (Trasarrecife ±1.0 m de profundidad, Planicie ±0.5 m y Frente ±3.0 m en marea baja) del arrecife La Azufrada (Parque Nacional Natural Isla Gorgona). Los erizos dentro del cuadrante fueron contados y medidos (ancho de la testa). En adición, se capturaron 90 individuos (30 por zona), para determinar la tasa de bioerosión. La densidad y talla promedio fueron de 8.28±11.65 ind./m2 y 19.62±5.02 mm, respectivamente. La tasa promedio de bioerosión fue de 0.083 KgCaCO3/m2/año, y se vio afectada significativamente por el tamaño del equinoideo (p0.001) y la zona arrecifal (p=0.0002). También se observó una relación directa entre la cantidad de carbonato de calcio intestinal y la profundidad (p=0.043) y la talla (p0.001). Finalmente, se puede afirmar que, aunque los erizos grandes pueden ocasionar un efecto importante sobre el arrecife, debido a su baja abundancia relativa es probable que la erosión causada por D. mexicanum no sea una amenaza para el arrecife La Azufrada, lo que se ve reflejado en una tasa de bioerosión relativamente baja en comparación con otros arrecifes del Pacífico Oriental Tropical.
Palabras clave: Bioerosión; Pacífico Oriental; Erizo de mar; Arrecife coralino; Isla Gorgona
INTRODUCTION
Coral reefs are one of the most biodiverse ecosystems in the world (Hoegh-Guldber et al., 2007); however, some of the species these ecosystems harbor, cause bioerosion. According to Neumann (1966), bioerosion is the destruction and removal of consolidated material or lytic substrate by the direct action of organisms, which have been divided into microborers, macroborers and grazers (Tribollet and Golubic, 2011). Grazers are mainly fish [e.g., Labridae (subfamily Scarinae), Balistidae and Tetraodontidae] and regular urchins (e.g., Diadematidae and Cidaridae) (Downing and El-Zahr, 1987; Hernández- Ballesteros et al., 2013; Londoño-Cruz et al., 2003).
The echinoids, or regular sea-urchins, are a group of benthic fauna that play an important ecological role in community structure (Herrera-Escalante et al., 2005; Barberá et al., 2011). These organisms are generally herbivorous and feed on both micro- and macroalgae (Lawrence, 1975), which they detach from the substrate using their beak (Aristotle’s lantern). Using this method of feeding, known as grazing, sea-urchins remove (i.e., bioerode) small fractions of the material on which the algae grow, i.e., calcium carbonate (CaCO3) in the case of coral reefs. When the densities of echinoid populations are low, the effects of grazing on the reef community, particularly on corals, can be positive, as it opens space for coral settlement (Sammarco, 1982: Morrison, 1988; Sánchez-Jeréz et al., 2001; Dumont et al., 2013), but when densities are high, rates of bioerosion may be excessive and may cause reef deterioration and weakening (Glynn et al., 1979; Bak, 1990; Glynn and Leyte-Morales, 1997). In addition to population density, there are other factors, both biotic (e.g., predation, territoriality, population density and the size of individuals) and abiotic (e.g., time of day and reef area and depth), that affect the rate of bioerosion by echinoids (Glynn, 1988; Eakin, 1992; Bak, 1994; Nodarse, 2001; Toro-Farmer et al. 2002; Herrera-Escalante et al., 2005).
Some echinoid species in the genus Diadema stand out for their bioerosion capacity (e.g., D. antillarum Philippi in the Caribbean and Atlantic and D. savignyi (Audouin) and D. setosum (Leske) in the Indo-Pacific - Bak, 1990, 1994; Carreiro-Silva and McClanahan, 2001; Dumont et al., 2013). In the Tropical Eastern Pacific, D. mexicanum A. Agassiz is one of the most conspicuous species (Lawrence, 2007), and its bioerosive effect has been studied in regions such as the Galapagos (Ecuador), Panama, Costa Rica and Mexico (Glynn, 1988; Guzmán and Cortes, 1992; Herrera-Escalante et al., 2005; Alvarado et al., 2012). In Colombia, the reefs of Gorgona Island have been the subject of several studies (Zapata, 2001, Zapata and Vargas-Ángel, 2003, Zapata et al., 2010), but few have been conducted on echinoids, particularly D. mexicanum. In addition, the few studies on echinoids have focused more on their taxonomy and spatial distribution (Vargas-Ángel, 2003; Neira and Cantera, 2005) than on their role as bioeroders. The only records of echinoid bioerosion in the Colombian Pacific are from Toro-Farmer et al. (2002) in coral reefs (Playa Blanca, Gorgona Island) and Lozano et al. (2011) in rocky ecosystems (Palma Island, Málaga bay). Therefore, the objective of this work was to determine the rate of bioerosion by D. mexicanum on La Azufrada reef (Gorgona Island) and to evaluate if this rate was affected by the size of individuals, reef zone and depth.
STUDY AREA
Gorgona Island (2°58’N, 78°11’W), which is part of Gorgona National Natural Park (one of the five protected areas within the Colombian Pacific), located 30 km off Guapi (Cauca), the nearest populated area in the mainland, is the largest island (13.2 km2) on the Colombian Pacific continental shelf (Fig. 1). The position of Gorgona Island with respect to the Intertropical Convergence Zone determines the bi-seasonal climate and unimodal annual precipitation pattern (Zapata et al., 2010). The tidal cycle on the island is semidiurnal with an amplitude of approximately 4 m (Prahl et al., 1979). One of the main coral reefs on the island is La Azufrada, which is relatively small (approximately 0.094 km2) and underdeveloped. The main builders of the reef framework are branching corals of the genus Pocillopora (e.g., P. damicornis (Linnaeus) and P. capitata (Verril)) followed by some colonies of massive corals (e.g., Porites spp. and Pavona spp.). The reef lacks a clear zonation pattern; however, three zones can be recognized: Back-reef (TR), Reef flat (PL) and Reef front (FR). The coral cover of the first zone is less than 50% with patches of coral and sand, and its depth is ±1 m at low tide. The second zone is the shallowest area (±0.5 m) at low tide, and the living coral cover is between 80 and 90%. In the third zone, the coral cover drastically decreases as depth increases (±3.0 m). For more information on the coral reefs of the island, see Zapata (2001).
MATERIALS AND METHODS
Density, size and depth
Population samples were conducted in November 2014 and April and July 2015 following the method proposed by Toro-Farmer et al. (2002). Three hundred and twenty 1 m2 quadrats were randomly distributed in each reef zone, TR (n=100), PL (n=115) and FR (n=105). To determine body size, test width of all individuals within the quadrats was measured with an underwater digital caliper (Zebra Tech) that also recorded depth at which the measurement was made. All sampled individuals were classified into three size classes: Small (<15 mm, P), Medium (>15 mm-<20 mm, M) and Large (>20 mm, G) as proposed by Herrera-Escalante et al. (2005). To determine the intestinal content of CaCO3, the last individual of every ten that was counted (within the quadrat) was systematically collected for a total of 30 per reef zone. The collected urchins were subsequently fixed (injection with 10% formalin), properly labeled and transported in plastic containers (5 L) containing 10% formalin to the Marine Biology laboratories of the Universidad del Valle in Cali.
Bioerosion rate
The amount of intestinal CaCO3 in the 90 collected sea-urchins was determined following the protocol proposed by Carreiro-Silva and McClanahan (2001) with modifications: the digestive system was extracted, dried in an oven at 60 °C for 48 h and weighed on an analytical balance (precision of 0.0001 g). It was subsequently burned in a muffle furnace at 550 °C for 3 h to remove the organic material so that only the inorganic portion was preserved; the difference in weight before and after incineration was used to calculate the amount of organic matter. Finally, the inorganic fraction was treated with 5% HCl and washed with distilled water on filter paper before being dried at 60 °C for 12 h. The difference in the dry weight before and after digestion was used to calculate the amount of intestinal CaCO3 in each individual. To calculate the bioerosion rate, a 24-h intestinal turnover period was assumed (Glynn et al., 1979; Bak, 1990; Herrera-Escalante et al., 2005).
The bioerosion rate was calculated considering: 1) the average CaCO3 present in individuals of a given size (P, M, G) expressed in kg and 2) the average density of individuals of that size in each reef zone (TR, PL, FR). The sum of the partial rates by reef zones or size classes allowed us to calculate the total bioerosion rate for the reef, correcting for age density (see Equation 1).
Equation
Where:
TBT(Dm)=Total bioerosion rate by D. mexicanum in La Azufrada reef
i=Size class
j=Reef zone
ij =Average CaCO3 (in kg) for a given size class in a given reef zone
ƿij =Density of D. mexicanum of a given size in a given reef zone.
Data analysis
The population density of D. mexicanum was calculated for the entire reef and for each reef zone. The spatial distribution (i.e., clustered, uniform, random) of the population was determined using the dispersion index (I=variance/mean) and tested with a Chi-square (χ2) test (Elliot, 1977). Sea-urchin density was tested between reef zones with a generalized linear model under a negative binomial distribution due to the overdispersion of the data (z=3.71, p<0.001). Because size data was not normal (Anderson-Darling, A=6.52, p<0.0001) and homoscedastic (Levene, F=20.81; p<0.000), a randomization test was performed (10,000 iterations), from which a reference distribution was generated (Edgington and Onghena, 2007) in order to evaluate the effect of reef zones on sea-urchin size. When significant differences were found, multiple comparison tests were performed. The rate of bioerosion (transformed with natural logarithm) was evaluated using a factorial ANOVA with size (three levels) and reef zone (three levels) as explanatory factors. Because one of the factor combinations (P-TR) had no replicates, the interaction between factors was not included in the model. When significant differences were found, Tukey’s multiple comparison test was used. Finally, two linear regressions were performed to determine the dependence of the intestinal CaCO3 content on the size of the individuals and the capture depth, respectively. The capture depth data were standardized relative to the chart datum using the free software WXTide32, version 4.7 (Free Software Foundation Inc. 2007). All analyses were performed with the free software R (R Core Team, 2014), version 3.1.0, using a significance value of α=0.05 and following the relevant statistical assumptions (Zar, 1999).
RESULTS
Density, size and depth
A total of 2,650 individuals of D. mexicanum were counted, which resulted in an average reef density of 8.28±11.65 ind/m2. Population densities differed significantly between reef zones, with FR showing the highest average density (13.0±11.53 ind/m2) followed by PL (6.73±8.46 ind/m2) and TR (5.11±13.38 ind/m2) (Fig. 2A). Although density was lowest in TR, the largest number of individuals (n = 77) in a quadrant was counted in this area. Finally, the dispersion index (I) showed that D. mexicanum depicts an aggregated distribution pattern.
Size was measured in a total of 860 individuals, of which 45.7% corresponded to individuals of size M, 40.6% to G and 13.7% to P. The mean size of the sea-urchins was 19.62±5.02 mm, with a maximum of 41.5 mm and a minimum of 9.0 mm. There were significant differences in size among all reef zones, with TR being the zone with the greatest average sized individuals (22.86±5.62 mm) followed by PL (19.80±4.98 mm) and FR (17.86±3.75 mm) (Fig. 2B). The sizes were inversely related to the density; i.e., the area with the highest density (FR) had the smallest individuals and vice versa (Fig. 2B). Finally, the mean depth at which the echinoid measurements were performed gradually increased from the coast to the sea as follows: TR: 1.49 m, PL: 1.83 m and FR: 3.62 m.
Bioerosion rate
The calculation of the bioerosion rate was performed with 81 of the 90 collected sea-urchins, due to possible errors during the calcination and digestion of the samples. The size of sea-urchins significantly affected bioerosion rate; size G individuals removed larger volumes than the M and P individuals (Fig. 3A). There was also a significant effect of the reef zone on the bioerosion rate, but in this case, only marginal differences were found between FR and TR . The highest rate was in the FR followed by PL and TR (Fig. 3B). Furthermore, the size of the echinoids (Fig. 4A) and collection depth (Fig. 4B) also affected the content of CaCO3. Finally, the average annual rate of bioerosion by D. mexicanum on La Azufrada reef, considering the relative abundances of each size class in each reef zone, was (Table 1).
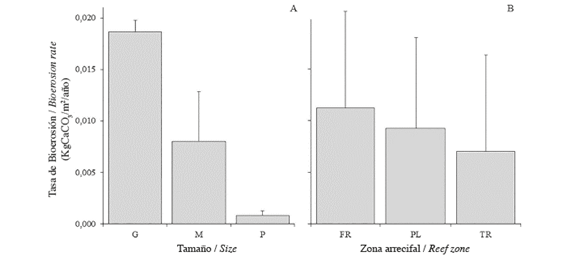
Figure 3 Average rate of bioerosion (kg CaCO3/m2/yr±SD) by Diadema mexicanum by size class (A) and reef zone (B) on La Azufrada reef, Gorgona Island, Colombian Pacific.
DISCUSSION
It is important to know the population density of echinoids in marine ecosystems, such as coral reefs, not only because it directly affects the bioerosion rate (i.e., higher densities result in higher rates of bioerosion) but because echinoids help modulate the relative abundances and composition of algae and coral species (Herrera- Escalante et al., 2005). In this study, the population densities of D. mexicanum on La Azufrada were relatively high compared to other areas of the Tropical Eastern Pacific (Table 1); higher values have only been found in localities (e.g., Uva Island, Panama) affected by anomalous events (e.g., El Niño) (Eakin, 1992). Under such conditions, the high densities of echinoids are the final response to a series of changes caused by higher water temperatures including increased coral mortality, rapid algal growth on the coral skeletons and, finally, an increase in the abundance of the echinoids that feed on them (Glynn, 1988; Toro-Farmer et al., 2002).
During the study period, large patches of dead coral were observed, which possibly resulted from anomalous temperature events in the region (NOAA, 2016). Extreme low tides were also recorded during the same period (IDEAM, 2015). These types of events probably triggered the process of coral mortality with the concomitant increase in the densities of echinoids. In addition, echinoid densities are known to be related to individual size and substrate complexity (Londoño et al., 2003; Lee, 2006); that is, the relationship between the number and size of refuges determines both the abundance and size of the echinoids that can colonize an area (Lozano-Cortés et al., 2011). In this study, the FR echinoids were smaller but more abundant than those of TR, which may be indicative of the structural complexity of these two areas. Although this characteristic was not measured, field observations suggest that the refuges in the TR are larger but less abundant than in FR, which would accommodate animals with larger average sizes but smaller abundances, as observed in the present study. In addition, it has been shown that there is an inversely proportional relationship between coral cover and the abundance of echinoids (Glynn et al., 1979; Bak, 1990), which could explain the high abundance of D. mexicanum in FR compared to PL, which has greater coral cover (Zapata, 2001). Nevertheless, it is likely that no single factor (e.g., coral cover or refuge size) modulates both the size structure and the population density of a particular species in the different zones of a complex ecosystem, such as a coral reef; instead, several factors (biotic and abiotic) combine to determine the individual and population characteristics of organisms found in a particular subdivision (e.g., TR, PL, FR) of an ecosystem.
Three primary and significant factors have been suggested to influence the erosive effect of echinoids on coral reefs: species, individual size and density (Bak, 1994). The results of the present study indicate the effect of echinoid size on the amount of material removed from the reef by individuals per unit area and time (i.e., the rate of bioerosion), which is consistent with results reported by other authors for different echinoid species: Echinometra mathaei (McClanahan and Muthiga, 1989), Centrostephanus coronatus (Toro-Farmer et al., 2002), D. mexicanum (Herrera-Escalante et al., 2005; Alvarado et al., 2012) and D. setosum (Dumont et al., 2013) or echinoids in general (Bak, 1990; Carreiro-Silva and McClanahan, 2001). Such results are not surprising, but it is worth noting, as mentioned above, that size is only one factor in the equation, so investigating its effect combined with those of other factors (e.g., the reef zone) is an interesting challenge. In this sense, the impact caused by echinoids of a certain size can be modulated by their density in each zone or on the reef in general. It seems plausible to think that small individuals at high densities (e.g., as observed in FR) may have a similar impact as large individuals at low densities (e.g., as observed in TR), resulting in similar rates of bioerosion across the different reef zones.
To clearly understand the true bioerosive effect of echinoids, obtaining overall averages is not enough; it is necessary to consider both size and specific density. Although echinoid densities on La Azufrada reef are high, mean sizes are relatively small, so the values of CaCO3 removal are within the range reported for both the Colombian Pacific (Lozano-Cortés, et al., 2011) and the Tropical Eastern Pacific (Mexico - Herrera-Escalante et al., 2005; Costa Rica - Alvarado et al., 2012).
When determining rates of bioerosion, by any type of organism, an important parameter to take into account, is the time of intestinal turnover. Some authors (Glynn et al., 1979; Bak, 1990; Toro-Farmar et al., 2002) have calculated 24-h, but others have shown that they may be longer (1.18 days for Diadema setosum - Carreiro-Silva and McClanahan, 2001) or shorter (0.5 days for Echinometra vanbrunti - Lozano-Cortés et al., 2011). Therefore, it is important that future studies estimate species-specific turnover times because results could vary amply depending on this value.
In contrast, depth, in biological terms, does not significantly influence the average rate of bioerosion, mainly because the depth amplitudes encountered in this study were small (between approximately 1 and 7 m). In addition, other authors have considered depth to be a secondary factor in nearby reefs (Londoño-Cruz et al., 2003) due to the lack of a marked depth gradient and the relatively wide (±4 m) tidal range in the zone, which leads to wide-ranging, small-scale variation that could affect the zonation of erosion-producing organisms. Although this “instability” could have an insignificant effect on echinoids, detailed studies are necessary to test this hypothesis. In this sense, some studies have found a significant effect of depth on bioerosion (Glynn and Leyte-Morales, 1997; Griffin et al., 2003; Herrera-Escalante et al., 2005), but the depth range was larger in those studies (between 1 and 13 m).
Finally, some studies have shown that the rate of CaCO3 production by pociloporid corals on La Azufrada reef can be as much as three orders of magnitude higher (5.56 kg CaCO3/m /yr - Palacios et al., 2014) than the bioerosion values recorded for D. mexicanum (0.083 kg CaCO3/m /yr) in the present study. Therefore, the bioerosive effect of this echinoid may not have a significant effect on the stability of the reef. It is necessary to emphasize, however, that both the calculations by Palacios et al. (2014) and those reported here constitute only two components of the balance between accretion and bioerosion. To elucidate the bioerosive effect, it is necessary to consider the contribution of other coral species (e.g., massive species) and coralline algae in the process of accretion and the contributions of other organisms, both borers (e.g., sponges, worms, crustaceans and bivalves) and grazers (e.g., fish and other echinoids), in the process of bioerosion.
ACKNOWLEDGEMENTS
The authors thank the Universidad del Valle for funding the study, which was carried out under the “Information Center 7970” project. We also thank the Natural National Parks Unit and especially the officials of the Gorgona Island Natural National Park for their assistance in the field. Finally, we thank Juan F. Lazarus, Wilmar Torres and José Tavera for their advice on the statistical analysis, Juan Carlos Mejía for drafting and designing the map, and the members of the LITHOS Group for assistance during the writing of the manuscript.
REFERENCES
Alvarado, J.J., J. Cortés and H. Reyes-Bonilla . 2012. Reconstruction of Diadema mexicanum bioerosion impact on three Costa Rican Pacific coral reefs. Rev. Biol. Trop., 60: 121-132. [ Links ]
Bak, R.P.M . 1990. Patterns of echinoid bioerosion in two Pacific coral reef lagoons. Mar. Ecol. Prog. Ser., 66: 267-272. [ Links ]
Bak, R.P.M. 1994. Sea urchin bioerosion on coral reefs: Place in the carbonate Budget and relevant variables. Coral Reefs, 13: 99-103. [ Links ]
Barberá, C, D. Fernández-Jover, J.A., López Jiménez, D. González Silvera, H. Hinz and J. Moranta. 2011. Trophic ecology of the sea urchin Spatangus purpureus elucidates from gonad fatty acids composition analysis. Mar. Environ. Res., 71: 235-246. [ Links ]
Carreiro-Silva, M. and T.R. McClanahan. 2001. Echinoid bioerosion and herbivory on Kenya coral reefs: the role of protection from fishing. J. Exp. Mar. Biol. Ecol., 262: 133-153. [ Links ]
Downing, N. and C.R. El-Zahr . 1987. Gut evacuation and filling rates in the rock-boring sea urchin, Echinometra mathaei. Bull. Mar. Sci., 41(2): 579-584. [ Links ]
Dumont, C.P., D. Lua, J.C. Astudillo, K. Fong, S. Chak and J.W. Qiu . 2013. Coral bioerosión by the sea urchin Diadema setosum in Hong Kong: Susceptibility of different coral species. J. Exp. Mar. Biol. Ecol., 441: 71-79. [ Links ]
Eakin, C.M. 1992. Post-El Niño Panamanian reefs: Less accretion, more erosion and damselfish protection. Proc. 7th Int. Coral Reef Symp. Guam 1:387-396. [ Links ]
Edgington, E.S. and P. Onghena. 2007. Randomization tests. Chapman and Hall/CRC, 4th Edition. Boca Raton, USA. 338 p. [ Links ]
Elliot J.M. 1977. Some methods for the statistical analysis of sample benthic invertebrates. Segunda Edic. Freshwater Biol. Assoc. Ambleside, UK. 156 p. [ Links ]
Free Software Foundation, Inc. 2007. WXTide32, ver. 4.7. http://www.wxtide32.com/download.html . 16/05/2016. [ Links ]
Glynn, PW. 1988. El Niño warming, coral mortality and reef framework destruction by echinoid bioerosion in the Eastern Pacific. Galaxea, 7: 129-160. [ Links ]
Glynn, P. W. and G.E. Leyte-Morales. 1997. Coral reefs of Huatulco, west México: reef development in upwelling Gulf of Tehuantepec. Rev. Biol. Trop., 45(3): 1033-1047. [ Links ]
Glynn, P.W., G.M. Wellington and C. Birkeland. 1979. Coral reef growth in the Galapagos: Limitation by sea urchins. Science, New Series, 203(4375): 47-49. [ Links ]
Griffin, S.P., R.P. García and E. Weil. 2003. Bioerosion in coral reef communities in southwest Puerto Rico by the sea urchin Echinometra viridis. Mar. Biol., 143: 79-84. [ Links ]
Guzmán, H.M. and J. Cortés. 1992. Cocos Island (Pacific of Costa Rica) coral reefs after the 1982-83 El Niño disturbance. Rev. Biol. Trop., 40: 309-324. [ Links ]
Hernández-Ballesteros, L., E. Elizalde-Rendón, J. Carballo and J. Carricart. 2013. Sponge bioerosion on reef-building corals: Dependent on the environment or on skeletal density? J. Exp. Mar. Biol. Ecol., 441: 23-27. [ Links ]
Herrera-Escalante, T., R. López-Pérez and G. Leyte-Morales. 2005. Bioerosion caused by the sea urchin Diadema mexicanum (Echinodermata: Echinoidea) at Bahías de Huatulco, western México. Rev. Biol. Trop., 53: 263-273. [ Links ]
Hoegh-Guldberg, O., P.J. Mumby, A.J. Hooten, R.S. Steneck, P Greenfield, E. Gómez, C.D. Harvell, P.F. Sale, A.J. Edwards, K. Caldeira, N. Knowlton, C.M. Eakin, R. Iglesias-Prieto, N. Muthiga, R.H. Bradbury, A. Dubi and M.E. Hatziolos . 2007. Coral reefs under rapid climate change and ocean acidification. Science, 318: 1737-1742. [ Links ]
IDEAM. 2015. Pronóstico de pleamares y bajamares en la costa Pacífica colombiana. IDEAM. Bogotá. 177 p. [ Links ]
Lawrence, J.M. 1975. On the relationships between marine plants and sea-urchins. Oceanogr. Mar. Biol. An. Rev., 13: 213-286. [ Links ]
Lawrence, J.M. 2007. Edible sea urchins: biology and ecology. Develop. Aquac. Fish. Sci., 37, 529 p. [ Links ]
Lee, S.C. 2006. Habitat complexity and consumer-mediated positive feedbacks on a Caribbean coral reef. Oikos, 112: 442-447. [ Links ]
Londoño-Cruz, E., J. Cantera, G. Toro-Farmer and C. Orozco . 2003. Internal bioerosion by macroborers in Pocillopora spp. in the tropical Eastern Pacific. Mar. Ecol. Prog. Ser., 265: 289-295. [ Links ]
Lozano-Cortés, D., E. Londoño-Cruz y F. Zapata. 2011. Bioerosión de sustrato rocoso por erizos en bahía Málaga (Colombia), Pacífico Tropical. Rev. Cienc., 15: 9-22. [ Links ]
McClanahan T. and N. Muthiga . 1989. Patterns of predation on a sea urchin, Echinometra mathaei (de Blainville), on Kenya coral reefs. J. Exp. Mar. Biol. Ecol., 126: 77-94. [ Links ]
Morrison, D. 1988. Comparing fish and urchin grazing in shallow and deeper coral reef algal communities. Ecology, 69: 1367-1382. [ Links ]
Neira, R. y J. Cantera. 2005. Composición taxonómica y distribución de las asociaciones de equinodermos en los ecosistemas litorales del Pacífico colombiano. Rev. Biol. Trop., 53: 195-206. [ Links ]
Neumann, A.C. 1966. Observations on coastal bioerosion in Bermuda and measurements of the boring rate of the sponge Cliona lampa. Limnol. Oceanogr., 11: 92-108. [ Links ]
NOAA. 2016. National Centers for Environmental Information, State of the Climate: Global Climate Report for Annual 2015. https://www.ncdc.noaa.gov/sotc/global/201513. [ Links ]
Nodarse, A. 2001. Abundancia y distribución del erizo Echinometra lucunter (Linnaeus) (Echinodermata, Echinoidea) en un arrecife del litoral norte de ciudad de la Habana. Rev. Invest. Mar., 22: 107-115. [ Links ]
Palacios, M.M., C.G. Muñoz and F.A. Zapata. 2014. Fish corallivory on a pocilloporid reef and experimental coral responses to predation. Coral Reefs, 33:625-636. [ Links ]
Prahl, H.v., F. Guhl y M. Grögl. 1979. Gorgona. Futura, Bogotá. 279 p. [ Links ]
R Core Team. 2014. R: A language and environment for statistical computing. R Foundation for Statistical Computing, Viena. URL URL http://www.R-project.org/ (18/05/2016). [ Links ]
Sammarco, P.W. 1982. Echinoid grazing as a structuring force in coral communities: whole reef manipulations. J. Exp. Mar. Biol. Ecol., 61: 31-35. [ Links ]
Sánchez-Jerez, P., A. Cesar, F. Cortez, C. Pereira y S. Silva. 2001. Distribución espacial de las poblaciones más abundantes de erizos de la costa sudeste del litoral de São Paulo (Brasil). Cienc. Mar., 27(1): 139-153. [ Links ]
Toro-Farmer, G., J. Cantera, E. Londoño-Cruz, C. Orozco y R. Neira. 2002. Patrones de distribución y tasas de bioerosión del erizo Centrostephanus coronatus (Diadematoida: Diadematidae), en el arrecife de Playa Blanca, Pacífico colombiano. Rev. Biol. Trop., 52(1): 67-76. [ Links ]
Tribollet, A. and S. Golubic . 2011. Reef bioerosion: agents and processes: 117-134. En Dubinsky, Z. y N. Stambler (Eds.). Coral Reefs : An Ecosystem in Transition. Springer. Dordrecht, Germany. 541 p. [ Links ]
Vargas-Ángel, B. 2003. Coral community structure off the Pacific coast of Colombia: Onshore vs offshore coral reef. Atoll. Res. Bull., 499: 1-21. [ Links ]
Zapata, F.A. 2001. Formaciones coralinas de Isla Gorgona: 27-40. En Barrios, L.M. y M. López-Victoria (Ed.). Gorgona marina: contribución al conocimiento de una isla única. INVEMAR, Ser. Publ. Espec. 7, 160 p. [ Links ]
Zapata, F. and B. Vargas-Ángel . 2003. Corals and coral reefs of the Pacific coast of Colombia. 419-447. En Cortés, J. (Ed.) Latin America coral reefs. Elsevier Science B. V. Amsterdam. 497 p. [ Links ]
Zapata, F.A., A. Rodríguez-Ramírez, C. Caro-Zambrano and J. Garzón-Ferreira. 2010. Mid-term coral-algal dynamics and conservation status of a Gorgona Island (Tropical Eastern Pacific) coral reef. Rev. Biol. Trop., 58: 81-94. [ Links ]
Zar, J.H. 1999. Biostatistical analysis. Cuarta Edic. Prentice Hall. New Jersey. 663 p. [ Links ]
Received: March 24, 2017; Accepted: August 10, 2017