Services on Demand
Journal
Article
Indicators
-
Cited by SciELO
-
Access statistics
Related links
-
Cited by Google
-
Similars in SciELO
-
Similars in Google
Share
Boletín de Investigaciones Marinas y Costeras - INVEMAR
Print version ISSN 0122-9761
Bol. Invest. Mar. Cost. vol.47 no.2 Santa Marta July/Dec. 2018
https://doi.org/10.25268/bimc.invemar.2018.47.2.750
Research Articles
Abundance and distribution of the surface zooplankton of the coastal zone Arapito - Santa Fe, Sucre state, Venezuela
1 Lab. Zooplancton. Dpto. Biología Marina, Instituto Oceanográfico de Venezuela, Universidad de Oriente, Sucre - Venezuela. bmarquez2001@gmail.com.
2 Instituto de Investigaciones Científicas - Universidad de Oriente, Núcleo Nueva Esparta, Cumaná, Sucre - Venezuela. luis.troccoli@gmail.com
3 Lab. Ictioplancton. Dpto. Biología Marina, Instituto Oceanográfico de Venezuela, Universidad de Oriente, Cumaná, Sucre - Venezuela. baumarm@gmail.com
4 Lab. Fitoplancton. Dpto. Biología Marina, Instituto Oceanográfico de Venezuela, Universidad de Oriente, Cumaná, Sucre - Venezuela. jrdiazramos@ yahoo.com
5 Facultad de Ciencias del Mar, Universidad Estatal Península de Santa Elena, Ecuador
The study of zooplankton in the coastal zone is fundamental to understand ecological processes. The coastal zone Arapito - Santa Fe, located in the Mochima National Park, Sucre state, Venezuela, is considered an area of great natural importance and therefore the study on zooplankton was carried upon. For this reason, the composition and community structure of zooplankton were determined in 20 stations, separated in a) coastal stations and b) away from the coast during December 2008. Samples were collected obliquely by dragging a Bongo net (500 and 300 μm, with a flow meter). The community was characterized by the presence of holoplanktonic organisms and a lower proportion of meroplankton. The main holoplankton groups were cladocerans, copepods and gelatinous plankton (appendiculate, siphonophores and hydromedusae). The most abundant species were the cladocerans Penilia avirostris and Pseudevadne tergestina and the copepods Acartia tonsa and Temora turbinata. The high percentage of holoplankton in the study area let it to be considered as a growth and feeding zone. The AMOSIM-MDS showed significant differences (p <0.05) between the two environments with R = 0.27 but with low dissimilarity (17.4%), which suggests that mixing processes promote homogeneous distribution of zooplankton in the study area.
KEY WORDS: Abundance; Copepods; Holoplankton; Meroplankton; Mochima National Park
El estudio del zooplancton en la zona costera es fundamental para entender los procesos ecológicos. La zona costera Arapito - Santa Fé, ubicada en el Parque Nacional Mochima, estado Sucre, Venezuela, es considerada un área de gran importancia natural y por ello se planteó el estudio sobre el zooplancton. En este sentido, se determinó la composición y estructura comunitaria del zooplancton, en 20 estaciones, separadas en a) estaciones costeras y b) alejadas de la costa, durante diciembre 2008. Las muestras fueron recolectadas arrastrando oblicuamente una red de Bongo (500 y 300 μm, con flujómetro). La comunidad estuvo caracterizada por la presencia de organismos holoplanctónicos y en menor proporción de meroplancton. Los principales grupos holoplanctónicos fueron cladóceros, copépodos y plancton gelatinoso (apendiculados, sifonóforos e hidromedusas). Las especies más abundantes fueron los cladóceros Penilia avirostris y Pseudevadne tergestina y los copépodos Acartia tonsa y Temora turbinata. El alto porcentaje de holoplancton en la zona de estudio permite considerarla como una zona de crecimiento y alimentación. El ANOSIM-MDS, mostró diferencias significativas (p<0.05) entre los dos ambientes con R=0.27 pero con baja disimilitud (17.4%), lo que sugiere que procesos de mezcla promueven la distribución homogénea del zooplancton en la zona de estudio.
PALABRAS CLAVE: Abundancia; Copépodos; Holoplancton; Meroplancton; Parque Nacional Mochima
INTRODUCTION
Zooplankton includes species from practically every animal group found in the marine pelagic ecosystem (Kiørboe, 2008), and these animals can effectively control the abundance pattern of phytoplankton and constitute an important food source for different organisms of plankton, nekton, and marine birds. Therefore, zooplankton represents a component of biomass and fundamental biological diversity in the trophodynamics of the pelagic ecosystem, thereby transferring a significant part of the energy fixed by the primary producers to higher trophic levels (D’Alelio et al., 2016; Heneghan et al., 2016) and playing an important role in biogeochemical cycles (RaphaëlN’doua et al., 2009; Jose et al., 2015). Pulses of the hydrographic regime can affect the zooplanktonic community because the nutrient load first impacts the phytoplankton and then produces response patterns in the zooplankton (Liess et al., 2016) and fish population density (Selleslagh and Amara, 2008) because zooplanktonic organisms tend to present relatively short life cycles (Litchman et al., 2013). Thus, understanding changes in the structure of the zooplankton community as a function of environmental variability at different temporal and spatial scales is fundamental for the description of the functions and biological productivity of marine pelagic ecosystems, and changes in the zooplankton community can be used to monitor aquatic ecosystems (Smitha et al., 2013; Bork et al., 2015).
The Venezuelan eastern coastal zone is considered important because of its biological diversity and fisheries (Márquez and Jiménez, 2002; Márquez- Rojas et al., 2006a; Allen et al., 2007) as well as tourism because the busiest beaches of the region are located in the area. It is also a coastal upwelling zone (Okuda, 1975; Alvera-Azcárate et al., 2009; Rueda- Roa and Muller-Karger, 2013). Studies on the specific composition, abundance and spatiotemporal distribution of zooplankton are available for various environments of the northeastern coast, such as the Mochima Bay (Márquez et al., 2007, 2008; Marcano et al., 2010), the Cariaco Basin (Márquez et al., 2009) and the Gulf of Cariaco (Legaré, 1961; Zoppi 1961; Marín et al., 2004; Márquez et al., 2006b; 2011). However, the information available for the Arapito-Santa Fe coast is scarce. This coastal axis is subject to the influence of hydrographic conditions, especially the intermittent coastal upwelling (Menge and Menge, 2013).
Therefore, this study proposed to determine the composition, abundance, biomass and spatial distribution of zooplankton in the coastal area of Arapito-Santa Fé in Sucre State during December 2008.
MATERIALS AND METHODS
Study area
The study area is situated in the marine-coastal region between Playa Arapito and the town of Santa Fe (10°24’N, 64°40’W; Figure 1), which is located in Sucre State, Venezuela, along with the SE Caribbean Sea. The area is an approximately 12 km long and presents sandy beaches, a highly rugged coastline, and a very short continental shelf, which extends offshore from 40 m to 80 m in depth. Similar to northeastern Venezuela, this area is affected by the phenomenon of seasonal coastal upwelling (Okuda, 1975; Alvera-Azcárate et al., 2009; Scranton et al., 2014), which is closely related to the regime of the trade winds, and three climatic periods with different upwelling magnitudes are observed during the year. The first, is a period between December and April, and it presents strong wind intensity (upwelling season) accompanied by low temperature and dissolved oxygen concentrations and high nutrient concentrations; the second, is between May and July (transition period), and it presents a moderate wind intensity and subtropical water; and the third, is between August and November, and it presents the lowest wind intensity (relaxation period), high temperatures and dissolved oxygen concentrations and low nutrient concentrations (Lorenzoni et al., 2013).
Fieldwork
Sampling was carried out during the survey of the B/O GUAIQUERI II on December 06 and 07, 2008. The stations were located between the coastal areas of the entrance of the Gulf of Santa Fe and the Arapo Islands, which present strong bathymetric gradients. These stations were established in protected areas as zones of a special protection regime (ABRAE) and are part of the Mochima National Park. Samples were collected at 20 stations, which were divided into coastal stations on the continental shelf (stations 3, 5, 9, 12, 14, 20, 23, 26, 28 and 31) and offshore stations in the open sea (greater than 1 km, stations 34, 36, 37, 38, 39, 40, 41, 44, 46 and 49) (Figure 1).
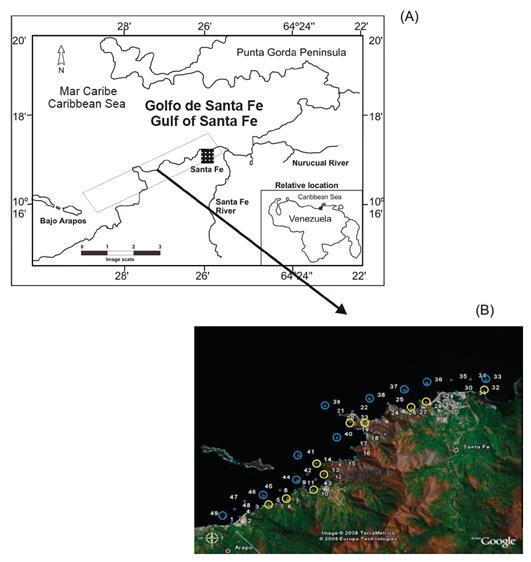
Figure 1 (A) Geographical location of the study area. (B) Location of the sampling stations in the coastal area of Arapito-Santa Fe, Sucre State, Venezuela. The yellow circles represent the coastal stations, and the blue circles represent the offshore stations.
A Bongo mesh net with a pore size of 500 and 300 μm and a mouth diameter of 56 cm was used, and it was equipped with a Rigosha flowmeter. At the coastal stations, daily oblique hauls were performed from the bottom towards the surface; and at the open sea stations, daily oblique hauls were performed from 50 m below the surface to the surface for 15 min at a hauling speed of two knots. The samples were fixed in 5% buffered formalin and transferred to the zooplankton laboratory at the Oceanographic Institute of Venezuela. Abundance was determined by extrapolating the analyzed volume to the original sample. The filtered volume was calculated based on the flowmeter data, which served as the basis for estimating the mesozooplankton abundance per cubic meter of filtered water. To establish the microalgal abundance, samples were taken in each station using Niskin bottles at a depth of 1 m, and they were then poured into plastic bottles (250 ml) and fixed with 4% formalin neutralized with hexamethylenetetramine.
Physical-chemical analysis of water
Water samples were collected through a conductivity-temperature-depth (CTD) rosette (SBE-19) using 5 L Niskin bottles set at a 5 m depth. The samples (500 ml) were kept frozen (-20°C) until processing and analysis at the Laboratory of Nutrient Elements, Department of Oceanography, Oceanographic Institute of Venezuela.
The dissolved oxygen was determined according to the Winkler method described by Strickland and Parsons (1972). The CTD (SBE-19) was used for the vertical recording of temperature and salinity. The concentration of phosphates and nitrates was established according to the recommendations of Gordon et al. (1993). The results for these elements were expressed in μmol L-1.
Determination of chlorophyll a (Chl a) on the surface was performed using the spectrophotometric method described by Lorenzen and modified by Strickland and Parsons (1972). For this purpose, samples were collected in dark polyethylene bottles (2 L).
Analysis of phytoplankton and zooplankton
Microalgae were quantified by the sedimentation method of Utermöhl (1958) using columns of 50 ml. Subsequently, Lugol drops were added to facilitate cell sedimentation and better assess their structures. The microalgae were identified based on the studies of Cupp (1943), Thomas (1997), Berard-Therriault et al. (1999) and Rivera-Tenembaum (2006). Heterotrophic dinoflagellates (e.g., Protoperidinium) were not tallied.
For the zooplankton, each 300 μm mesh sample was homogenized and divided into two equal portions using a Folsom’s Plankton Sample Divider. One part was designated for the study of the community structure and the other for the biomass analysis through the gravimetric method (wet biomass, Postel et al., 2000), without separating the gelatinous organisms. The data were expressed in mg m-3. For the taxonomic determination of the concentrated samples (500 ml), 2.5 ml subsamples were taken with a Stempel pipette. Counting and identification were performed using the works of Tregouboff and Rose (1957), Zoppi (1971), Campos-Hernández and Suárez-Morales (1994) and Boltovskoy (1999), as well as the database of Razouls et al. (2005-2017). For some groups, the most important species were highlighted.
Data analysis
Statistical tests were performed using the software Statgraphics plus 5.1. The Kolmogorov-Smirnov normality and Bartlett’s homoscedasticity tests (Sheskin, 2004) did not meet the assumptions of the parametric analysis. Therefore, the abundance of phytoplankton, biomass and total zooplankton abundance were compared between the coastal and offshore stations using the nonparametric Mann-Whitney test (Wilcoxson Test). A significance level of alpha = 0.05 was considered.
To establish whether there were significant differences in the community structure among the main zooplankton groups, an analysis of similarities (ANOSIM) and non-metric multidimensional scaling (MDS) were performed. The latter was used to visualize the ordination of the zooplankton groups in two dimensions relative to the station location by testing the differences between the groups of the zooplankton community according to the station locations. The abundance data were transformed to the fourth root to create a Bray-Curtis similarity matrix for the analyses. The identification of the main taxonomic groups that contributed the most to the similarity and dissimilarity between the station locations was carried out with the similarity percentage analysis (SIMPER). These analyses were run in Primer 6 + PERMANOVA (Clarke and Gorley, 2006).
To establish the relationship between environmental variables (temperature, salinity, dissolved oxygen, nitrate, nitrite and phosphate) and phytoplankton abundance and the zooplankton groups of each zone, a redundancy analysis (RDA) was conducted after demonstrating that the distance of the gradient in the first component had a value < 3 (Muylaert et al., 2009).
The surface graphics were produced with Surfer version 8.0 to represent the isolines of the physical-chemical variables.
RESULTS
Environmental variables
The surface water temperature varied from 26.60°C at station 24 to 28.60°C at station 21, with an average of 27.70°C ± 0.43°C (Figure 2A). The minimum salinity value of the surface waters in the study area was 29.40 at station 31, and the maximum was 36.90 at station 13, with an overall average of 36.24 ± 1.60 (Figure2B). The dissolved oxygen varied between 3.89 ml L-1 (station 3) and 4.93 ml L-1 (station 8), with an average of 4.44 ml L-1 ± 0.22 ml L-1 (Figure 2C).
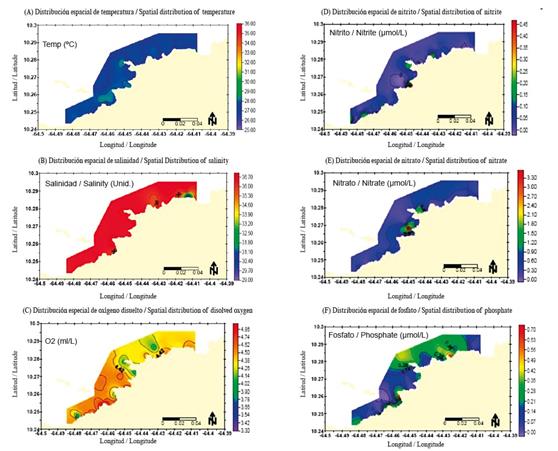
Figure 2 Spatial distribution of (A) temperature (°C); (B) salinity; (C) dissolved oxygen (ml/L); (D) nitrite; (E) nitrate; and (F) phosphate (μmol/L) of the surface waters in the coastal area of Arapito - Santa Fe, Sucre State, Venezuela during December 2008.
The nitrite values varied between non-detectable (ND) and 0.48 μmol L-1, with an overall average of 0.06 μmol L-1 ± 0.08 μmol L-1. The highest values were recorded at stations 16 and 20, near the coast between Arapito and Playa Colorada and the sector of Santa Cruz and Punta Colorada (Figure 2D). The nitrate values were between 0.01 μmol L-1 at station 16 and 3.62 μmol L-1 at station 17, with an average of 0.47 μmol L-1 ± 0.55 μmol L-1. In almost all the regions studied, the nitrate values were lower than 1 μmol L-1 except for the populated areas of Santa Cruz and to the east of Punta Colorada, where they were higher than 1.50 μmol L-1 (Figure 2E). The phosphate concentrations fluctuated between ND and 0.77 μmol L-1, with an average of 0.20 μmol L-1 ± 0.17 μmol L-1. The maximum values were recorded to the east of Playa Colorada and in front of the company Camaronera Aguamarina de la Costa (stations 9 and 13, respectively; Figure 2F).
Biotic variables
The concentrations of Chl a in the area were undetectable. A high abundance of flagellated forms was found (75 - 93% of the total individuals < 10 μm), which could not be identified; the remainder of the community was formed by chlorophytes, diatoms, dinoflagellates, euglenophytes, prymnesiophytes, and silicoflagellates. The samples also presented ciliates, tintinnids, cladocerans, and copepods at different life cycle stages. The abundance ranged between 47 cells ml-1 and 1,177 cells ml-1. The abundance of phytoplankton did not significantly vary concerning the station locations (W Test = 3.65; p > 0.05).
The zooplankton biomass expressed as wet biomass varied between 0.14 mg m-3 and 0.71 mg m-3. The lowest values (< 0.20 mg m-3) were found at the stations located towards the west, i.e., stations 4 and 49, which were far from the populated centers; and the highest values were found in the stations located to the east, i.e., station 34 (0.71 m-3) and 36 (0.64 m-3), which were opposite the town of Santa Fe. No significant differences were found with respect to the station locations (W Test = 0.67; p > 0.05).
The zooplankton abundance varied between 1.226 ind. m-3 and 3.708 ind. m-3 (Table 1). The highest values were recorded at stations 5, 14, 34 and 36 (3.341 ind. m-3- 3,708 ind. m-3), and intermediate values were recorded at stations 23, 28, 31, 39, 40 and 41 (2.266 ind. m-3 - 2.774 ind. m-3) (Figure 3A Table 1). Stations 20 and 37 had the lowest abundance values at < 1,400 ind. m-3 (Figure 3A). The zooplankton abundance did not vary significantly with respect to the station locations (W Test = 0.75; p > 0.05; Figure 3B).
Table 1 Abundance of surface zooplankton (ind.m-3) in the coastal area of Arapito - Santa Fe, Sucre State, Venezuela, during December 2008.
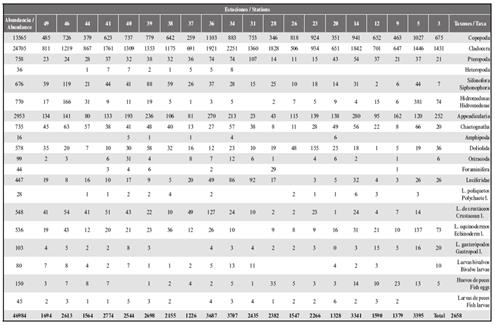
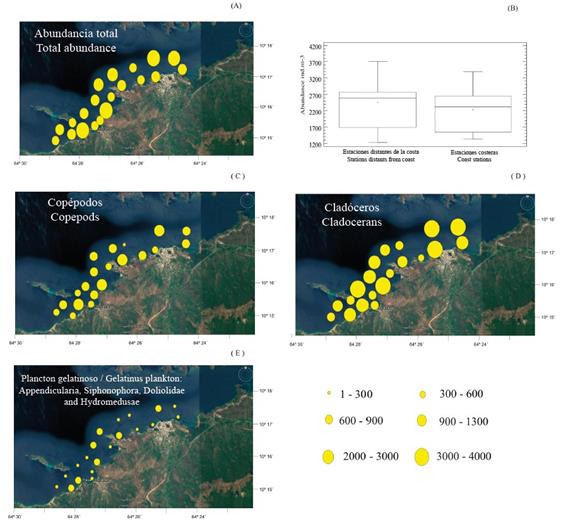
Figure 3 Location of the stations and spatial distribution of total zooplankton abundance (ind. m-3) (A and B); spatial distribution of the principal zooplanktonic groups (ind. m-3), copepods (C), cladocerans (D) and gelatinous plankton (E) in the coastal area of Arapito - Santa Fe, Sucre State, Venezuela, during December 2008.
Twenty taxonomic groups were identified (Table 1). The highest abundance values were recorded in the offshore stations for copepods (coastal, 346 ind. m-3 to 1.027 ind. m-3; offshore, 259 ind. m-3to 1.103 ind. m-3) and cladocerans (coastal, 506 ind. m-3 to 1,842 ind. m-3; offshore, 691 ind. m-3 to 2.251 ind. m-3). However, for both groups, no significant differences in abundance were detected between the station locations (W Test = 0.98 and W Test = 0.75, respectively; p > 0.05; Figure 3C, D).
A similar result was found for the gelatinous zooplankton and the siphonophores because they did not show a marked distribution pattern, whereas the opposite result was observed for the hydromedusae (coastal, 503 ind. m-3; offshore, 267 ind. m-3; Figures 3E, 4A). However, for this last group, no significant differences were found between the abundance values and the station locations (W Test = 0.40; p > 0.05; Figure 4A).
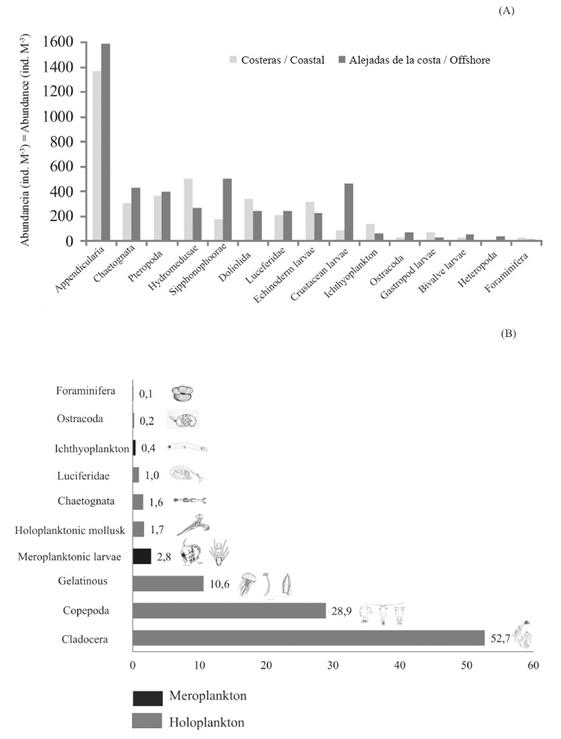
Figure 4 (A) Abundance (ind.m-3) of the zooplanktonic groups considering the coastal and offshore stations; cladocerans and copepods were not considered. (B) Relative abundance of the main zooplankton groups captured in the coastal area of Arapito - Santa Fe, Sucre State, Venezuela. The gray bars represent the holoplankton, and the black bars represent the meroplankton.
Of the total abundance of the taxonomic groups analyzed in both coastal and offshore stations, cladocerans accounted for 52.7% of relative abundance. Copepoda had the second highest relative abundance (28.9%), followed by gelatinous plankton with 10.6% (hydromedusae, appendicularians, siphonophores, and ctenophores). These three groups, which are representatives of holoplankton, had a relative abundance of 92.9% (Figure 10B). The contribution of the meroplanktonic groups in the study area was minimal. Of these groups, the highest abundance corresponded to crustacean larvae (1.17%), followed by echinoderm larvae (1.14%), with the remainder corresponding to mollusks and polychaete larvae (Figure 4B).
Among the most important zooplanktonic groups counted in the Arapito-Santa Fe coastal axis, three species of cladocerans (Penilia avirostris, Pseudevadne tergestina and Evadne spinifera) and 43 species of copepods stood out (among which Acartia tonsa, Temora turbinata and Paracalanus quasimodo) were most abundant. Among the Appendicularia, Oikopleura (Coecaria) longicauda and Oikopleura (Vexillaria) dioica stood out.
The ANOSIM showed significant differences (p < 0.05; global R 0.27) in zooplankton abundance between the coastal and offshore stations.
The SIMPER analysis indicated that the dissimilarity of the community structure between the locations of the stations was low at a percentage of 17.4% (Table 2). The greatest difference in the coastal stations was the absence of Heteropoda, whereas in the offshore stations, the greatest differences were mainly observed among crustacean larvae, bivalve larvae, ostracods, and siphonophores.
Table 2 Percentage of the contribution of the main zooplankton groups that marked the dissimilarity between the station locations analyzed by SIMPER. Dissimilarity: 17.4%.
Grupos / Groups | Abundancia Est. Costeras / Abundance Coastal Stations | Abundancia Est. Alejadas / Abundance Offshore Stations | P. Diss | Porcentaje Contribución Acumulado / Percentage Contribution Accumulated | ||
---|---|---|---|---|---|---|
Heterópodos / Heteropods | 0.00 | 1.11 | 1.85 | 8.08 | 8.08 | |
L. crustáceos / Crustacean larvae | 1.45 | 2.52 | 1.55 | 7.96 | 16.04 | |
L. bivalvos / Bivalve larvae | 0.75 | 1.41 | 1.44 | 6.40 | 29.30 | |
Ostrácodos / Ostracoda | 0.93 | 1.30 | 1.20 | 5.85 | 35.14 | |
Sifonóforos / Sophonophora | 1.93 | 2.59 | 1.46 | 5.13 | 51.16 |
The MDS calculation of the zooplankton abundance based on the station location did not show significant differences between the two zones in which the study was divided; nevertheless, higher similarity could be observed in the offshore stations (Figure 5).
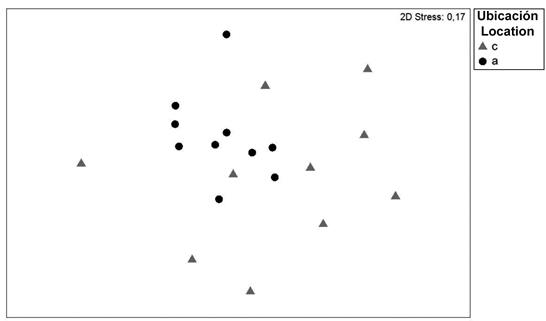
Figure 5 MDS calculation of the stations in the coastal area of Arapito - Santa Fe, Sucre State, Venezuela during December 2008. Coastal stations are represented by gray triangles, and offshore stations are represented by black circles.
The redundancy analysis showed a species- environment correlation of 0.52, and between components 1 and 2, this value was 0.58. In component 1, nitrite was inversely correlated with phytoplankton, siphonophores, crustacean larvae and cladocerans. In component 2, phosphate was inversely correlated with temperature and salinity and chaetognaths, hydromedusae, copepods, and doliolids were positively correlated with phosphate (Figure 6).
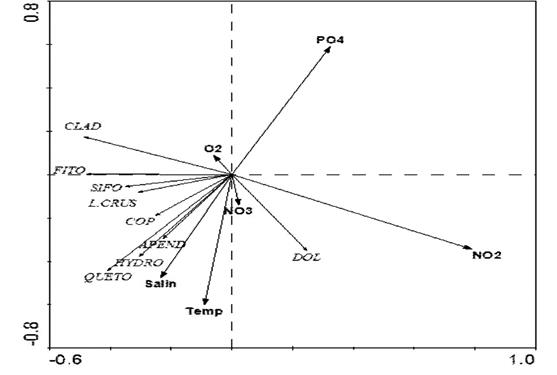
Figure 6 Redundancy analysis (RDA) between the environmental variables and phytoplankton and zooplankton groups of the Arapito - Santa Fe coastal axis, Sucre State, Venezuela. PO4, phosphate; NO2, nitrite; NO3, nitrate; O2, dissolved oxygen; Salin, salinity; Temp, water temperature; Clad, cladocerans, Phyto, phytoplankton; Sipho, siphonophores; Crus. L., crustacean larvae; Cop, copepods; APpend, Appendicularia; Hydro, hydromedusae; Chaeto, Chaetognata; and Dol, doliolids.
DISCUSSION
The oceanographic conditions of the Arapito- Santa Fé coastal zone, as well as the entire northeastern region of Venezuela are affected by the phenomenon of seasonal upwelling (Okuda, 1975; Alvera-Azcárate et al., 2009; Scranton et al., 2014). If the area constituted a zone of thermohaline stability (Rueda-Roa and Muller-Karger, 2013), then marked spatial variations in temperature and salinity should not have been observed during the sampling period. Rather, the differences in temperature and salinity values should have corresponded to temporal differences reflecting the intensity of coastal upwelling in this area, which has been suggested by various authors (Okuda, 1975; Allen et al., 2007; Martínez, 2009).
A comparison of variables recorded in this study with previous research in the area (Okuda, 1975; Allen et al., 2007) for the same season showed lower temperatures reported by the latter (< 27ºC) as well as higher salinity and dissolved oxygen. These differences could be explained by seasonal changes in the intensity of the upwelling mentioned by Taylor et al. (2012), which indicates that in the sampling month of this work, evidence was not observed for a high- intensity upwelling; thus, a delay may have occurred in that particular year.
The nutrient concentrations in this study were relatively higher compared with those reported by Okuda and Benítez (1974) and Okuda (1975), which was related to rainfall in the area and the fluvial contribution, which exceeded the annual average for this area of the country (López-Monroy and Troccoli-Ghinaglia, 2014). The high concentrations of nutrients in the Arapito-Santa Fé marine coast suggest that there are direct anthropogenic contributions as well as continental discharges caused by increased runoff and river flows that enrich the surface waters of the coastal marine regions (Okuda, 1975; Martínez, 2009). No spatial differences were found, indicating that local changes in phosphate and nitrate concentrations that occur through the biochemical reactions in the study area were relatively small. Okuda (1975) mentioned that these high nutrient concentrations persisted in most of the water column for a prolonged period of the year. This natural advantage is exploited by the first link in the trophic chain, which is reflected in high primary productivity and results in an increase in zooplankton abundance, which in turn leads to productive fisheries in the area (Rueda-Roa et al., 2017).
Similar to the physical-chemical variables, the phytoplankton biomass showed marked seasonal variations in northeastern Venezuela associated with the upwelling events that occur between December and July (Okuda, 1975; Alvera-Azcárate et al., 2009; Scranton et al., 2014). The fact that the concentration of Chl a was not detectable in all seasons can be explained because the percentage of nanoflagellates quantified containing chlorophyll was not determined. According to Moustaka- Gouni et al. (2016), nanoflagellates are cells with various methodsofusingenergy(photosynthesizers, heterotrophs, and mixotrophs); therefore, they can proliferate in waters with variable nutrient concentrations. In addition, the samples contained zooplankton organisms that do not normally appear in small volume samples, such as those used to evaluate phytoplankton. This finding suggests that herbivores consume the larger cells (diatoms and dinoflagellates), with higher pigment content, which likely explains the differences regarding the results obtained by Estrella-López (2007), who used samples of 1000 ml or smaller and reported a biomass interval of 0.14 to < 1 mg Chl a m-3 for the Gulf of Santa Fe from July to December in 1999.
Overall, the zooplankton composition of the Arapito-Santa Fé coastal axis reflects the dominance of cladocerans and copepods, followed by gelatinous plankton. Cladocerans stood out among the marine zooplankton mainly because of their abundance, especially P. avirostris over P. tergestina. These two species represented up to 52% of the total zooplanktonic abundance.
Cladocerans are a predominant group of species from continental waters. However, the marine representatives constitute an important part of the zooplankton community and the production of organic matter (Marazzo and Valentin, 2004). P. avirostris is considered typical of warm waters and euryhaline coastal environments (Marazzo and Valentin, 2000), and it is also the only marine cladoceran species that feeds by filtration (Atienza et al., 2008). P. tergestina is a carnivorous species found mainly on the surface of coastal seas and reservoirs (Marazzo and Valentin, 2004), and when it is abundant, it is consumed by fish and other zooplankton predators (Fofonoff, 1994). Moreover, this species can reach great abundances because the parthenogenetic eggs develop into embryos before being released into the environment (paedogenesis) (Marazzo and Valentin, 2000).
The dominance of the cladocerans in the zooplanktonic community could have been due to the aforementioned physical processes because both species are typical of warm waters. P. avirostris was the most abundant and is optimally found in water with temperatures around 25°C (Onbé, 1999), although it can be found at temperatures as low as 18°C, which occurs in the coastal areas and bays of Mexico (Hernández-Trujillo et al., 2010). P. tergestina is considered an indicator of coastal warm water masses, and the thermal interval in which it is optimally distributed is 22 to 25°C (Marazzo and Valentin, 2001). The thermal conditions of the water during this study were within this range, which is recognized as optimal for both species and thus can explain the high concentrations observed.
During a two-year study of the interior of Mochima Bay, Márquez et al. (2008) reported that cladocerans were the most abundant group of zooplankton. Zoppi (1961) stated that cladocerans can compete with copepods in numerical abundance in many areas, particularly in stations near coasts and during calm months. These observations are not consistent with the results obtained here because no spatial differences were found in terms of abundance, and the differences in study results were likely generated by the high variability involved with the long duration of these studies.
A high abundance of the copepods A. tonsa, T. turbinata and P. quasimodo was observed. The presence of these species coincides with the background of dominant copepods in the northeastern part of the country (Zoppi, 1961; Legaré, 1961; Marín et al., 2004; Márquez et al., 2007, 2008, 2011) as well as the Caribbean Sea, southeast Caribbean (Michael and Foyo, 1976) and southern Gulf of Mexico (Álvarez-Cadena et al., 2007; Ruíz-Pineda et al., 2016). A. tonsa has been reported to present a wide tolerance range for salinity and temperature (Martínez- Barragán et al., 2009), and it is also an efficient consumer of phytoplankton, and its populations can increase rapidly in the presence of abundant food (Teixeira et al., 2010). Therefore, the high abundance of the filter feeders P. avirostris and A. tonsa could explain the low concentrations of Chl a.
The zooplankton abundance recorded for the Arapito-Santa Fé coast during this study was higher in comparison to values obtained in other regions of the northeast of the country for similar periods. Márquez et al. (2011) recorded values between 38 and 413 ind.m-3 for December 2003 for the Gulf of Cariaco, and Márquez et al. (2007, 2008) found values of 1210 ind.m-3 for Mochima Bay. These differences are likely due to the seasonality of the coastal upwelling and wind intensity, which has also been suggested by Rueda-Roa and Muller-Karger (2013) based on fluctuations in the biotic and abiotic parameters over several years of study in northeastern Venezuela. These findings reinforce the need to establish long-term studies.
Each species has its own niche and therefore a differential influence on the overall planktonic community. However, the basic premise is that the numerically (or biomass)-dominant species would exert greater influence on the transfer of energy to higher trophic levels, thus constituting important biomass nodes in the trophic web of the epipelagic ecosystem (Mendoza-Portillo, 2013). This premise holds true for the cladocerans and copepods in the Arapito-Santa Fe coastal axis, which is corroborated by the greater relative abundance of the holoplankton groups. However, the low abundance of crustacean larvae as well as meroplankton in general might indicate an absence of reproductive activity because in other studies performed in the area, high abundances of crustaceans did not correspond to the month of the present work (Márquez et al., 2006a). Rather, the abundance of holoplankton suggests that the Arapito-Santa Fé coast axis is mostly a growth and feeding area.
Considering the dietary habits of the zooplankton groups identified, the food web of the mesozooplankton in the study area consisted basically of herbivores, especially cladocerans and copepods, which are organisms that tend to remain in the upper part of the water column (Calbet et al., 1999); followed by appendicularians and siphonophores, which are filtering organisms that include different organisms in their diet (coccolithophores, diatoms, radiolarians and foraminifera); and jellyfish, chaetognathans and luciferids, which are carnivorous or omnivorous organisms that probably play a regulatory role in the community (Hopkins et al., 1993).
The absence of significant differences in the abundance of phytoplankton and the dissimilarity of zooplankton between coastal and off-coast stations shows that the oceanographic conditions in the study area produced a relatively homogeneous distribution of organisms and a very low dissimilarity index value (17.4%). This result is likely due to the absence of heteropods in the coastal stations and the greater abundance of ostracods, siphonophores, crustacean larvae and bivalve mollusks in the offshore stations. These spatial differences were evidenced in the ANOSIM test, which indicated a greater abundance of cladocerans, copepods, appendicularians and chaetognathans in the external stations and a greater similarity of the magnitudes among these species as demonstrated by the MDS.
Cladocerans and copepods are equally abundant at the coastal and remote stations, although they dominate at the remote stations and characterize the community, and similar patterns were observed with the Chaetognatha and pteropods. These findings are not in agreement with those described by Zoppi (1961), who suggested that in coastal regions and shallow waters, the biomass and abundance of zooplankton is greater because the terrigenous nutrients increase primary production in areas near the coast.
However, the relatively high concentration of nutrients, mainly nitrite (via oxidation-reduction processes), in coastal stations due to the discharge of sewage and rivers could have influenced the lower abundance observed at the coastal stations as evidenced by the RDA.
The Arapito-Santa Fé coastal axis is a receiving body for the discharge of sewage drains from urban, agricultural and aquaculture wastewater, which is discharged into the system without prior treatment. This constant addition of nutrients has caused important changes in water quality and system dynamics, which surely leads to changes in the levels and production cycles of the organisms as well as in the composition of the populations at the impacted sites, which is consistent with the observations by Calvo-Trujillo et al. (2015) and Soto et al. (2015) for the Venezuelan coastal areas. These authors described a similar situation of abundant supply of nutrients with sufficient light, low values of Chl a and phytoplankton, thus indicating a strong circulation pattern and short residence time.
The results presented in this study also corroborate those reported by Ferraz-Reyes (1993), who indicated that the climatic (high cloudiness and rainfall and low wind speed) and oceanographic (warming and stratification, inland waters loaded with suspended material and low surface salinity) processes characteristic of the relaxation period resulted in low values of Chl a and phytoplankton abundance, with a predominance of nanoflagellates. However, if the samples had been collected at a greater depth, the ascent of the isotherms at ≤ 24°C could be demonstrated, which would indicate the beginning of the upwelling period. The predominance of nanoflagellates instead of diatoms would be explained because the waters that have risen have not yet replaced the warm nutrient- poor waters typical of the relaxation period. Therefore, the significant increase in zooplankton abundance was presumed to be the product of the growth of autotrophic and heterotrophic forms (nanoflagellates) triggered by the nutrient contributions of inland waters. This type of study should be conducted during the period of upwelling to determine the structural changes that occur in the phytoplankton and zooplankton community in response to seasonal changes.
Zooplankton biomass and abundance are rough indicators of secondary productivity, and their variability depends on the response of the constitutive taxa to the fluctuations of the environment (Mann and Lazier, 1991). Therefore, the zooplankton biomass, abundance and composition results obtained in this study offer a comparison baseline for subsequent studies in which these variables will be monitored because changes in these variables can produce negative impacts on the biological productivity of the region and environmental services that the zone provides for fisheries and tourism.
CONCLUSIONS
The abundance and richness of holoplankton in the study area explains why this region is a growth and feeding zone, and this finding is corroborated by the low concentrations of Chl a, which might indicate a high grazing rate due to the high abundance of filtering species. The reduced seasonal differentiation of zooplankton indicates a relatively homogeneous distribution of the organisms.
ACKNOWLEDGMENTS
The present study was supported by the Minister of Popular Power for the Environment of the Bolivarian Republic of Venezuela via the Environmental Consulting (Consultora Ambiental) of the Universidad de Oriente (CAMUDOCA) and the project “Levantamiento ambiental del eje costero San Pedrito - Arapo - Arapito - Playa Colorada - Santa Fe, Municipio Sucre, estado Sucre (Environmental Survey of the coastal axis San Pedrito - Arapo - Arapito - Playa Colorada - Santa Fé, Municipality of Sucre, Sucre State)”. The authors thank the support personnel and technicians who participated in the field and laboratory work as well as the reviewers who contributed to the improvement of this manuscript.
REFERENCES
Allen, T., M. Jiménez, B. Márquez e Y. Figueroa. 2007. Ictiofauna de tres praderas de Thalassia testudinum de la costa norte del Golfo de Santa Fe, Estado Sucre, Venezuela. Bol. Inst. Oceanogr. Venezuela. 46(1):67-78. [ Links ]
Álvarez-Cadena, J.N., U. Ordóñez-López, D. Valdés-Lozano, A.R. Almaral-Mendívil y A. Uicab-Sabido. 2007. Estudio anual del zooplancton: composición, abundancia, biomasa e hidrología del norte de Quintana Roo, Mar Caribe de México. Rev. Mex. Biodivers., 78: 421-430. [ Links ]
Alvera-Azcárate, A., A. Barth and R.H. Weisberg. 2009. A nested model of the Cariaco Basin (Venezuela): description of the basin’s interior hydrography and interactions with the open ocean. Ocean Dyn., 59(1):97-120. [ Links ]
Atienza, D., A. Saiz E, Skovgaard, I. Trepat and A. Calbet. 2008. Life history and population dynamics of the marine cladoceran Penilia avirostris (Branchiopoda: Cladocera) in the Catalan Sea (NW Mediterranean). J. Plankton Res., 30: 345-357. [ Links ]
Berard-Therriault, L., M. Poulin and L. Bosse. 1999. Guide d´ identification du phytoplankton marin de l´ estuarine et du golfe du Saint-Laurent. CNRC. Ottawa, Canada. 387 p. [ Links ]
Boltovskoy, D. 1999. South Atlantic zooplankton. The Netherlands. Backhuys Publishers, Leiden. Vol. I y II. 1706 p. [ Links ]
Bork, P., C. Bowler, C. de Vargas, G. Gorsky, E. Karsenti and P. Wincker. 2015. Tara Oceans studies plankton at planetary scale. Science, 348(6237): 873-873. [ Links ]
Calbet, A., E. Saint, X. Irrigoren, M. Alcazar and I. Trepat. 1999. Food availability and diel feeding rhythms in the marine copepods Acartia grani and Centropages typicus. J. Plankton Res., 21:1009-1015. [ Links ]
Calvo-Trujillo, A., J.R. Díaz-Ramos, S. Subero-Pino, L. Charzeddine-Charzeddine, K. Rincones-Reyes, L. Troccoli-Ghinaglia y B. Márquez. 2015. Variación a corto plazo del fitoplancton en la bahía de Turpialito, Golfo de Cariaco, Venezuela, durante la época de lluvias. Bol. Inst. Oceanogr. Venezuela, 54(1):13-24. [ Links ]
Campos-Hernández, A. y E. Suárez-Morales. 1994. Copépodos pelágicos del Golfo de México y Mar Caribe. 1. Biología y Sistemática. Centro de Investigaciones de Quintana Roo. CONACYT. México. 306 p. [ Links ]
Clarke, K. and R. Gorley. 2006. PRIMER v5 (and v6): User manual/tutorial. Plymouth: PRIMER-E. [ Links ]
Cupp, E. 1943. Marine plankton diatoms of North America. Bull. Scripps Inst. Oceanogr., 5:1-283. [ Links ]
D’Alelio, D., S. Libralato, T. Wyatt and M.R. d’Alcalà. 2016. Ecological-network models link diversity, structure and function in the plankton food-web. Sci. Rep., 6: 21806. [ Links ]
Estrella-López, G. 2007. Distribución espacio temporal y estructura de la comunidad fitoplanctónica del Golfo de Santa Fe, Venezuela. Trab. Ascenso. Dpto. de Biología, Escuela de Ciencias, Universidad de Oriente, Cumaná, Venezuela. 741 p. [ Links ]
Ferraz-Reyes, E. 1993. Estudio del fitoplancton en la cuenca Tuy-Cariaco, Venezuela. Bol. Inst. Oceanogr. Venezuela, 32(1 y 2):111-124. [ Links ]
Fofonoff, P.W. 1994. Marine cladocerans in Narraganset Bay. PhD Thesis, University of Rhode Island, Kingston. 51 p. [ Links ]
Gordon, L.I., J.C. Jennings, A.A. Ross and J.M. Crest. 1993. A suggested protocol for continuous flow automated analysis of seawater nutrients. Corvallis: College of Oceanic and Atmospheric Sciences, Oregon State University, USA. 55 p. [ Links ]
Heneghan, R.F., J.D. Everett, J.L. Blanchard and A.J. Richardson. 2016. Zooplankton are not fish: improving zooplankton realism in size-spectrum models mediates energy transfer in food webs. Front. Mar. Sci., 3: 201. [ Links ]
Hernández-Trujillo, S., G. Esqueda-Escárcega, J.R. Hernández-Alfonso y R. Pacheco-Chávez. 2010. Variación diaria de la abundancia del zooplancton en Bahía Magdalena, B.C.S. México. Rev. Mex. Biodiv., 81:913-920. [ Links ]
Hopkins, T.L, T. M. Lancraft, J. J. Torres and J. Donnell. 1993. Community structure and trophic ecology of zooplankton in the Scotia Sea marginal ice zone in winter (1988). Deep Sea Res., 40(1):81-105. [ Links ]
Jose, E.C., E.F. Furio, V.M. Borja, N.C. Gatdula and M.D. Santos. 2015. Zooplankton composition and abundance and its relationship with physico-chemical parameters in Manila Bay. Oceanography, 3:136. Doi:10.4172/2332-2632.1000136. [ Links ]
Kiørboe, T. 2008. A mechanistic approach to plankton ecology. Princeton University Press, Princeton, New Jersey, USA. 224 p. [ Links ]
Legaré, H. 1961. Estudios preliminares del zooplancton en la región de Cariaco. Bol. Inst. Oceanogr. Univ. Oriente, 1(1):191-218. [ Links ]
Liess, A., O. Rowe, S. N. Francoeur, J. Guo, K. Lange, A. Schröder and C. L. Faithfull. 2016. Terrestrial runoff boosts phytoplankton in a Mediterranean coastal lagoon, but these effects do not propagate to higher trophic levels. Hydrobiologia, 766(1): 275-291. [ Links ]
Litchman, E., M.D. Ohman and T. Kiørbe. 2013. Trait-based approaches to zooplankton communities. J. Plankton Res., 35: 473-484. [ Links ]
López-Monroy, F. y L. Troccoli-Ghinaglia. 2014. Aproximación sobre la climatología de la Isla de Margarita y su importancia en los procesos oceánicos. Saber, 26 (4): 465-471. [ Links ]
Lorenzoni, L., G.T. Taylor, C. Benitez-Nelson, D.A. Hansell, E. Montes, R. Masserini, K. Fanning, R. Varela, Y. Astor, L. Guzmán and F.E. Muller-Karger. 2013. Spatial and Seasonal variability of dissolved organic matter in the Cariaco Basin. J. Geophys. Res. Biogeosci., 118:1-12. [ Links ]
Mann, K. and J. Lazier. 1991. Dynamics of marine ecosystems. Blackwell Scientific Publications. Oxford. 466 p. [ Links ]
Marazzo, A. and J.L. Valentin. 2000. Daily variation of marine cladoceran densities in a tropical bay-Brazil. Hydrobiologia. 428:205-208. [ Links ]
Marazzo, A. and J.L. Valentin. 2001. Spatial and temporal variations of Penilia avirostris and Evadne tergestina (Crustacea, Branchiopoda) in a tropical bay, Brazil. Hydrobiologia, 445:133-139. [ Links ]
Marazzo, A. and J.L. Valentin. 2004. Population dynamics of Pseudevadne tergestina (Branchiopoda:Onychopoda) in Guanabara Bay, Brazil. Braz. Arch. Biol. Technol., 47:713-723. [ Links ]
Marcano, Y., B. Márquez, J.R. Díaz-Ramos, L. Troccoli, B. Marín y I. Salazar. 2010. Variables fisicoquímicas que influyen a corto plazo en el zooplancton de la Bahía de Mochima, Venezuela. Bol. Inst. Oceanogr. Venezuela, 49 (2):129-145. [ Links ]
Marín, B., C. Lodeiros, D. Figueroa y B. Márquez. 2004. Distribución vertical y abundancia estacional del microzooplancton y su relación con los factores ambientales en Turpialito, Golfo de Cariaco, Venezuela. Rev. Cientif. FCV-LUZ., 14(2):133-139. [ Links ]
Márquez, B. y M. Jiménez. 2002. Moluscos asociados a las raíces sumergidas del mangle rojo Rhizophora mangle, en el Golfo de Santa Fe, Estado Sucre, Venezuela. Rev. Biol. Trop., 50 (3/4):1101-1112. [ Links ]
Márquez-Rojas, B., J.P. Blanco-Rambla, M. Jiménez y T. Allen. 2006a. Crustáceos asociados a las raíces del mangle rojo Rhizophora mangle (L.) en el Golfo de Santa Fe, Estado Sucre, Venezuela. Ciencia, 14 (1):12-27. [ Links ]
Márquez, B. , B. Marín, E. Zoppi y C. Moreno. 2006b. Zooplancton del Golfo de Cariaco. Bol. Inst. Oceanogr. Venezuela, 45(1):61-78. [ Links ]
Márquez, B., B. Marín, J.R. Díaz-Ramos, L. Troccoli y S. Subero-Pino. 2007. Variación estacional y vertical de la biomasa del macrozooplancton en la bahía de Mochima, Estado Sucre - Venezuela, durante 1997-1998. Rev. Biol. Mar. Oceanogr., 42(3):241-252. [ Links ]
Márquez, B., B. Marín, J.R. Díaz-Ramos y L. Troccoli. 2008. Biomasa, densidad y composición zooplanctónica de la bahía de Mochima, Venezuela. Gayana. 72(1):52-67. [ Links ]
Márquez, B., J.R. Díaz-Ramos, L. Troccoli, B. Marín y R. Varela. 2009. Densidad, biomasa y composición del zooplancton, en el estrato superficial de la cuenca de Cariaco, Venezuela. Rev. Biol. Mar. Oceanogr., 44 (3):737-749. [ Links ]
Márquez, B., L. Troccoli, L.M. Marcano, J. Morales, T. Allen, B. Marín y J.R. Díaz-Ramos. 2011. Estructura comunitaria del zooplancton en dos localidades del golfo de cariaco, Venezuela. Bol. Inst. Oceanogr. Venezuela, 50(2):103-119. [ Links ]
Martínez-Barragán, M., J. Medina-Calderón, A. Franco-Herrera y A. Santos-Martínez. 2009. La comunidad de copépodos (Crustacea) en las islas de Providencia y Santa Catalina (Caribe colombiano) durante el período lluvioso de 2005. Bol. Invest. Mar. Cost., 38(1):85-103. [ Links ]
Martínez, G. 2009. Aspectos abióticos. Estudio de línea base ambiental del eje costero San Pedrito-Playa Colorada-Arapito, Municipio sucre, estado Sucre. Informe presentado a la Consultora Ambiental de la Universidad de Oriente (CAMUDOCA), Cumaná, Venezuela. 125 p. [ Links ]
Mendoza-Portillo, F.J. 2013. Estructura de la comunidad del holoplancton y meroplancton durante invierno y verano en el golfo de California. Trabajo de grado de Maestro en Ciencias en Manejo de Recursos Marinos. Instituto Politécnico Nacional, La Paz, Baja California Sur. 86 p. [ Links ]
Menge, B.A. and D.N. Menge. 2013. Dynamics of coastal meta-ecosystems: the intermittent upwelling hypothesis and a test in rocky intertidal regions. Ecol. Monograph., 83(3):283-310. [ Links ]
Michael, H. B. and M. Foyo. 1976. Caribbean zooplankton. Part I. Shiphonophora, Heteropoda, Copepoda, Euphausiacea, Chaetognatha and Salpidae. Office of Naval Research Department of Navy. Bethesda, Maryland. 712 p. [ Links ]
Moustaka-Gouni, M., S. Genitsaris, K. A. Kormas, M. Scotti, E. Vardaka and U. Sommer. 2016. Heterotrophic pico-and nano-flagellates: A food web within the pelagic food webs. Rapp. Comm. int. Mer Médit., 41:401 p. [ Links ]
Muylaert, K., K. Sabbe and W. Vyerman. 2009. Changes in phytoplankton diversity and community composition along the salinity gradient of the Schelde estuary Belgium/The Netherlands. Est. Coast. Shelf Sci., 82:335-340. [ Links ]
Okuda, T. 1975. Características Hidroquímicas del Golfo de Santa Fe y áreas adyacentes. Bol. Inst. Oceanogr. Univ. Oriente, 14 (2):251-268. [ Links ]
Okuda, T. y J. A. Benítez. 1974. Condiciones hidroquímicas del capas superiores en la Fosa de Cariaco y áreas adyacentes durante la época lluviosa. Bol. Inst. Oceanogr. Univ. Oriente, 13(1 y 2):147-162. [ Links ]
Onbé, T. 1999. Ctenopoda and Onychopoda (=Cladocera): 797-813. En: Boltovskoy, D. (Ed.). South Atlantic Zooplankton. Backhuys Publishers, 1: 999 p. [ Links ]
Postel, L., H. Fock and W. Hagen. 2000. Biomass and abundance: 83-174. En: Harris, R.P., P.H. Wiebe, J. Lenz, H.R. Skjoldal and M. Huntley. (Eds.). Ices Zooplankton methodology manual. San Diego, California, Academic Press, 684 p. [ Links ]
RaphaëlN’doua, E., A.M. Kouassi and A. MaryseN’guessan. 2009. Spatio-Temporal variations of the zooplankton abundance and composition in a West African tropical coastal lagoon (Grand-Lahou, Côte d’Ivoire). Hydrobiologia, 624:171-189. [ Links ]
Razouls, C., F. de Bovée, J. Kouwenberg and N. Desreumaux. 2005-2017. Diversity and geographic fistribution of marine planktonic copepods. http://copepodes.obs-banyuls.fr/en. 25/04/2017. [ Links ]
Rivera-Tenembaum, D. 2006. Dinoflagelados e tintinídeos da região central da Zona Econômica Exclusiva brasileira: guia de identificação. Museu Nacional, Rio de Janeiro. 287 p. [ Links ]
Rueda-Roa, D. and F. Muller-Karger. 2013. The Southern Caribbean Upwelling System: Sea surface temperature, wind forcing and chlorophyll concentration patterns. Deep-Sea Res. I., 78:102-114. [ Links ]
Rueda-Roa, D., J. Mendoza, F. Muller-Karger, J.J. Cárdenas, A. Achury and Y. Astor. 2017. Spatial variability of Spanish sardine (Sardinella aurita) abundance as related to the upwelling cycle off the southeastern Caribbean Sea. PLoS ONE., 12 (6):e0179984. Doi: https://doi.org/10.1371/journal.pone.0179984. [ Links ]
Ruíz-Pineda, C., E. Suárez-Morales y R. Gasca. 2016. Copépodos planctónicos de la Bahía de Chetumal, Caribe Mexicano: variaciones estacionales durante un ciclo anual. Rev. Biol. Mar. Oceanogr., 51(2):301-316. [ Links ]
Scranton, M.I., G.T. Taylor, R. Thunell, C.R. Benitez-Nelson, F. Muller-Karger y K. Fanning. 2014. Interannual and subdecadal variability in the nutrient geochemistry of the Cariaco Basin. Oceanography. 27(1):148-159. Doi: http://dx.doi.org/10.5670/oceanog.2014.18. [ Links ]
Selleslagh, J. y R. Amara. 2008. Environmental factors structuring fish composition and assemblages in a small macrotidal estuary (eastern English Channel). Estuar. Coast. Shelf Sci., 79:507-517. [ Links ]
Sheskin, D. 2004. Parametric and nonparametric statistical procedures. 4 ed. Boca Raton, Florida: Editorial Chapman & Hall. 585 p. [ Links ]
Smitha, P., A. Shivashankar y G. Venkataramana. 2013. Zooplankton diversity of Chikkadevarayana Canal in relation to physico-chemical characteristics. J. Env. Biol., 34:819-24. [ Links ]
Soto, G., L. Troccoli, J. R. Díaz, G. Martínez y R. Crescini. 2015. Variabilidad a corto plazo de la hidrografía y fitoplancton en la ensenada de Turpialito, Venezuela. Bol. Inst. Oceanogr. Venezuela, 54(2):125-137. [ Links ]
Strickland, J. D. and T. R. Parsons. 1972. A practical handbook of seawater analysis. 2 ed. J. Fish. Res. Board Can., 311p. [ Links ]
Taylor, G.T., F.E. Muller-Karger, R.C. Thunell, M.I. Scranton, Y. Astor and R. Varela. 2012. Ecosystem responses in the southern Caribbean Sea to global climate change. Proc. Natl. Acad. Sci. USA. 10.1073/pnas.120751410. [ Links ]
Teixeira, P., S. Kaminski, T. Avila, A. Cardozo, J. Bersano and A. Bianchini. 2010. Diet influence on egg production of the copepod Acartia tonsa (Dana, 1896). An. Acad. Bras. Ciênc., 82 (2):333-339. [ Links ]
Thomas, C. 1997. Identifying marine diatoms and dinoflagellates. Academic Press. New York, USA. 808 p. [ Links ]
Tregouboff, G. and M. Rose. 1957. Manuel de Planctonologie Medditerraneenne. I y II eds. Paris: Centre de la Recherche Scientifique. 590 p. [ Links ]
Utermöhl, H. 1958. Zur vervolkommung der quantitativen phytoplankton methodig. Mitt. Verein. Theor. Argiew. Limnol. 9:1-38. [ Links ]
Zoppi, E. 1961. Distribución vertical del zooplancton en el Golfo y extremo este de la Fosa de Cariaco. Bol. Inst. Oceanogr. Univ. Oriente., 1 (1):219-248. [ Links ]
Zoppi, E. 1971. Apendicularias de la región oriental de Venezuela. Stud. Fauna. Curaçao Caribbean Isl. 38(1):1-109. [ Links ]
Received: November 01, 2017; Accepted: September 03, 2018