Services on Demand
Journal
Article
Indicators
-
Cited by SciELO
-
Access statistics
Related links
-
Cited by Google
-
Similars in SciELO
-
Similars in Google
Share
Boletín de Investigaciones Marinas y Costeras - INVEMAR
Print version ISSN 0122-9761
Bol. Invest. Mar. Cost. vol.48 no.2 Santa Marta July/Dec. 2019 Epub Dec 11, 2019
https://doi.org/10.25268/bimc.invemar.2019.48.2.768
Research Articles
Characterization of coccolithophore assemblages in the offshore basins of the Colombian Pacific
1. Instituto de Investigaciones en Estratigrafía IIES, Universidad de Caldas, Manizales, Colombia. osorio.gomez.esteban@gmail.com.
2. Departamento de Ciencias Geológicas Universidad de Caldas. Calle 65 N°26-10, Manizales, Colombia.
3. Centro de Innovación y Tecnología Instituto Colombiano del Petróleo, ICP-Ecopetrol, km 7vía Piedecuesta, Santander, Colombia.
This study was conducted on coccolithophores recovered from 39 piston-core samples taken offshore Chocó and Tumaco basins, on the Colombian Pacific. Qualitative and quantitative analyses of the coccoliths showed changes in the relative abundances and the state of preservation in the two basins. The examined sediments were characterized by the coccoliths Gephyrocapsa oceanica, Gephyrocapsa muellerae, Gephyrocapsa <3 μm, Emiliania huxleyi, Calcidiscus leptoporus and Helicosphaera carteri, which presented abundances higher than 2 %. We also identified a minority assemblage (<2%) constituted by Ceratolithus spp, Coccolithus pelagicus, Florisphaera profunda, Helicosphaera princei, Helicosphaera sellii, Helicosphaera wallichii and Pontosphaera spp. together with reworked specimens of Reticulofenestra spp, Sphenolithus spp. and Discoaster spp. The recovery of E. huxleyi as part of the assemblage indicates that the studied sediments are younger than the biozone NN21, covering an age range of Middle Pleistocene (Ionian). The number of coccoliths per gram (cc/g) was calculated, demonstrating an average of 5.7x106 cc/g and 1.2x107 cc/g for Chocó and Tumaco basins, respectively. In order to interpret the causes of this variance, we performed a multivariate redundancy analysis (RDA), showing that the distance to the coastline is the controlling factor of the fluctuations of the relative abundances and distribution of the coccoliths in both basins.
KEYWORDS: Coccolithophores; Colombian Pacific; Chocó basin; Tumaco basin; Quaternary
El presente estudio fue llevado a cabo en los cocolitóforos extraídos de 39 piston core perforados en el offshore de las cuencas Chocó y Tumaco, en el Pacífico colombiano. Los análisis cualitativos y cuantitativos mostraron cambios en las abundancias relativas y en el grado de preservación de los 20 taxones identificados. Los sedimentos examinados en ambas cuencas revelaron tener una asociación de cocolitos >2% constituida por Gephyrocapsa oceanica, Gephyrocapsa muellerae, Gephyrocapsa <3 μm, Emiliania huxleyi, Calcidiscus leptoporus y Helicosphaera carteri. Con una abundancia más baja (<2%), se observaron Ceratolithus spp, Coccolithus pelagicus, Florisphaera profunda, Helicosphaera princei, Helicosphaera sellii, Helicosphaera wallichii y Pontosphaera spp., junto con formas retrabajadas de Reticulofenestra spp., Sphenolithus spp. y Discoaster spp. La identificación de E. huxleyi dentro de la asociación indica que la edad de estos sedimentos no debe ser más antigua que la biozona NN21 del Pleistoceno Medio (Ioniano), aunque formas retrabajadas de edad Mioceno-Plioceno también fueron vistas en los sedimentos. Las estimaciones del número de cocolitos por gramo (cc/g) indicaron que la abundancia promedio de la Cuenca Chocó fue 5,7x106 cc/g, la cual es menor que la Cuenca Tumaco con 1,2x107 cc/g. El análisis estadístico de redundancia (RDA) sugiere que la distancia a la línea de costa es la variable determinante que controla estas diferencias de abundancias relativas y distribución de los cocolitos en ambas cuencas.
PALABRAS CLAVES: Cocolitóforos; Pacífico colombiano; Cuenca Chocó; Cuenca Tumaco; Cuaternario
INTRODUCTION
Coccolithophores are unicellular, marine microalgae belonging to the Haptophyta Division, which normally have an external structure composed of tiny calcium carbonate plates (<30 μm) known as coccoliths (Jordan et al., 1995). Once the microorganism dies, the coccoliths sink into the water column, either in the form of fecal pellets or disarticulated fragments included in the "marine snow", reaching the ocean floor where they become part of the sediment (Young et al., 2003; Flores and Sierro, 2007). Once found in rocks, coccolithophores are included in the group of calcareous nannofossils, including some similar forms that have been observed in sedimentary record as old as the late Triassic (Bown, 1998). Coccolithophores have been observed from the polar oceans to the tropical seas as part of marine phytoplankton, living exclusively in the photic zone (Flores and Sierro, 2007). Due to their high abundance and capacity for processes such as photosynthesis and calcification, coccolithophores play a fundamental role in the regulation of the ocean-atmosphere system through the planet's biogeochemical CO2 cycles (Thierstein and Young, 2004). The study of these microorganisms and their fossil record has gained importance in paleoclimatic and paleoceanographic research, as their high susceptibility to changes in sea surface temperature, nutrient availability, luminosity, and surface salinity has been demonstrated. Likewise, their use as a biostratigraphic tool is common and very useful (Giraudeau, 1992; Flores et al.., 1997, 1999, 2000; Balch, 2004; Antunes, 2007; Saavedra-Pellitero et al., 2010, 2011; Poulton et al., 2017). The Colombian equatorial Pacific Ocean, where this work is carried out, has particular oceanographic characteristics, among which the following stand out: 1) Its high precipitation rates (2,000 to 12,700 mm/year) that to a great extent feed the channels of the rivers San Juan, Patía, and Mira, among others. These freshwater tributaries carry a large amount of sediment calculated at 35x109 m3/year, which considerably affects the physical-chemical properties of marine surface waters such as salinity, turbidity, nutrient concentration and temperature (Poveda and Mesa, 2000; Restrepo and Kjerfve, 2000; CCCP, 2002; Betancur and Martínez, 2003; Gómez and Martínez, 2005; López and Costeros, 2006; Restrepo, 2006; Restrepo and López, 2008; Patarroyo and Martínez 2013, 2016); 2) The seasonal dynamics of oceanic upwelling, caused by low-level winds that cross the Panamanian Isthmus from January to March, produce a cooling of surface waters and an increase in nutrient concentration due to the ascent of deeper waters to the surface (D'Croz and O'Dea, 2007, 2009).
The objective of this study is to determine the composition and distribution of the quaternary assemblages of coccoliths analyzed in the 39 core tops extracted from the seabed in the departments of Chocó and Nariño (Figure 1). This information will establish a time range and examine the distribution pattern of the coccolithophores communities that inhabit these tropical marine waters near the Colombian Pacific coast. Additionally, this information is a starting point for databases through which to analyze the composition of calcareous nanoplankton in the Colombian territory.
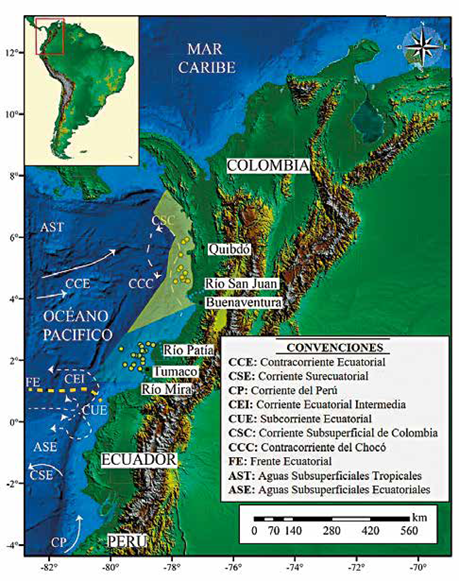
Figure 1 Some characteristics of ocean currents in the Colombian Pacific Ocean. The Surface Currents are represented by the continuous white lines; the discontinuous white lines indicate the Subsurface Currents. The Equatorial Front is represented by discontinuous orange lines. Tropical and Equatorial Surface Waters are also represented. The yellow and blue polygons delimit the areas of the Chocó and Tumaco Basins respectively, and the yellow dots indicate the location of the core pistons. Map drawn up by integrating the information reported in Wyrtki (1966), Restrepo and Correa (2002), Fiedler and Talley (2006), Restrepo and López (2008) and Rodríguez-Rubio et al. (2008).
STUDY AREA
The eastern tropical Pacific is an area characterized by the confluence of marine currents from the north (California Current) and the south (Peru Current), giving rise to a border zone known as the Equatorial Front, which limits circulation between temperate surface waters of the north and cold highly saline equatorial waters of the south (Wyrtki, 1965, 1966; Pak and Zaneveld, 1974; Okada, 1983; Fiedler and Talley, 2006). The Pacific Ocean in Colombia is characterized by an important ocean-atmosphere dynamic in which the trade winds of the north and south converge, controlling the displacement of the Intertropical Convergence Zone (Wyrtki, 1965, 1966; Pak and Zaneveld, 1974; Fiedler and Talley, 2006). According to Knauss (1960), Wyrtki (1966) and Fiedler and Talley (2006), the oceanic currents that influence the surface dynamics of the Colombian Pacific are: (a) the Equatorial Countercurrent (ECC) from tropical-subtropical waters with high nutrient concentration (>25 °C and <34 psu); (b) the Equatorial Under Current (SEC) which transports considerable volumes of warm water to the west, and its northern boundary to the west is with the ECC; and (c) the Peru Current (PC), also known as the Humboldt Current, characterized by its cold temperate and eutrophic waters (15 to 28 °C and >36 psu). On the other hand, among the subsurface currents circulating in the Colombian Pacific are the Equatorial or Cromwell Under Current and the Intermediate Equatorial Current (Restrepo and Correa, 2002). In addition to these, two currents described by Restrepo and López (2008) and Rodríguez-Rubio et al. (2008) on the continental margin of the Colombian Pacific are reported as follows: the Colombian Subsurface Current (CSC) and the Chocó Counter Current (CCC).
Salinities and temperatures in the Colombian Pacific Ocean behave inversely. In regions with high temperatures, salinity tends to be low (CCCP, 2002). The sea surface temperature has oscillated between 24.5 and 29.8 °C during the last 100 years (Málikov and Villegas, 2005; NOAA, 2017), and is warmer in areas close to the coast and cooler towards more distal regions (Figure 2). Surface waters tend to have a higher surface temperature in the Chocó region than in Tumaco region, mainly because the Chocó Countercurrent transports warm waters from the north (CCCP, 2002). This pattern is closely related to changes in salinity in the Colombian Pacific, with coastal ranges between 30 and 32.8 PSU and higher values around 35 PSU as it moves south and west (CCCP, 2002) (Figure 2).
MATERIALS AND METHODS
Between November 25 and December 11, 2009, and commissioned by Ecopetrol S.A., the company GEMS-HRT together with DIMAR carried out a sampling process of the seabed in the technical evaluation areas (TEAs) of Merayana (Chocó Basin) and Egoro (Tumaco Basin), in offshore zones of the departments of Chocó and Nariño respectively. Thirty-nine piston core samples were collected from the vessel ARC Providencia at an average distance of 50 km measured from the coastline. The points were selected from seismic information, bathymetric images, and the signal of a 3.5 KHz high frequency sediment penetrator. The samples used in this study belong to the first 11 cm (base roof) of the 39 core tops, distributed as follows: 18 piston core samples in the Tumaco Basin (TEA Egoro) and 21 piston core samples in the Chocó Basin (TEA Merayana) (Figure 3; Table 1). It should be clarified that the first centimeters of the recovered sedimentary material could have become mixed with sediments, as a result of using the piston core technique.
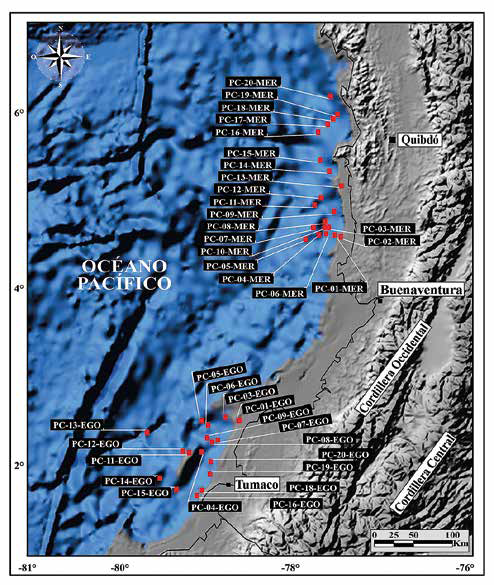
Figure 3 Location of sampling areas in the Chocó and Tumaco Basins. Note the special location of sample PC-11-EGO on a topographic high of the sea floor.
Table 1 Information on piston core samples from the Colombian Pacific. Samples that did not contain any coccolithophores are not included in this table. *The values of sediment discharge rates have been taken from Restrepo et al. (2000), Restrepo (2006) and Restrepo and López (2008).
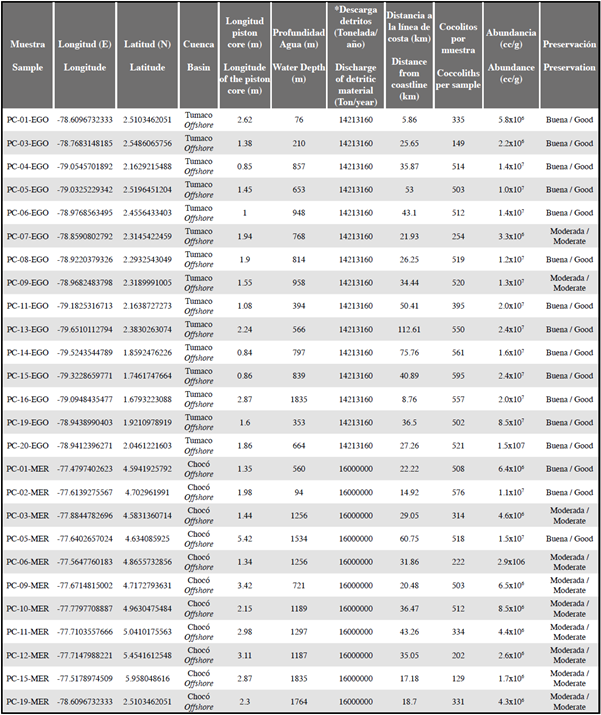
Sample preparation
The collected material was prepared in the Calcareous Microfossils laboratory at the Instituto de Investigaciones en Estratigrafía (IIES), Universidad de Caldas, following the decanting technique proposed by Flores and Sierro (1997). This method requires weighing the amount of sediment to be decanted, which then allows a homogeneous distribution of the microfossils in an area of known dimensions, thus enabling the calculation of coccolith abundance per gram of sediment using the formula:
N= n x R2 x V x r-2 x g-1 x v-1.
Where n is the number of nannofossils counted in a given area, R is the radius of the Petri dish where the sample was decanted, V is the volume of water added to the dry sediment during preparation, r is the radius of the visual field of the microscope used in the count, g is the weight of the dry sediment, and v is the volume of solution added with the micropipette.
A Nikon Digital Sight DS-Ri1 camera with a DS-U2 controller attached to a Nikon Eclipse 50i POL petrographic microscope was used for the photographic records, using Nikon Imaging NIS-Elements software version 3.2. In order to obtain higher resolution and more detailed photographs, the most abundant samples were prepared for image taking using the Scanning Electron Microscope (SEM).
Qualitative and quantitative microscopic analyses
To study the coccolithophores, 39 slides were prepared and subsequently analyzed using a petrographic microscope with a magnification of 1000x. This analysis was divided into two parts. Initially, a qualitative estimate of the state of preservation of the coccoliths was carried out according to the criteria established by Roth and Thierstein (1972); Roth (1983); Raffi and Flores (1995) where: (G) Good: The specimens present little or no dissolution and/or recrystallization. (G-M) Good-Moderate: Light to moderate dissolution and/or recrystallization and species and identification is limited. (M) Moderate: Specimens show moderate dissolution and/or excessive recrystallization. (P) Poor: The microfossils present extreme dissolution and/or excessive recrystallization.
Subsequently, all coccolithophores were taxonomically identified, following the Young et al. taxonomic guide (2003) for Pleistocene coccolith assemblages and the descriptions by Perch-Nielsen (1985) for Miocene-Pliocene reworked forms. Coccoliths of Gephyrocapsa, which were characterized by being smaller than 3 μmand not being recognized by the petrographic microscope due to their tiny size, were grouped under the name of Gephyrocapsa <3 μm. A list of the taxa found in the samples is presented in Appendix A.
Finally, a quantitative analysis was performed using two types of counts. The first was a count of 500 specimens per sample, regardless of the number of fields of view observed. In samples with a low number of coccoliths, a maximum of 400 visual fields were counted. This method guarantees, to a 95% confidence level, that specimens with 1% abundance are included within the assemblage (Dennison and Hay, 1967; Fatela and Taborda, 2001). The second count was conducted in 40 visual fields, looking exclusively for those species that showed abundance <2%, in order to quantify the species underestimated or overestimated in the first count (Appendix B).
Statistical analysis
The abundance matrix used for statistical analysis was created by omitting samples with an abundance of less than 100 specimens and discarding species with abundances of less than 2% (Schneider et al., 2011). This ensures that interpretations based on statistical results contain the most representative taxa of the assemblage (Dennison and Hay, 1967; Fatela and Taborda, 2001; Schneider et al., 2011). Additionally, reworked forms of calcareous nannofossils were also excluded from statistical treatment in order to avoid alterations during processing.
A redundancy analysis (RDA) was performed to summarize the relationships between coccolithophores species and environmental parameters (Zar, 1996; Hammer et al, 2001; Whitlock and Schulter, 2009; Borcard et al, 2011). Counts were standardized from a Hellinger-type transformation to allow low incidence taxa to be weighted in statistical analysis in the R core Team software, (2015) with the Vegan package. The significance of each environmental parameter was evaluated based on the length of the gradient in the management space and its correlation with the two main axes. The direction and size of the vectors are indicators of the influence of variables on the distribution of taxonomic groups.
RESULTS
Age
The presence of Emiliania huxleyi - whose appearance dates back to the Ionian (Middle Pleistocene) - in the assemblage marks the lower limit of the biozone NN21 (Martini, 1971). This bioevent dates back 290,000 yrs. (Thierstein et al., 1977; Backman et al., 2012). From the assemblage of coccoliths found, it is established that these sediments should be no older than the biozone NN21 of the Ionian (Middle Pleistocene).
Assemblage and abundance of coccolithophores
The results of the microscopic analysis showed that out of 39 samples analyzed, 9 samples - 8 from the Chocó Basin and 1 from the Tumaco Basin - were barren. The rest of the samples containing microfossils made it possible to identify 20 taxa of coccolithophores in both localities (Figure 4).
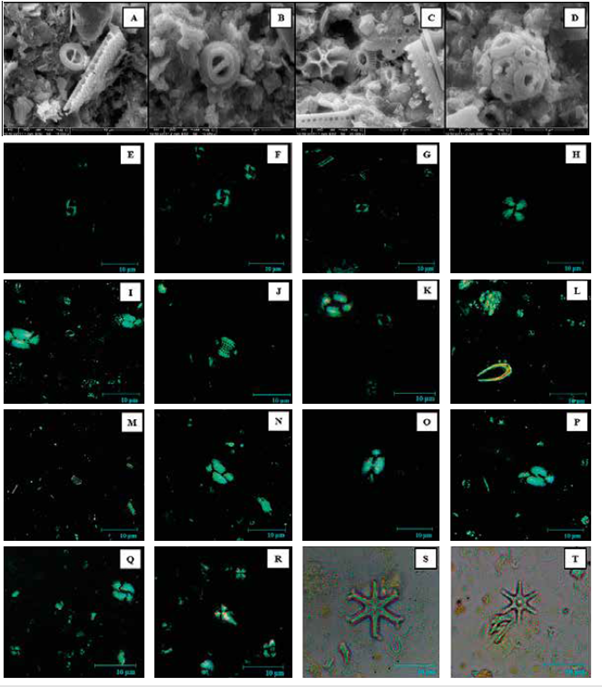
Figure 4 Species of coccoliths found in the analyzed samples. Images taken under Scanning Electron Microscope (SEM). A. Gephyrocapsa oceanica (10 μm). B. Gephyrocapsa oceanica (5 μm). C. Emiliania huxleyi (5 μm). D. Coccosphere of Gephyrocapsa spp. (5 μm). Images captured under petrographic microscope and enlarged by 1000x. Crossed Nicol (dark field). Parallel Nicol (light field). E. Gephyrocapsa oceanica. F. Gephyrocapsa muellerae. G. Emiliania huxleyi. H. Calcidiscus leptoporus. I. Helicosphaera carteri. J. Pontosphaera spp. K. Coccolithus pelagicus. L. Ceratolithus spp. M. Florisphaera profunda. N. Helicosphaera princei. O. Helicosphaera sellii. P. Helicosphaera wallichii. Q. Reticulofenestra pseudoumbilicus. R. Sphenolithus heteromorphus (lower) and Sphenolithus abies (higher). S-T. Discoaster variabilis.
Among the taxa recorded, G. oceanica, G. muellerae, Gephyrocapsa <3 μm, E. huxleyi, C. leptoporus and H. carteri presented relative abundances greater than 2% (Figure 4 E-I; Table 2). In contrast species such as Ceratolithus spp., C. pelagicus, F. profunda, H. princei, H. sellii, H. wallichii y Pontosphaera spp. presented an abundance of less than 2% (Figure 4 J-P). Another common component of the assemblages were the reworked Miocene and Pliocene forms of calcareous nannofossils, mainly forms of Reticulofenestra spp. (Figure 4 Q). The sample PC-11-EGO was characterized by having the highest rework values, with a presence of D. variabilis, S. abies and S. heteromorphus. (Figure 4 R-T).
Although the most modern specimens of coccolithophores were seen in both the Chocó Basin and the Tumaco Basin, there was a contrast in the number of coccoliths per gram (cc/g) calculated for the two regions (Table 1). Similarly, qualitative analyses of the degree of preservation of the coccoliths varied between the two basins, showing that in the Tumaco Basin, overall preservation was good, without excessive dissolution and/or recrystallization; whereas, the taxa of the Chocó Basin commonly presented moderate preservation (Table 1). However, recognition at species level was achieved in most of the specimens (Figure 5).
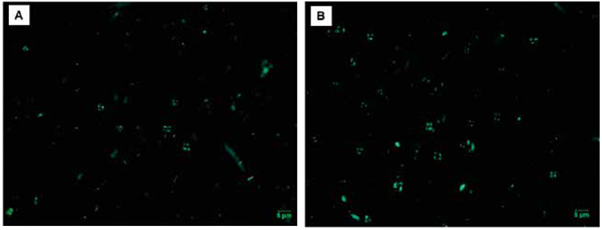
Figure 5 Example of the abundance and preservation of coccoliths. A. sample PC-19-MER; B. sample PC-15-EGO. Note the difference in abundance and preservation in the two samples.
Calculations of coccoliths abundances per gram of sediment showed that the Tumaco Basin has the highest values, with an average of 1.2x107 cc/g. The highest abundances are found in the samples PC-11-EGO, PC-13-EGO, PC-14-EGO, PC-15-EGO and PC-16-EGO, with values of around 2.0x107 cc/g, while the samples with lower abundance correspond to PC-12-EGO and PC-18-EGO with values of 7.9x105 cc/g and 6.8x105 respectively. The points sampled from the Chocó Basin present lower values, with an average of 5.7x106 cc/g, where samples PC-02-MER and PC-05-MER present the highest abundance, with values of 1.1x107 and 1.5x107 cc/g respectively. A lower concentration is observed in the samples PC-15-MER and PC-17-MER with values between 1.2x106 and 1.7x106 cc/g (Figure 6; Table 2).
Multivariate analyses
Redundancy analysis RDA (Zar, 1996; Hammer et al., 2001; Whitlock and Schulter, 2009; Borcard et al., 2011) provided significant information on the relationship between coccolithophore abundance, demonstrating that the most explanatory and/or correlated factor with respect to taxon distribution and abundance is related primarily to distance from the coastline (axis 1; 1.4276028; DC) compared to water column depth (axis 2; 0.5770010; WD) and sediment discharge (-0.4538382; T.a), (Table 3; Figure 7). These parameters explain 74%, 14%, and 12% respectively of the total variance. E. huxleyi, Gephyrocapsa <3 μm and G. muellerae reveal a trend towards axis 1 with negative values, while the vector of greatest magnitude on this axis is given by H. carteri, and to a lesser extent by C. leptoporus (Figure 7). As for the species G. oceanica, greater correspondence is shown with axis 2, the vector of this taxon being the closest to said axis, showing preferences for greater depth and affinity for high influence of sediments (Figure 7).
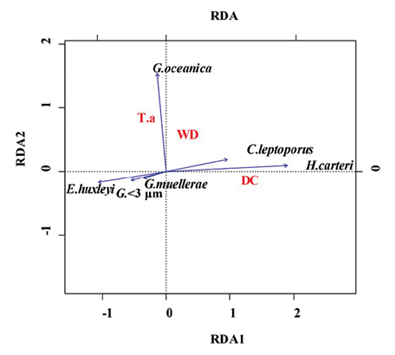
Figure 7 Trends in coccolithophore species with respect to variations with main axes, distance to coastline (DC), depth of water column (WD), and discharge of detritic material (T.a).
DISCUSSION
Comparison with other coccolithophore assemblages in the Eastern Tropical Pacific
The coccolith counts indicate that the main assemblage (greater than 2%) in the Chocó and Tumaco samples is: G. oceanica, G. muellerae, Gephyrocapsa <3 |m, E. huxleyi, C. leptoporus and H. carteri (Figure 4; Table 2). The following were observed to a lesser extent (<2%): Ceratolithus spp., Coccolithus pelagicus, F. profunda, H. princei, H. sellii, H. wallichii and Pontosphaera spp. Together with reworked forms of R. pseudoumbilicus, D. variabilis, S. heteromorphus and S. abies (Figure 4). The dominant assemblage recorded in this study differs from the coccolithophores reported by Saavedra-Pellitero et al. (2010; 2011) near the Cocos and Carnegie Ridges (Figure 8). One of the most striking differences in this comparison is the number of specimens of F. profunda to the west in the Quaternary sediments of the Cocos Ridge, and to the south in the Carnegie Ridge (Ahagon, 1993; Saavedra-Pellitero et al., 2010, 2011).
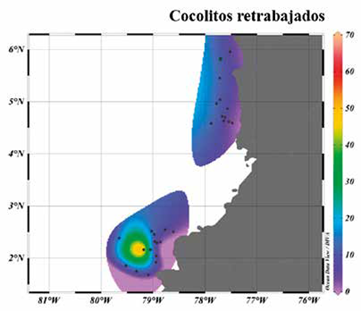
Figure 8 Geographic distribution and relative abundance maps of the groups considered to be reworked. The area with the highest concentration of these corresponds to the sample PC-11-EGO.
The most abundant species reported in the Cocos Ridge is F. profunda (34.89%), followed by G. oceanica (21.98%), Gephyrocapsa<3 μm (18.74%), and G. muellerae (10.75%). In these assemblages, C. leptoporus, E. huxleyi and H. carteri present abundances of less than 4%. The most abundant groups in the Carnegie Ridge continue to be F. profunda (28.8%) and G. oceanica (26.2%), followed by Gephyrocapsa <3 μm (16.1%), E. huxleyi (11%), G. muellerae (10.75%) and C. leptoporus (4.3%) (Figure 9), while this paper reports abundances of G. oceanica (24-26%), G. muellerae (18-22%), Gephyrocapsa <3 μm (14-15%), E. huxleyi (12-14%), C. leptoporus (3-4%), H. carteri (3-2%), and F. profunda with abundances of below 2% (Table 2). These distribution patterns in taxa may reflect variations in the species' paleoecological affinities and/ or sorting effects associated with dissolution. However, discriminating between the possible factors that affected the distribution of assemblages requires a more comprehensive study that includes other chemical and paleontological tools along with the use of statistical techniques.
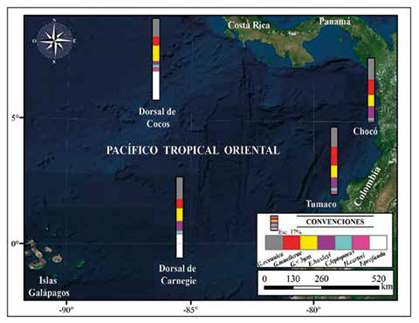
Figure 9 Comparison of the assemblages and average abundances of coccolithophores reported in piston core samples taken on the Cocos Ridge, the Carnegie Ridge (Saavedra-Pellitero 2010, 2011) and the Colombian Pacific in this work.
Abundance and preservation of coccoliths: Chocó vs. Tumaco
Descriptive and quantitative analyses of coccoliths assemblages extracted from the Chocó and Tumaco basins show a similarity in the composition of the nannoflora (Figure 4-6, Table 2). However, a difference was observed in the degree of preservation and abundance between the localities (Figure 5; Table 1). In the case of the Chocó Basin, its preservation was moderate and the number of coccoliths per gram was lower (5.7x106 cc/g) compared to the Tumaco Basin. This value is related to the fact that the majority of samples presented very low abundances, 8 of 21 samples were catalogued as barren, and only 11 had a recovery higher than 100 coccoliths (Table 1). In contrast, for the Tumaco Basin, only one barren sample was obtained, estimating good preservation and an average abundance of 1.2x107 cc/g (Table 1). In an attempt to explain this difference in abundance and distribution, a multivariate RDA statistical analysis was performed evaluating the available variables that may have controlled coccoliths production. This analysis revealed that fluctuations in coccoliths abundances >2% can be explained mainly by distance from the coastline (Table 3; Figures 6 y 7). The greatest abundances of E. huxleyi and Gephyrocapsa <3 μm were found in samples whose location was close to the coastline and in shallower areas (i.e., sampling points PC-15 MER, PC-19 MER, PC-01-EGO and PC-03-EGO). Although H. carteri showed low levels of abundance (Figure 6), the distribution and abundance of this species seem to be very controlled by the distance to the coastline as supported by the RDA analysis (Table 3) with the most abundance in the samples taken furthest from the continent (Figure 7). According to statistical processing, the variables with the lowest percentages of influence are: the amount of detritus per year and depth of the water column, these factors were not determinant in the abundance of coccoliths calculated for the samples examined.
Modern studies undertaken in the oceanic waters of the Chocó Basin indicate that the temperature is higher towards the coast, and higher compared to the temperature of the Tumaco Basin. In contrast, salinity is higher towards the distal zone and is more intense than that presented in the waters of the Chocó Basin (Figure 2) (CCCP, 2002; NOAA, 2017). These characteristics are closely related to the influence of ocean currents of greater magnitude, such as the Chocó Counter Current (with less cold water) in the Chocó area, and the Peru Current (colder water masses) in areas of Tumaco (CCCP, 2002). Although in general these physical-chemical characteristics may be reflected by the microfossils found in this study, the lack of quantitative data does not allow an evaluation of the impact of these variables on the abundance and preservation of coccolithophore assemblages.
Occurrence of reworking
Reworked forms were identified and quantified among the results acquired by identifying and counting the coccoliths of the 39 samples (Figure 4 Q-T). The most common reworked species were those belonging to the genus Reticulofenestra spp. that was seen in most of the samples. The PC-11-EGO sample, located south of the Tumaco Basin, showed the highest content and variety of reworked species (Figures 3 and 9).
Sphenolithus abies, Sphenolithus heteromorphus, Discoaster variabilis and Reticulofenestra spp. were the most abundant species in that sample (Figure 9). The presence of this assemblage in Pleistocene sediments enables us to infer the contribution of marine sediments from the Miocene-Pliocene to the study area. Based on the location of the PC-11-EGO sample in the slopes of a positive relief (Figure 3), it is proposed that the origin of these calcareous nannofossils may be related to processes of seabed removal, which would incorporate forms of Neogene in Quaternary sedimentation. This seems to be supported by the fact that this was the only sample with retrieval of the oldest specimens, noticing that the surrounding sites did not present forms of Sphenolithus and Discoaster. Additionally, although the course of the Mira and Patía rivers on the Neogene marine rocks exposed in Tumaco Bay may have constituted another origin of the reworked rocks (Borrero et al, 2012; Bedoya et al, 2013), the exclusive concentration of Miocene-Pliocene nannofossils in the PC-11-EGO sample once again suggests more localized processes that would be providing older materials (Figure 3).
CONCLUSIONS
Micro paleontological analyses of 39 samples taken from the offshore Tumaco and Chocó Basins show that the main assemblage (greater than 2%) of coccolithophores is G. oceanica, G. muellerae, Gephyrocapsa <3 μm, E. huxleyi, C. leptoporus and H. carteri. In a smaller proportion (<2%) Ceratolithus spp., C. pelagicus, F. profunda, H. princei, H. sellii, H. wallichii and Pontosphaera spp. were observed along with reworked forms of Reticulofenestra spp., Sphenolithus spp. and Discoaster spp. of Miocene-Pliocene. The presence of E. huxleyi within the assemblage indicates that the age of these sediments should not be older than Ionian biozone NN21 (Middle Pleistocene). Estimates of the number of coccoliths per gram (cc/g) revealed that the average abundance of the Chocó Basin (5.7x106 cc/g) is lower compared to the Tumaco Basin (1.2x107 cc/g). According to RDA multivariate statistical analyses, this difference can be explained mainly by distance to the coast, which seems to have controlled the distribution and abundance of the coccoliths found.
REFERENCES
Ahagon, N., Y. Tanaka and H. Ujiie. 1993. Florisphaera profunda, a possible nannoplankton indicator of late Quaternary changes in sea-water turbidity at the northwestern margin of the Pacific. Mar. Micropaleontol., 22: 255-273. [ Links ]
Antunes, R. L. 2007. Nanofósseis calcários do Quartenário da margem continental brasileira. Petrobrás, p. 75. [ Links ]
Backman, J., I. Raffi, D. Rio, E. Fornaciari and H. Palike. 2012. Biozonation and biochronology of Miocene through Pleistocene calcareous nannofossils from low and middle latitudes. Newslet. Stratigr., 45 (3): 221-244. [ Links ]
Balch, W. M. 2004. Re-evaluation of the physiological ecology of coccolithophores: 165-190. In Coccolithophores, Springer, Berlin, Heidelberg. [ Links ]
Bedoya, E. L., J.A. Flores y A. Pardo. 2013. Nanofósiles calcáreos y bioestratigrafía del Mioceno Tardío del SW de la Cuenca Tumaco onshore (Pacífico Colombiano). Bol. Geol. UIS, 35(1): 55-66. [ Links ]
Betancur, M. J. e I. Martínez. 2003. Foraminíferos bentónicos recientes en sedimentos de fondo de la Cuenca de Panamá (Pacífico Colombiano), como indicadores de productividad y oxigenación. Bol. Invest. Mar. Cost., 32: 92-123. [ Links ]
Borcard, D., F. Gillet and P Legendre. 2011. Numerical ecology with R. Springer, New York, p 435. [ Links ]
Borrero, C., A. Pardo , C.M. Jaramillo, J.A. Osorio, J.A. Cardona, J-A. Flores, J-A., S. Echeverry Rosero, J. García y H. Castillo. 2012. Tectonoestratigraphy of the Cenozoic Tumaco forearc basin (Colombian Pacific) and this relationship with the northern Andes orogenic build up. J. South Am. Earth Sci., 39: 75-92. [ Links ]
Bown, P. R. 1998. Calcareous nannofossil biostratigraphy: 315. Bown, P. (Ed.). Chapman and Hall. [ Links ]
Centro Control Contaminación del Pacífico CCCP. 2002. Compilación oceanográfica de la cuenca Pacífica colombiana. Serie Publ. Esp. CCCP, 107 p. [ Links ]
D'Croz, L. and A. O'Dea. 2007. Variability in upwelling along the Pacific Shelf of Panama and implications for the distribution of nutrients and chlorophyll. Est., Coast. Shelf Sci., 73: 325-340. [ Links ]
D'Croz, L . and A. O'Dea . 2009. Nutrient and chlorophyll dynamics in Pacific Central America (Panama): 335-344. In: Lang, M.A., I.G. MacIntyre and K. Ruetzler (Eds.), Proc. Smithsonian Mar. Sci. Symp. Smithsonian Institution Scholarly Press, Washington DC. [ Links ]
Dennison, J. M. and W.W. Hay. 1967. Estimating the needed sampling area for subaquatic ecological studies. J. Paleontol., 4: 706-708. [ Links ]
Fatela, F. and R. Taborda. 2001. Confidence limits of species proportions in microfossil assemblages. Mar. Micropaleontol ., 45: 169-174. [ Links ]
Fiedler, P. C. and L.D. Talley. 2006. Hydrography of the eastern tropical Pacific: A review. Progr. Oceanogr., 69 (2): 143-180. [ Links ]
Flores, J. A. and F.J. Sierro. 1997. Revised technique for calculation of calcareous nannofossil accumulation rates. Micropaleontology, 43: 321-324. [ Links ]
Flores, J.A. y F.J. Sierro . 2007. Paleoceanography, biological proxies Coccoliths. Elsevier, Oxford. 1646 p. [ Links ]
Flores, J. A ., F.J. Sierro , G. Francés, A. Vázquez, A. and I. Zamarreño. 1997. The last 100,000 years in the Western Mediterranean: sea surface water and frontal dynamics as revealed by coccolithophores. Mar. Micropaleontol ., 29: 351-366. [ Links ]
Flores, J. A ., R. Gersonde y F.J. Sierro . 1999. Pleistocene fluctuations in the Agulhas Current Retroflection based on the calcareous plankton record. Mar. Micropaleontol ., 37: 1-22. [ Links ]
Flores, J.A ., M.A. Bárcena and F.J. Sierro . 2000. Ocean-surface and wind dynamics in the Atlantic Ocean off Northwest Africa during the last 140 000 years. Palaeogeogr., Palaeoclimatol., Palaeoecol., 161: 459-478. [ Links ]
Giraudeau, J. 1992. Distribution of recent nannofossils beneath the Benguela system: southwest African continental margin. Mar. Geol., 108: 219-237. [ Links ]
Gómez, C. e I. Martínez . 2005. Nueva productividad biológica en el Pacífico oriental ecuatorial: respuesta al aporte de nutrientes por los ríos del Pacífico colombiano, surgencia costera y polvo atmosférico. Bol. Geol., 27 (2): 100-114. [ Links ]
Hammer, Ø., D. Harper and P. Ryan. 2001. Paleontological statistics software: package for education and data analysis. Palaeontol. Electron., 4: 1-9. [ Links ]
Jordan, R. W., A. Kleijne, B.R. Heimdal and J.C. Green. 1995. A glossary of the extant Haptophyta of the world. J. Mar. Biol. Ass. UK, 75 (4): 769-814. [ Links ]
Knauss, J. A. 1960. Measurements of the Cromwell Current. Deep Sea Res., 6: 265-286. [ Links ]
López, J. C. R. y P. Costeros. 2006. Aporte de caudales de los ríos Baudó, San Juan, Patía y Mira a la cuenca Pacífica colombiana. Bol. Cient. CCCP 13: 17-32. [ Links ]
Málikov, I. y N. Villegas. 2005. Construcción de series de tiempo de temperatura superficial del mar de las zonas homogéneas del océano Pacífico colombiano. Bol. Cient. CCCP 12: 79-93. [ Links ]
Martini, E. 1971. Standard Tertiary and Quaternary calcareous nannoplankton zonation: 739-785. In: Farinacci, A. (Ed.) Proceedings of the Second Planktonic Conference, Rome, 2. Tecnosciencia, Rome. [ Links ]
McIntyre, A. and A.W.H. Bé. 1967. Modern Coccolithophoridae of the Atlantic Ocean I. Placoliths and Cyrtoliths. Deep-Sea Res., 14: 561-597. [ Links ]
McIntyre, A ., A.W.H. Bé and M.B. Roche. 1970. Modern Pacific Coccolithophorida: a paleontological thermometer. Transact. NY Acad. Sci. Ser. II, 32: 720-731. [ Links ]
Okada, H. 1983. Modern nannofossil assemblages. [ Links ]
Okada, H. and S. Honjo. 1973. The distribution of oceanic coccolithophores in the Pacific. Deep-Sea Res ., 20: 355-374. [ Links ]
Okada, H . and A. McIntyre. 1979. Seasonal distribution of modern coccolithophores in the western North Atlantic Ocean. Mar. Biol., 54: 319-328. [ Links ]
Pak, P., Jr. and V. Zaneveld. 1974. Equatorial front in the Eastern Pacific Ocean. J. Phys. Oceanogr., 4: 570-578. [ Links ]
Patarroyo, G. D. y J. I. Martínez. 2013. Foraminíferos bentónicos recientes en las aguas profundas de la Cuenca de Panamá: ecología y su posible relación con las corrientes de fondo. Bol. Invest. Mar. Cost ., 42 (1): 31-55. [ Links ]
Patarroyo, G. D . y J. I. Martínez. 2016. Paleoxigenación y paleoproductividad en el Golfo de Panamá durante el Holoceno tardío. Bol. Geol ., 38 (2): 75-92. [ Links ]
Perch-Nielsen, K. 1985. Cenozoic calcareus nannofossils: 329-426. In: H. M. Bolli, J. B. Saunders and K. Perch-Nielsen (Eds.): Plankton stratigrafphy. Cambridge University Press, Cambridge. [ Links ]
Poulton, A. J., PM. Holligan, A. Charalampopoulou and T.R. Adey. 2017. Coccolithophore ecology in the tropical and subtropical Atlantic Ocean: New perspectives from the Atlantic meridional transect (AMT) program. Progr. Oceanogr ., 158: 150-170. [ Links ]
Poveda, G. and J. Mesa. 2000. On the existence of Lloró (the rainiest locality on Earth): enhanced ocean-land- atmosphere interaction by a low-level jet. Geophys. Res. Let., 27: 1675-1678. [ Links ]
Raffi, I. and J.A. Flores . 1995. Pleistocene through Miocene calcareous nannofossils from Eastern Equatorial Pacific Ocean (Leg 138): 233-286. In: N.G. Pisias, L.A., T.R. Mayer, A. Janecek, A. Palmer-Julson and T.H. van Andel (Eds.) Proceedings of the Ocean Drilling Program, Scientific Results, 138. College Station, US. [ Links ]
Restrepo, J. 2006. Aporte de caudales de los ríos Baudó, San Juan, Patía y Mira a la Cuenca Pacífica colombiana. Bol. Cient. CCCP , (13): 17-32. [ Links ]
Restrepo, J. D. e I.D. Correa. 2002. Geología y oceanografía del delta del río San Juan, litoral Pacífico colombiano. Universidad EAFIT, Medellín. 132 p. [ Links ]
Restrepo, J. D . and S.A. López. 2008. Morphodynamics of the Pacific and Caribbean deltas of Colombia, South America. J. South Am. Earth Sci ., 25: 1-21. [ Links ]
Restrepo, J. D ., B. Kjerfve, I.D. Correa and J. González. 2000. Morphodynamics of a high discharge tropical delta, San Juan River, Pacific coast of Colombia. Mar. Geol ., 192 (4): 355-381. [ Links ]
Rodríguez-Rubio, E.J., D.M. Guerrero y J.G. Rueda-Bayona. 2008. Caracterización oceanográfica de las corrientes costeras del Pacífico colombiano (Corriente Colombia-Corriente del Chocó), una revisión actualizada. Grupo Est. Oceanogr. Fen. El Niño. Área Oceanogr. Operac., Centro Control Contam. Pacífico (CCCP-DIMAR). Tumaco, Colombia. 2 p. [ Links ]
Roth, P. H. 1983. Jurassic and Lower Cretaceous calcareous nannofossils in the western north-Atlantic (site- 534)-biostratigraphy, preservation, and some observations on biogeography and paleoceanography. Init. Rep. Deep Sea Drill. Proj., 76: 587. [ Links ]
Roth, P. H. and H.R. Thierstein. 1972. Calcareous nannoplankton: Leg 14. Deep Sea Drilling Project. [ Links ]
Saavedra-Pellitero, M., J.A. Flores , K.H. Baumann and F.J. Sierro . 2010. Coccolith distribution patterns in surface sediments of Equatorial and Southeastern Pacific Ocean. Geobios, 43 (1): 131-149. [ Links ]
Saavedra-Pellitero, M ., J.A. Flores , F. Lamy, F.J. Sierro and A. Cortina. 2011. Coccolithophore estimates of paleotemperature and paleoproductivity changes in the southeast Pacific over the past~ 27 kyr. Paleoceanography, 26: 1-16. [ Links ]
Schneider, L. J., T.J. Bralower and L.R. Kump. 2011. Response of nannoplankton to early Eocene ocean destratification. Palaeogeogr., Palaeoclimatol., Palaeoecol ., 310 (3-4): 152-162. [ Links ]
Thierstein, H.R. and J.R. Young. 2004. Coccolithophores: from molecular processes to global impact: Springer Media Verlag, Berlin. [ Links ]
Thierstein, H.R ., K.R. Geitznauer, B. Molfino and N.J. Shackleton. 1977. Global synchronicity of late Quaternary coccolith datum levels: validation by oxygen isotopes. Geology, 5: 400-404. [ Links ]
Whitlock, M. C. and D. Schluter. 2009. The analysis of biological data. Roberts, Greenwood Village, USA. [ Links ]
Wyrtki, K. 1965. Corrientes superficiales del océano Pacífico Oriental Tropical. Comisión Interamericana del Atún Tropical, 9: 295-304, p 565. [ Links ]
Wyrtki, K. 1966. Oceanography of the Eastern Equatorial Pacific Ocean. Mar. Biol . An. Rev., 4: 33-68, p 704. [ Links ]
Young, J. R., M. Geisen, L. Cros, A. Kleijne , C. Sprengel, I. Probert and J. Østergaard. 2003. A guide to extant coccolithophore taxonomy. J. Nannoplank. Res., 125. [ Links ]
Zar, J. 1996. Biostatistical analysis. Prentice Hall, New Jersey. 940 p. [ Links ]
REFERENCES
CIIFEN http://ciifen.org/index.php [ Links ]
INA http://ina.tmsoc.org/ [ Links ]
Nannotax3 http://www.mikrotax.org/Nannotax3/ [ Links ]
NASA (Visible Earth) http://www.visibleearth.nasa.gov/ [ Links ]
NOAA https://www.esrl.noaa.gov/ [ Links ]
ACKNOWLEDGEMENTS
The authors are grateful to geologist Eduardo López, from the Vice-presidency of Exploration at Ecopetrol S.A for the description of the core, and to technologist Adrián Delgado for the samples taken aboard the ARC Providencia. We would also like to thank Commander Ferrero, the crew of ARC Providencia and the group of GEMS professionals who managed the acquisition of the piston core. Special thanks to Valentina Ramírez Valencia for her valuable contributions in the statistical analyses, and to Professor Gladys Bernal and the anonymous reviewers for their contribution to the manuscript.
APPENDIX A.
The following are the coccolithophore taxa that were identified in this study.
Calcidiscus leptoporus (Murray y Blackman, 1898) Loeblich y Tappan, 1978.
Ceratolithus cristatus (Kamptner, 1950).
Coccolithus pelagicus (Wallich, 1877) Schiller, 1930.
Discoaster brouweri (Tan, 1927) emend. Bramlette y Riedel, 1954.
Discoaster pentaradiatus (Tan, 1927).
Discoaster variabilis (Martini y Bramlette, 1963).
Emiliania huxleyi (Lohmann, 1902) Hay y Mohler en Hay et al, 1967.
Florisphaera profunda (Okada y Honjo, 1973) var. profunda Okada y McIntyre, 1979.
Gephyrocapsa muellerae (Bréhéret, 1978).
Gephyrocapsa oceanica (Kamptner, 1943) McIntyre y Bé, 1967; McIntyre et al. 1970.
Helicosphaera carteri (Wallich, 1877) Kamptner, 1954 var. carteri. McIntyre y Bé, 1967.
Helicosphaera princei (da Gama y Varol, 2013).
Helicosphaera sellii (Bukry y Bramlette, 1969) Jafar y Martini, 1975.
Helicosphaera wallichii (Lohmann, 1902) Okada y McIntyre, 1979.
Pontosphaera spp. (Kamptner, 1948) exDeflandre in Deflandre y Fert, 1954; Roth, 1970.
Reticulofenestra spp. (Hay et al., 1966).
Sphenolithus abies (Deflandre in Deflandre y Fert, 1954).
Sphenolithus heteromorphus (Deflandre, 1953).
Received: November 17, 2018; Accepted: July 31, 2019