Services on Demand
Journal
Article
Indicators
-
Cited by SciELO
-
Access statistics
Related links
-
Cited by Google
-
Similars in SciELO
-
Similars in Google
Share
Boletín de Investigaciones Marinas y Costeras - INVEMAR
Print version ISSN 0122-9761
Bol. Invest. Mar. Cost. vol.48 no.2 Santa Marta July/Dec. 2019 Epub Dec 11, 2019
https://doi.org/10.25268/bimc.invemar.2019.48.2.770
Research Articles
Interactions of massive corals with turf algae and other reef organisms in Tayrona National Natural Park
1 Universidad Nacional de Colombia - Sede Caribe - Instituto de Estudios en Ciencias del Mar - CECIMAR. Atte. Invemar, Calle 25 No. 2-55, Playa Salguero. Rodadero, Santa Marta, Colombia. macgomezcu@unaledu.co.
2 Pontificia Universidad Javeriana - Departamento de Biología, Facultad de Ciencias, Laboratorio de Ictiología, Unidad de Ecología y Sistemática (UNESIS). Carrera 7No. 40-62, Bogotá D.C., Colombia. camila_gomezc@hotmail.com.
3 Universidad de Bogotá Jorge Tadeo Lozano - Sede Santa Marta, Facultad de Ciencias Naturales e Ingeniería. Carrera 2 No. 11-68, Rodadero, Santa Marta, Colombia. adolfo.sanjuan@utadeo.edu.co.
4 Universidad Nacional de Colombia - Sede Caribe - Instituto de Estudios en Ciencias del Mar - CECIMAR. Atte. Invemar, Calle 25 No. 2-55, Playa Salguero. Rodadero, Santa Marta, Colombia. szeas@unaledu.co.
In coral reefs, competition between sessile benthic organisms for access to space and other resources is a determining factor in community structuring. Recent coral mortality has favored the development of algal turfs, which are now competing with corals, often displacing them, although slowly. To determine the frequency of interactions between coral-turf and coral-other benthic categories, and how their distribution and results (coral wins or coral loses) are modulated by environmental factors, band photo transects were evaluated for 12 stations in the Parque Nacional Natural Tayrona, Santa Marta, Colombian Caribbean. It was found that algal turfs are the most frequent competitors of massive corals, and that the frequency of coral-turf interactions depends on the particular susceptibility of a coral species to the loss of tissue, and past disturbances that have affected the reef in which it lives. Furthermore, the results of these interactions depend on the ways the colonies are organized, meandroid and cerioid colonies being the most successful in competition against turfs, as their vertical growth constitutes an effective avoidance mechanism. By contrast, plocoid colonies are more prone to lose. In general, biological factors (the coral species in question and type of colonial organization) were determinants in the frequency and apparent result of coral-turf interactions, while environmental factors (disturbance gradient, depth, and degree of wave exposure) appear not to play a predominant role. As a consequence, historical and future changes in coral species cover, and the nature of colony organization in the Santa Marta area, should reflect a combination of their susceptibility to deleterious agents and their capacity to counteract or evade competition, especially with algal turfs.
KEY WORDS: Competition; Reef corals; Algal turfs; Coral reefs; Colombian Caribbean
En los arrecifes de coral, la competencia entre organismos bentónicos sésiles por acceso a espacio y otros recursos es determinante en la estructuración de las comunidades. La mortalidad coralina reciente ha favorecido el desarrollo de céspedes algales que pasan a competir con los corales, a menudo desplazándolos, aunque lentamente. Para determinar qué tan frecuentes son las interacciones coral-césped y coral-otras categorías bentónicas, y cómo su distribución y resultado (coral gana o coral pierde) son modulados por factores ambientales, se evaluaron fototransectos de banda en 12 estaciones del Parque Nacional Natural Tayrona en Santa Marta, Caribe colombiano. Se encontró que los céspedes algales son el competidor más frecuente de corales masivos así como que la frecuencia de interacciones coral-césped depende de la susceptibilidad particular de una especie de coral a perder tejido y de las perturbaciones que en conjunto afectaron el arrecife donde habita. Además, el resultado de estas interacciones dependió del tipo de organización colonial, siendo las colonias de tipo meandroide y cerioide los competidores más exitosos contra los céspedes, debido al mecanismo de evasión por crecimiento en altura, mientras que las colonias de tipo plocoide fueron más propensas a perder. En general, los factores biológicos (especie de coral y tipo de organización colonial) fueron determinantes en la frecuencia y resultado aparente de las interacciones coral-césped, en tanto que los factores ambientales (gradiente de perturbación, profundidad y grado de exposición al oleaje) parecen no desempeñar un papel preponderante. Por lo tanto, los cambios históricos y futuros en cobertura de cada especie coralina y el tipo de organización colonial en el área de Santa Marta deben reflejar una combinación entre su susceptibilidad a agentes deletéreos y su capacidad de contrarrestar o evadir la competencia, especialmente con céspedes algales.
PALABRAS CLAVE: Competencia; Corales arrecifales; Céspedes algales; Arrecifes de coral; Caribe colombiano
INTRODUCTION
Coral reefs are areas of intense competition between sessile benthic organisms (Barott et al., 2012), for access, among other things, to space (required to establish, maintain and expand territory), light, and food (Lang and Chornesky, 1990). To a large extent the result of these interspecific interactions determines the structure of the coral communities (Fricke et al., 2011; Barott et al., 2012; Gowan et al., 2014).
Competition between hard corals and benthic algae is frequent, even in healthy coral reefs (Sandin et al., 2008; Harris et al., 2015). However, the increased frequency and intensity of disturbances, particularly those of anthropogenic origin, have affected the equilibrium characterizing the natural patterns of these interactions, favoring algae and, in particular, algal turfs.
Algal turfs are mixed assemblages of algae whose canopy does not exceed 2.0 cm (Connell et al., 2014), and which accumulate sediments in their fronds (Gorgula and Connell, 2004). They have the potential to limit the settlement of coral larvae (Steneck, 1997; Birrell et al., 2005) and other canopy-forming algae (Kennelly, 1987; Airoldi, 2003). They may, in addition, affect the recovery of damaged coral tissue (Alvarado and Acosta, 2009) and lead to progressive die-off of adjacent coral tissue (Jompa and McCook, 2003a, 2003b; Roy, 2004; Harris, 2015). They therefore constitute the principal competitors of hermatypic corals (Bruggemann et al., 1994; Ledlie et al., 2007; Barott et al., 2012; Hamilton et al., 2014; Harris, 2015). In fact, they promote a phase shift, in which reefs previously dominated by corals are taken over by algae, in particular in perturbed environments (Barott et al., 2009, 2012; Haas et al., 2010).
Coral-turf interactions occur when previously existing damage is caused by physical agents (storms, sedimentation, high temperatures, pollution, between others) and/or biological factors (epizootics, depredation, between others) (Rogers et al., 1982; Bythell et al., 1993; Díaz-Pulido and McCook, 2002; Alvarado and Acosta, 2009), generate partial mortality in colonies, stripping tissue from coral skeletons and exposing them to rapid colonization by these algal turfs, which, once established, begin to interact with living coral tissue on the boundary (McManus and Polsenberg, 2004; Fricke et al., 2011; Gowan et al., 2014). These interactions are complex and variable over space and time, as they depend on the species involved, both algae and corals (Titlyanov et al., 2007; Birrell et al., 2008) and the environmental circumstances in which they occur (Vermeij et al., 2010; Barott et al., 2012). However, because of the difficulty of identifying the species of algae involved, turfs have traditionally been evaluated in generalized terms, as a unique functional group (Connell et al., 2014).
The proliferation of turfs and of interactions with corals is particularly evident in large-scale events that cause mass mortality, such as hurricanes, epizootics or coral bleaching (Rogers et al., 1982; Bythell et al., 1993; Díaz-Pulido and McCook, 2002), but it also occurs in the presence of small-scale chronic or episodic mortality in individual colonies, and might not be noticed over short time periods (Alvarado and Acosta, 2009). Because of its scale and its environmental, social and economic implications it is important to quantify mass and chronic damage to corals and to monitor its consequences, processes in which algal turfs can play a major part. However, globally, few studies have examined the relation between these non-calcifying assemblages and global climate change (Johnson et al., 2017), while for the Caribbean only a few works have argued that the proliferation of turfs is detrimental to reefs, as a direct cause of the mortality of hard corals (Roy, 2004; Gómez-Cubillos, 2018). This demonstrates profound gaps in our knowledge concerning the important environmental role played by algal turfs and of their interactions in the processes involved in the structuring of reef communities (Fricke et al., 2011; Barott et al., 2012) and, therefore, in the potential paths towards recovery of the disturbed reefs of the Colombian Caribbean.
Recognition of the ecological importance of competition for space in the coralline benthos and an acceptance that reefs are facing change scenarios of differing scales, leads to a need to evaluate the spatial dynamics of coral-turf interactions. Thus, this study examines the frequency of coral-turf interactions (in relation to other interactions), the apparent results of this competition (coral wins or coral loses), and how certain biological and environmental factors modulate their frequency and their results. The approach represents and attempt to understand how important the effects of these processes have been, are, and might be in the future, for coral communities. The research was carried out in in the Tayrona National Natural Park (Tayrona NNP) in the Colombian Caribbean, an area that is subject to a dramatic alternation between coastal upwelling and continental runoff (Zea, 1993; Bayraktarov, 2013; Bayraktarov et al., 2014a, 2014b), and whose history of disturbance caused by different natural and anthropogenic pressures have produced significant losses in coral coverage in recent decades (see the compilation of Friedlander et al., 2014) and, as a consequence, the exposure of coral skeletons that are apt for colonization by turfs.
This spatial evaluation, carried out at the scale of the reef (km), provides a snapshot in time (Barott et al., 2012), establishing a baseline that will contribute to an understanding of the balance, recovery and future of the reef communities of the NNP Tayrona.
MATERIALS AND METHODS
Study area
In the region of Santa Marta and the PNN Tayrona (Fig. 1, 11° 12'- 11° 22' N and 73° 57'- 74° 15'W), the coral reefs that border the rocky coastline are reduced in scale, constituting fringing reefs found at medium depths (between 8-10m down to 20-30 m), and are principally composed of massive hemispherical corals. Patch reefs that break the surface are found only in the interior of the bays (Werding and Sánchez, 1989).
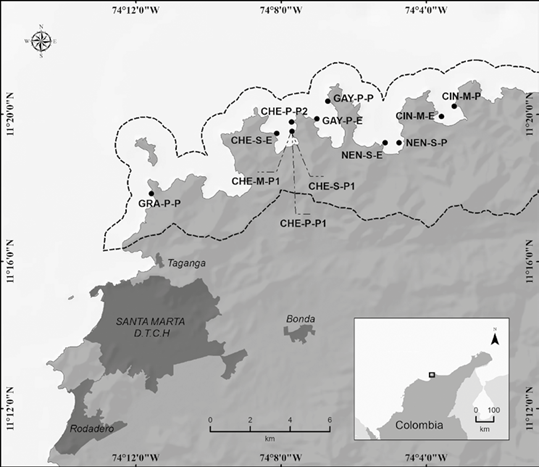
Figure 1 Location of the 12 sampling stations in the PNN Tayrona, Colombian Caribbean. The dotted line marks the limits of the protected area. The nomenclature of each station corresponds to the three initial letters of the bay (GRA-Granate, CHE-Chengue, GAY-Gayraca, NEN-Nenguange and CIN-Cinto), a letter denoting depth (shallow-S, medium-M and deep [deep in Spanish]-P), and another the relative level of exposure to wave action (exposed-E and protected-P). Source: prepared by Felipe Valencia, GIS specialist.
Given the geographical orientation of the coast and the direction of the prevailing winds (north westerly trade winds), the western shores of the bays are more exposed to wave action, leading to a greater preponderance of crustose hard coral formations, while on the protected eastern sides large massive forms abound and the coral formations are more complex and diverse (Werding and Sánchez, 1989; Díaz et al., 2000; Martínez and Acosta, 2005; Vega-Sequeda et al., 2008; Bayraktarov et al., 2014a). However, despite the large number of solid platforms available for the establishment of reefs, the coral formations in the Santa Marta area are not well developed, their growth being limited in comparison with other parts of Colombian Caribbean, because of the powerful influence of a bimodal climatic regime, which oscillates between periods of continental runoff and coastal upwelling (Zea, 1993).
During the rainy season (May to November) surface water temperatures are high (27-29°C), salinity declines (34) and the sediment load caused by continental runoff increases water turbidity, while in the dry season (December to April), the coastal upwelling caused by the northeasterly trade winds provokes a fall in sea temperatures (to 20-25 °C), salinity levels rise (38), along with wave intensity, and in general the waters become clearer (Ramírez, 1983; Zea, 1993; Franco-Herrera, 2005; Vega-Sequeda et al., 2008; Bayraktarov et al., 2014b). This climatic variability determines algal composition, generating in particular a great proliferation of macroalgae between January and April, which subsequently declines or disappears with the increase in water temperatures between September and November (Bula-Meyer, 1990), leaving the hard substrates that have not been colonized by macroinvertebrates covered in algal turfs and crustose coralline algae (Díaz-Pulido and Garzón-Ferreira, 2002).
Benthic coverage, the frequency of interactions between hard corals and other benthic categories and the apparent results of coral-turf competition
Field data was gathered in October 2016, during the rainy season, when macroalgal levels were low or non-existent and algal turfs predominated. In total, 12 stations were evaluated (Figure 1), in five bays of the PNN Tayrona, comparing the protected (eastern) and the exposed (western) sides at three depth levels, if they were to be found: shallow (2 to 6 m), medium (7 to 12 m) and deep (> 12 m).
In each station, a flexometer was placed on the floor of the bay, between two and four belt transects (10 x 2 m) being evaluated. The permanent transects developed by the Sistema Nacional de Monitoreo de Arrecifes Coralinos de Colombia (SIMAC) were used for reference purposes (Garzón-Ferreira et al., 2002; Garzón-Ferreira and Rodríguez-Ramírez, 2010).
The composition of the benthos was determined using the photoquadrat method (Preskitt et al., 2004). A 50 x 50 cm (0.25 m2) 1" PVC quadrat divided into 25 grid squares was placed at meter intervals, alternating on the right and the left side of the tape (Gowan et al., 2014), resulting in a total of 2.5 m2 being evaluated per transect. Using a Canon Powershot G16 digital camera enclosed in a watertight case, photographs were taken of the whole of the quadrats and of constituent sections, in order to gather more detailed information.
The photographs were processed using the ImageJ program. For each quadrat the number of populated grid squares was counted, covered area estimated (in cm2), and the perimeter of each of the categories described by Caricomp (2001) and Garzón-Ferreira et al. (2002) measured (cm), namely: hard corals (by species), soft corals (gorgonacea and zoanthids), milleporidae, algae (fronded, turfs and crustose), sponges (erect and incrussting) and the abiotic substrate (sand and debris). Subsequently, the percentage coverage of each benthic category and coral species was calculated according to the proportion between the area occupied by each category/species and the overall total categories/species.
In order to estimate the frequency (%) of coral-turf and coral-other-benthic-category interactions both overall and per station, the number of interactions per grid square was counted, and the perimeter of each interaction measured (cm). Frequency (%) was calculated on the basis of the percentage relation between the total number of grid squares occupied by each kind of interaction and the total number of grid squares in each quadrat (n = 25). The same method was used to calculate the frequency (%) of coral-turf interactions per coral species and type of colony organization, determined by the shape of the corallite and the structure of the corallum (Ogilvie, 1896; Reyes et al., 2010). In order to understand how representative this frequency measure (%) is compared to all the other possible ways a coral species might interact with a turf, an occurrence index (%) was calculated, based on the percentage relation between the total number of grid squares in which a coral species interacted with turfs and the overall number of grid squares occupied by the same species. In addition, the percentage relation between the perimeter of the area occupied by the coral-turf interaction of a single coral species and the overall perimeter of the species found in the quadrats was calculated.
The apparent result of the coral-turf interaction was determined by examining the different coral species and patterns of colony organization, according to the suggestions made by Barott et al. (2012), Márquez and Zea (2012) and Gowan et al. (2014): a) coral loses when there is visible damage to the coral tissue, (changes to coloration, loss of tegumentary continuity and necrosis) caused by smothering, light deprivation or direct contact with the turf; b) coral wins when the rim polyps form elevations, reminiscent of lips, skirts or mounds that evade interaction, and c) the result is apparently neutral when the coral tissue and the turf meet at the same level (at a 180° angle) and no deterioration of the polyps is observed at the interaction frontier.
Analysis of the information
Representativeness using coverage data (%) for reef-forming species (hard corals and milleporidae) was evaluated by station and by sample (transect) employing Shannon-Wiener's index of average accumulated diversity (H') applying the appropriate standard deviation, and using the EstimateS program, version 9.1 (Colwell, 2013), with 1,000 random permutations to determine whether the curve reached asymptote, if the variation coefficient of the final five values was below 5% and if the slope of the linear regression differed significantly from zero. This procedure was used to determine that, in the Santa Marta area, 3.5 m2 - equivalent to 1.5 transects - constitute a representative sample of the benthic reef-forming community. Consequently, for each of the analyses, all the transects in each station were combined in order to obtain a single data point for each variable, at each station.
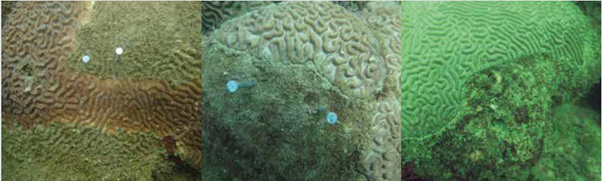
Figure 2 Apparent result of coral-turf interactions, with reference to colonies of Pseudodiploria strigosa: a) coral loses, b) coral wins and c) apparently neutral interaction. Photos: Sven Zea.
The differences in the frequency of coral-turf interactions and interactions between coral and other benthic categories between bays and stations, and the effects of environmental factors (perturbation gradient, depth of the water column, and degree of wind-wave exposure) on the frequency of these interactions were evaluated using contingency tables and Chi2 independence test with a confidence level of 95 % (Sokal and Rohlf, 1981).
Assuming that the coverage of algal turfs is greater in reef areas disturbed by human activity (Barott et al., 2012; Harris et al., 2015), a perturbation gradient was defined (NE-SW), starting from the station closest to the Santa Marta area of urban and port development in the SW, with the assumption that disturbance effects decrease in intensity and effect towards the PNN Tayrona in the NE (Werding and Sánchez, 1988; Zea, 1994, Bayraktarov and Wild, 2014). The effects of this factor were examined at only four stations, which shared similar characteristics (deep, and protected), in order to prevent depth and exposure factors from masking the effects of the gradient. Subsequently, on the assumption that algal turf development is stronger in shallow waters and in protected zones (Fricke et al., 2011), data from all 12 stations were used to evaluate the effects of depth levels (shallow, medium and deep) and by degree of exposure (exposed and protected) for each depth level.
To determine the overall frequency and results (coral wins, coral loses or apparently neutral) of coral-turf interactions by the coral species involved and the nature of colony organization, only data was analyzed only for the 10 coral species for which more than 30 interactions were registered. The frequency of the interactions was measured using One Way ANOVA, and the results for competition analyzed using Chi2 independence tests (Sokal and Rohlf, 1981).
In order to establish whether coverage (%) is a covariate of the perimeter occupied by coral-turf interactions (%) - assuming a priori that the greater the coverage of coral, the greater the perimeter of the coral-turf interaction - a separate Pearson correlation was performed for the perimeter and coverage of each coral species. Subsequently, multi-factor ANOVA (Agresti, 2002) was used to evaluate whether the perimeter for each coral species varied according to the selected environmental factors. The perimeter and coverage were transformed using the arcsine formula (Sokal and Rohlf, 1981) and the homogeneity of variances using the Levene test (Underwood, 1997).
Finally, binary regression models (De la Source, 2011; Guisande et al., 2013) were used to evaluate the effects of environmental factors (gradient, depth and exposure) on the results of coral-turf interactions, differentiated by coral species and type of colony organization. For this purpose, the response variable (result) was converted into binary (01), zero (0) representing coral loses and one (1) coral-does-not-lose lose (a category that included all the cases in which the coral won or the interaction remained neutral); the independent factors were converted into dummy variables.
RESULTS
Interactions between corals and other benthic categories
4,010 interactions between hard corals other benthic categories were identified in the 70.3 m2 and the 4,053 grid squares occupied by hermatypic corals. Coral-turf (58.7 %) and coral-crustose algae interactions (13.9 %) were the most frequent. The remaining 18.7 % of interactions occurred between hard corals and other species - namely milleporidae, frondy macroalgae, sponges and soft corals -while only 8.7 % of corals had no interaction with any other benthic organisms (Figure 3). These results having been produced, the following analyses were carried out, only three kinds of interaction being examined, namely coral-turf, crustose coralline algae and coral-other categories.
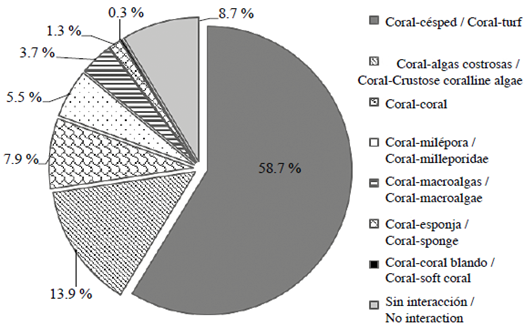
Figure 3 Overall frequency of interactions (%) between hard corals and other benthic categories in reefs in the PNN Tayrona.
The frequency of interactions between corals and other benthic categories varied between bays (Chi2 = 191.3; P < 0.05; GL = 8) and between stations (Chi2 = 423.1; P < 0.05; GL = 22). The overall tendency was, except in stations CHE-S-P and GRA-P-P, that coral-turf interactions were the most frequent (> 56 %), followed by coral-other categories and crustose coralline algae interactions. In CHE-S-P the dominant interaction was between coral and crusty algae (47.7 %), while in GRA-P-P, although coral-turf interactions dominated, crustose coralline algae interactions were more frequent than those between coral and other benthic categories (Figure 4).
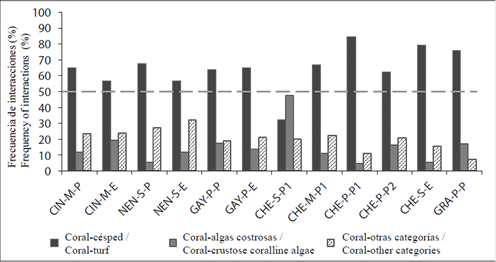
Figure 4 Frequency of interactions (%) between corals and other benthic categories in the 12 sample stations of the PNN Tayrona. The dotted line indicates the 50% cut-off. For abbreviations see Figure 1.
It was also determined that the frequency of interactions depends on the location of the station along the proposed perturbation gradient (Chi2 = 25.2; P < 0.05; GL = 4), coral-turf interactions being most frequent in the reefs that lie closest to Santa Marta Bay (Granate) than in those that are farthest away (Gayraca), while interactions between coral and other benthic categories tend to diminish towards the SW, crustose coralline algae interactions remaining constant (~15 %) (Figure 5a).
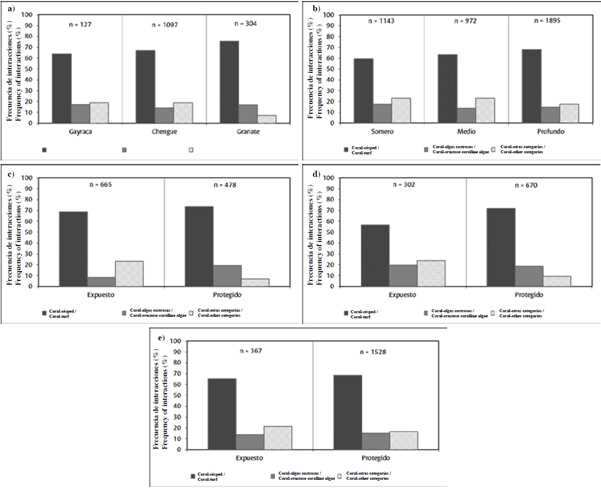
Figure 5 Frequency of interactions (%) between benthic categories: a) in deep-protected stations representing different sites on the perturbation gradient NE-SW; b) between depth levels; c) between levels of exposure in shallow stations (2-6 m); d) between levels of exposure in medium-depth stations (7-12 m) and e) between levels of exposure in deep stations (> 12 m).
The independence tests showed that the frequency of interactions varies between depth levéis (Chi2 = 29.3; P < 0.05; GL = 4). However, contrary to what was expected, in the PNN Tayrona, coral-turf interactions tend to increase with depth, while coral-other benthic categories and crustose coralline algae interactions were slightly more frequent in shallow environments (Figure 5b). Individual analyses by depth level showed that in shallow (Chi2 = 142.5; P < 0.05; GL = 2; n = 4;) and in medium depth stations (Chi2 = 54.3; P < 0.05; GL = 2; n. = 3), the data and evidence gathered suggest that the frequency of interactions is better explained by the degree of exposure to wind and wave action: coral-turf interactions being more frequent, especially in the protected parts of bays, while interactions between coral and other categories increased in exposed areas and crustose coralline algae interactions remained constant (~ 19 %), except in shallow, exposed, stations, where their frequency did not exceed 8 % (Figure 5c, d). By contrast, in the deep stations (Chi2 = 4.5; P > 0.05; GL = 2; n = 5), the frequency of interactions was unrelated to the level of exposure, the occurrence of the three types of interaction evaluated being similar in protected and exposed station alike (Figure 5e).
Coral-turf interactions
The 2578 coral-algal turf interactions identified involved 16 species of hard corals. Significant differences were found in the frequency of interactions between coral species for the 10 species selected that had more than 30 interactions with turfs (ANOVA, F = 4.9; P < 0.05).
The species Pseudodiploria strigosa (24.2 %), Colpophyllia natans (15.9 %) and Montastraea cavernosa (13.1 %) had the highest number of borders interacting with algal turfs, while Diploria labyrinthiformis (2.6 %), Orbicella annularis (1.7 %) and Meandrina meandrites (1.3 %) had the lowest (Figure 6).
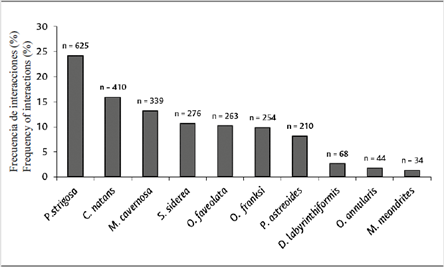
Figure 6 Frequency of interactions (%) with turfs, differentiated by coral de coral species (relative to total interactions with turfs).
The results of the occurrence index showed that Orbicella franksi (85.8 %), Porites astreoides (77.5 %) and M. cavernosa (64.2 %) are the species with the highest proportion of grid squares occupied by interactions with algal turfs, while D. labyrinthiformis (38.6 %) and O. annularis (44.9 %) have the lowest proportion of living tissues in similar contact (Figure 7a).
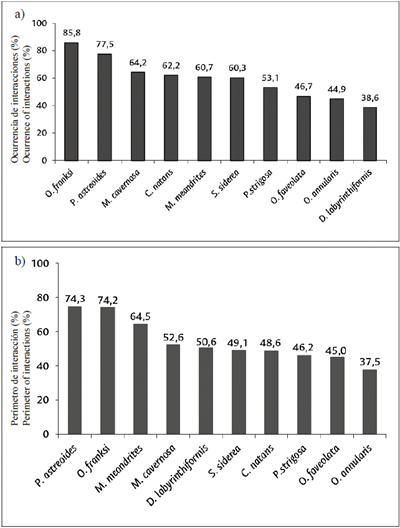
Figure 7 a) Occurrence index of interactions (%) with algal turfs, differentiated by coral species; b) perimeter occupied (%) by coral-turf interactions.
According to the perimeter (%) occupied by coral-turf interactions, differentiated by coral species, the results were similar to those of the occurrence index, showing that P. astreoides (74.3 %) and O. franksi (74.2 %) are the species with the greatest percentage of their perimeters exposed to turfs, while O. annularis (37.5 %) has the lowest (Figure 7 b).
For none of the 10 species evaluated was there a significant correlation between coverage (%) and perimeter (%) occupied by coral-turf interactions. This shows that coverage is not a good indicator for predicting the frequency of coral-turf interactions.
The multi-factor ANOVA showed that, of the 10 selected coral species, only in C. natans and P. astreoides did the perimeter (%) occupied by coral-turf interactions vary significantly as a result of the selected environmental factors (Table 1). In C. natans the perimeter in interaction with turfs varied with the three factors (gradient, depth and exposure), and there was significant interaction between exposure and gradient: that is, that the differences between gradients varied with exposure. Taken together, these results indicate that for these species the perimeter (%) of coral-turf interaction was greater in shallow waters with higher levels of anthropogenic influence (Table 2). In the case of P. astreoides, although the ANOVA indicates that the perimeter in interaction with turfs varies significantly with depth and gradient, the increase of perimeter with depth was only slight (Table 2). The results of this analysis suggest that in general the environmental factors studied are not decisive in explaining the frequency of coral-turf interactions in the area of study.
Table 1 Results of the Pearson correlations and multi-factor ANOVA for determining the effect of environmental and biological variables on the frequency of coral-turf interactions, differentiated by coral species. The numbers in bold are significant values (P < 0.05);
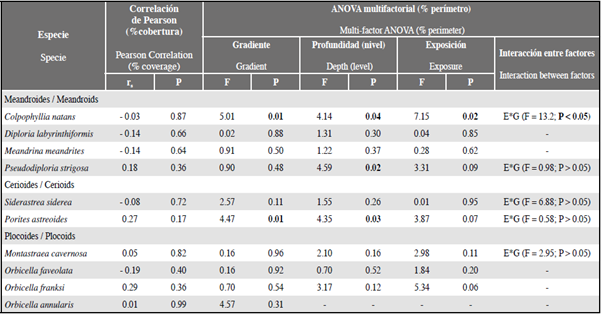
Apparent results of coral-turf competition
Of the total number of identified coral-turf interactions (n = 2578), it was established that in 37.5 % of the cases coral won, in 30.4 % it lost and in 32.2 % the interactions remained apparently neutral. The data for the 10 selected coral species (n. = 2523) established that the results of the interactions vary according to the coral species in question (Chi2 = 168.7; P < 0.05; GL = 18). Figure 8 shows that in the case of D. labyrinthiformis and O. annularis the coral won comprehensively. In the cases of P. strigosa, C. natans and P. astreoides the proportion of coral wins and neutral results was similar, but always greater than coral loses. For M. meandrites and O. faveolata neutral interactions predominated, while for M. cavernosa and O. franksi coral loses predominated and for S. siderea the proportion between coral loses and coral wins was similar.
Additionally, the results of coral-turf interactions depended on the nature of colony organization (Chi2 = 55.0; P < 0.05; GL = 4). When all the data were combined by colony type (independently of species), coral wins were greater in colonies that included meandroid and cerioid forms, although coral loses were more frequent than neutral results, while in meandroid colonies neutral results were greater than coral loses. By contrast, in colonies containing plocoid forms, the coral loses result dominated, followed by neutral, and coral wins (Figure 9).
The binary regression models for the results of the coral-turf interactions (0 = coral loses, 1 = coral-does-not-lose), differentiated by kind of colony organization and the selected environmental variables, were statistically significant (meandroids: Chi2 = 11.7; P < 0.05; cerioids: Chi2 = 18.6; P < 0.05 and plocoids: Chi2 = 18.1; P < 0.05) (Table 3).
Table 3 Binary logistic regression to predict the result of coral-turf competition (coral loses and coral-does-not-lose) for type of colony organization and coral species, in function of the factors gradient, depth and exposure to wave action. The numbers in bold are significant values (P < 0.05); R2 = percentage of data classified in the model; Chi2 test of independence of the accuracy of the model; de Wald Chiw 2 for multivariate samples; B = correlation coefficient for the model.
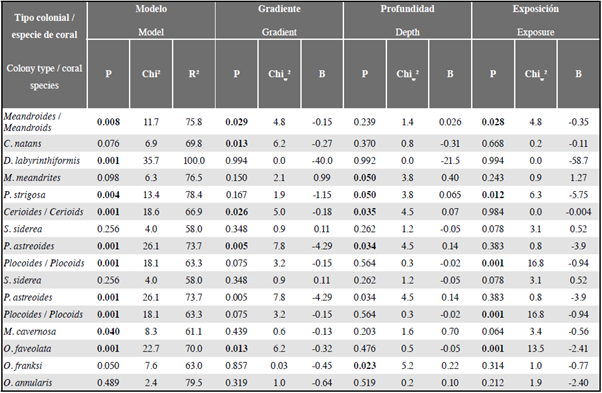
In meandroid colonies gradient and exposure were significant (P < 0.05), and their coefficients of correlation (exposure: -0.35, gradient: -0.15) indicate that the possibility of meandroid coral loses increases with less exposure to wave-action and with distance from Santa Marta. For cerioid colonies gradient and depth were significant, and their coefficients (gradient: -0.18 and depth: 0.07) indicate that the probability of loss increases the closer they are to Santa Marta and at greater water column depth. In plocoid colonies only exposure was significant, its coefficient (-0.94) suggesting that the probability of loss increases with less exposure to wave action (Table 3).
When this analysis, differentiated by coral species, was conducted it was found that, of the selected species, the models were statistically significant (P < 0.05) for six (D. labyrinthiformis, P. strigosa, M. cavernosa, O. faveolata, O. franksi and P. astreoides), while in the case of C. natans, M. meandrites, O. annularis and S. siderea it cannot be said that the result of the competition can be predicted on the basis of the selected variables (Table 3).
Depth was the most important variable in the case of P. strigosa, O. franksi and P. astreoides, whose coefficients (0.06, 0.22 and 0.14, respectively) indicate that at greater depth there is an increased possibility that the species will lose. The perturbation gradient was the most important variable for O. faveolata and contributed to the model for P. astreoides, and its coefficients (-0.32 and -4.29, respectively) indicate that the probability of loss is greater at less distance from Santa Marta. Exposure to wave action contributed to the models for O. faveolata and P. strigosa, and their coefficients (-2.41 and -5.75, respectively) suggest that less exposure increases the probability of loss. In the case of D. labyrinthiformis and M. cavernosa, although the model was significant, no variable was capable of explaining the result of the competition (Table 3).
However, for this analysis, in none of the 13 cases evaluated did Nagelkerke's R2 exceed 27 %, and the quotient between the probability that the event defining the dependent variable and the probability that it does not occur in the presence or absence of the factor, was in every case below 3 %. Therefore, these models, though statistically significant, only explain a small percentage of the variance of the dependent variable (coral loses and coral-does-not-lose), for which reason it is assumed that the selected factors are not good predictors of the results of coral-turf competition.
DISCUSSION
Interactions between corals and other benthic categories
In the reefs found in the PNN Tayrona, hermatypic corals interact with algae (turfs, macroalgae and crustose coralline species), others (massive, Milleporidae and soft corals) and sponges, the most frequent interactions being between coral-algal turf and crustose coralline algae (58.7 % and 13.9 % respectively).
In order to interpret these results correctly it should be borne in mind that coral-turf interactions generally occur when physical and/or biological agents produce a prior lesion in the coral tissue, which caused partial mortality in the colonies (Rogers et al., 1982; Bythell et al., 1993; Díaz-Pulido and McCook, 2002; Alvarado and Acosta, 2009), leaving behind the exposed skeleton, which is then colonized by turfs (McManus and Polsenberg, 2004; Fricke et al., 2011; Gowan et al., 2014). Therefore, the fact that coral-turf interactions are the most frequent reflects the residual effect of the perturbation gradient to which the reefs have been exposed (Hughes and Connell 1999). This history includes events such as the mass mortality of the sea urchin Diadema antillarum in the 1980s (Lessios et al., 1984), mass bleaching events in 1987, 1995, 2005 and 2010 (Navas-Camacho et al., 2010), hurricanes (Johan in 1988, Lenny in 1999 and Matthew in 2016, immediately before the fieldwork for this study was carried out), coral diseases such as white band in Acropora spp. (Gladfelter, 1982; Aronson and Precht, 2011) and aspergillosis in Gorgonia spp. between 1985 and 1988 (Garzón-Ferreira and Zea, 1992), the impact of invasive species such as Pterois volitans (Acero et al., 2019), and overfishing (Friedlander et al., 2014). These disturbances have led to algal turfs currently being the principal competitors of hard corals in the PNN Tayrona, a trend that has been reported previously for other tropical reefs (Hughes, 1994; Díaz-Pulido and McCook, 2002; Littler et al., 2006; Hughes et al., 2007; Sandin et al., 2008; Fricke et al., 2011; Barott et al., 2012; Harris et al., 2015).
For its part, the low frequency of interactions between coral and crusty algae, species that are important to the building and calcification of reefs (Heyward and Negri, 1999; Vermeij et al., 2010; Gómez-Lemos and Díaz-Pulido, 2017), might respond to effects derived from the synergistic action of global perturbations, such as the acidification of the oceans (Cyronaka and Eyrea, 2016) and at local level to factors such as the diminution in the population of herbivores (Burkepile and Hay, 2010), factors that limit the formation of crusty algae and, in consequence, the probability that they will interact with other benthic organisms.
Although coral-turf interactions are the most common kinds of interaction in the Tayrona National Natural Park, differences were found in their frequency between bays and stations. For example, in CHE-S-P the crustose coralline algae interaction dominated (47.7 %), perhaps because this station is made up principally of large heads of massive corals with few dead areas, a fact that suggests the level of impact exerted by perturbation affects each reef differentially, according to the intensity, periodicity and proximity of the event in question (Sousa, 1979; Connell, 1997).
Given that the environmental context plays a decisive role in the dynamics of interactions in the benthos (Airoldi et al., 1995; Burkepile and Hay, 2010; Vermeij et al., 2010; Barott et al., 2012; Swierts and Vermeij, 2016), in this research it was found that coral-turf interactions tend to be more frequent in reefs that are closer to Santa Marta, an area of urban and port development, while along the SW-NE axis that leads away from Santa Marta, interactions between coral and other benthic categories diminish. This suggests the existence of a major anthropogenic perturbation affecting corals (Zea, 1994), caused by a reduced population of herbivores, and increased levels of nutrients and sediments in the water (Gorgula and Connell, 2004; Barott et al., 2012), conditions that are prejudicial to coral species and increase the loss of reef calcifiers (Díaz-Pulido and McCook, 2002; Barott et al., 2012; Bender-Champ et al., 2014), but that are beneficial to the algal turfs, which are able to take advantage of the situation.
On the matter of depth, Fricke et al. (2011) demonstrate experimentally that, in reefs in Curacao, algal turfs are more abundant in shallow waters. However, in this research the frequency of coral-turf interactions increased with depth while coral-other categories interactions diminished, indicating not only that at depths of up to 15m light is not a factor that limits the development of algal turf assemblages, although their composition does vary (Gómez-Cubillos, 2018). Furthermore, the partial mortality of coral tissue in the PNN Tayrona has been greater in deeper waters (> 12 m), particularly during bleaching events or epizootics (Acosta et al., 2018). The accumulation of sediments as a result of resuspension also increases with depth (Bayraktarov, 2013; Bayraktarov et al., 2014a), permitting them to accumulate in the turf fronds in greater concentrations and, as a result, increase the damaging effects of these assemblages when they come into contact with coral tissues.
For the factor exposure to wave action, it was found that coral-turf interactions are more common in protected areas with depths less than 12m, while in exposed areas, although coral-turf interactions also dominated, the proportion of coral-other categories interactions increased. In deep waters ( > 12 m ), by contrast, no difference was found in the frequency of interactions between exposed and protected areas. These results suggest that in areas protected from wave action, with a depth of less than 12 m, and where the effect of turbulence is less (Bayraktarov, 2013; Bayraktarov et al., 2014a) and the sediment load increases (Roy, 2004), the effects on corals have been greater. These circumstances favor the development of algal turfs, as the algae trap and retain sediment particles along with detritus in their fronds, and they can then use this material to anchor their holdfasts and grow laterally (Airoldi and Virgilio, 1998; Connell et al., 2014), displacing coral tissues as a result.
Coral-turf interactions
In overall terms it was found that in the majority of the coral species the selected environmental factors do not exert a significant influence on the frequency of interactions. Furthermore, it was shown that the coverage (%) of a species is not a covariate of the perimeter that is in interaction with turfs, undermining the hypothesis the greater or lesser the coverage of coral, the greater or lesser the perimeter/frequency of interaction.
These results, alongside the premise that coral-turf interactions usually occur in the wake of the death of coral tissues caused by the actions of physical and biological agents (Rogers et al., 1982; Bythell et al., 1993; Díaz-Pulido and McCook, 2002; Alvarado and Acosta, 2009), permit the suggestion to be made that the current frequency of coral-turf interactions is indirect evidence of the particular susceptibility of each coral species to suffering lesions as a result of a combination of perturbations that affect the habitat in which that colony is found. This complements the hypothesis of Meesters et al. (1996) according to which susceptibility to partial mortality is specific to each coral species, a tendency already described by Ott and Lewis (1972), who found that in the reefs of Barbados the frequency of attack by fireworms (Hermodice carunculata), is greater in colonies of P. astreoides than in P. strigosa.
Apparent results of coral-turf competition
Of the total number of identified coral-turf interactions, corals appear to win in 37.5 % cases, lose in 30.4 % and draw in 32.1 %. This suggests that in the reefs of the PNN Tayrona a small-scale process exists in which minor lesions are repaired, and in which coral wins and coral loses, meaning that a balance is maintained between the different potential outcomes of these interactions. However, when only the 10 coral species of interest are examined it is apparent that the results of interactions vary with the coral species involved. This coincides with the results of other studies that confirm that coral-turf interactions are highly variable, both in terms of process and results (Jompa and McCook, 2003a), but that the result depends in particular on the mechanisms of competition and the morphology and physiology of the species involved (Díaz-Pulido and McCook, 2002; Jompa and McCook, 2002; Titlyanov et al., 2007; Barott et al., 2012; Corado-Nava et al., 2014; Swierts and Vermeij, 2016). Furthermore, the effects of gradient, depth and exposure on the result of competition, when differentiated by colony organization type and coral species, showed that in this case these selected factors are not reliable predictors of the apparent result of coral-turf competition. This contrasts with the results reported by Airoldi et al. (1995), who concluded that the characteristics of the physical environment in which the competition occurs were relevant.
When the results of coral-turf interactions are analyzed according to type of colony organization, and with the proviso that each component species responds in a different way, it was found that in meandroid and cerioid colonies coral wins was the most frequent result, while in plocoid structures, particularly with M. cavernosa, coral loses predominated. This confirms that the type of colony organization can be a partially decisive factor in deciding the results of the competition. For this reason, it appears to be the case that the corals present in different types of colony organization use a similar range of competition mechanisms in their border interactions with algal turfs and that the ones they use depend on the kinds of perturbation affecting their habitat. The effectiveness of these strategies depends on the capacity for regeneration of the damaged tissue in relation to the size of the lesion (Meesters et al., 1996) and the specific growth (or evasion/escape) rates of each species (Lang and Chornesky, 1990).
The possible competition mechanisms involved in coral-turf interactions are yet to be discussed. Thus, the justification of the hypothesis set out above is based on an analysis of the different mechanisms corals employ in their inter-species interactions with other corals (Lang and Chornesky, 1990; McCook et al., 2001), other benthic organisms (Richardson et al., 1979; Karlson, 1980; Chornesky, 1983; López-Victoria et al., 2006) and macroalgae (McCook, 2001; McCook et al., 2001; Nugues et al., 2004), stressing the fact that coral-turf interactions occur at very small scales (mm), given that the contact surfaces offered by the turf algae are minimal.
Corals have developed various physical and chemical mechanisms to defend themselves directly against their competitors (Lang and Chornesky, 1990). A first mechanism involves avoidance of competition through vertical growth (Meesters et al., 1996; López-Victoria et al., 2006), which raises a coral above its competitors in the form of a dome, roof, or lip, impeding their direct action and lateral growth.
The most important of the mechanisms of direct confrontation described in the literature are: extracelenteric digestion, in which mesenterial filaments and sweeper tentacles are used to abrade, sting or destroy enemy soft corals, the production and release into the surrounding seawater of chemical substances that injure opponents that are beyond physical reach, and the secretion of mucus (Richardson et al., 1979; Karlson, 1980; Schoener et al., 1983; Lang and Chornesky, 1990; McCook, 2001; Jompa and McCook, 2002).
In the Caribbean, corals with digestive dominance are the most abundant (Maguire and Porter, 1977). These are generally massive slow-growing forms, with large and small polygonal plocoid and cerioid polyps (Lang and Chornesky, 1990), inasmuch as meandroid corals generally avoid competition by growing upwards (Barnes, 1973; Meesters et al., 1996). This was evident in the case of P. strigosa in this study and in other unpublished histological observations, which produces growth roofs and lips that rise slightly above the turfs and other organisms, tending to cover them and in this manner avoid the negative effects of competition. This vertical growth mechanism is used to regenerate small lesions, since once an area of the colony has been damaged the tissue surrounding the lesion grows inwards in a process of evagination in order to repair the wound (Meesters et al., 1996). This process was described by Roy (2004) for colonies of S. siderea exposed to conditions with high sediment loads in reefs in Mexico, and by Meesters et al. (1992) for reefs in Curacao in colonies of P. strigosa. However, particularly in cases of very large lesions, the process of tissue regeneration may remain incomplete, leaving the damaged areas available for turfs to establish themselves and continue their advance. However, the more resistant species of meandroids, such as Meandrina meandrites and Mycetophyllia spp. have developed a greater regeneration potential, in order to reduce the damage caused by superficial and peripheral interactions (Meesters et al., 1996).
In sum, in frequently disturbed reefs, a strategy to evade coral-turf competition through vertical growth is more successful than strategies based on extracelenteric digestion (Maguire and Porter, 1977). This argument helps explain why in the PNN Tayrona the meandroid and cerioid colonies (with some exceptions in the interior of each kind of colony, e.g., O. annularis in the case of plocoids and M. meandrites for meandroids) presented a larger number of coral-does-not-lose interactions (wins + neutral), because they use the mechanism. However, the increase in the frequency and intensity of the perturbations, could have the effect of limiting recovery times and the ability of species to defend themselves, leading to future changes in the structure of the coral communities. This was documented by Loya et al. (2001) in the reefs off the southern coast of the Japanese island of Sesoko, where, following an episode of mass bleaching, species with more successful competitive strategies predominated.
This study contributes to an improved understanding of coral-turf interactions, and outlines two general approaches that have the potential to help understand and quantify biological processes that occur in reef communities, at least those found in the NNP Tayrona in the Colombian Caribbean. The first is that, more than its size and coverage, it is the species of coral (frequently in association with the way the colony is organized) that is decisive in the frequency and results of coral-turf interactions. The other is that this implies that in the future it is likely that the composition and complexity of these reefs will also change, in response to escalated levels of disturbance, which will increase the number of competition scenarios between hermatypic corals and algal turfs, and the potential results of these interactions that result from the strategies employed by the corals.
CONCLUSIONS
In the reefs of the PNN Tayrona the most frequent competitor of hermatypic corals are algal turfs, and coral-turf interactions are more frequent in reefs that are close to Santa Marta, and in deeper locations that are protected from wave action. This reflects a residual effect of the perturbation gradient to which these reefs have already been subjected. However, the differences in the frequency of interactions between bays and stations confirms that these perturbations affect each reef in a different way.
The frequency of coral-turf interactions depends on the coral species involved and of its susceptibility to losing coral tissue as a result of the perturbations affecting the reef they inhabit. The result of the competition depends on the coral species involved, in part association with the type of colony organization: meandroid and cerioid colonies being the most successful competitors because of the vertical growth strategies they employ. Plocoid colonies, on the other hand, are more likely to lose. By contrast, environmental factors (gradient, depth and exposure) do not seem to exert a major influence on the frequency and apparent results of these interactions.
Thus, the results of this research predict that the analysis of the historical data on coral cover in the PNN Tayrona should demonstrate greater or lesser degrees of loss in coverage by species from place to place, depending on the distribution of the conditions that are deleterious to coral and favorable to turfs. In addition, the same perturbation tendencies will continue to occur in the future, in the long term favoring, with some exceptions, meandroid and cerioid corals over plocoids.
ACKNOWLEDGEMENTS
This research was made possible by the support and financial backing of Universidad Nacional de Colombia, Sede Caribe and the Instituto de Estudios en Ciencias del Mar - Cecimar, provided by the Convocatoria Nacional para el Apoyo a Proyectos de Investigación y Creación Artística of Universidad Nacional de Colombia 2017-2018 (code: Hermes 39161). The authors also wish to express their gratitude to the Unidad Administrativa Especial del Sistema de Parques Nacionales Naturales - Parque Nacional Natural Tayrona (UAESPNN - PNN Tayrona) for including this research project in its portfolio, and to the Instituto de Investigaciones Marinas y Costeras - Invemar for giving us permission to participate in the field visits organized by the SIMAC groups during its October 2016 campaign, and for covering the diving costs. The results form a part of the MCGC thesis presented achieve the title of Master in Biological Sciences - Marine Biology [Magister en Ciencias-Biología, Línea Biología Marina] awarded by Universidad Nacional de Colombia, Sede Caribe. Cecimar Contribution N° 493.
REFERENCES
Acero-P., A., D. Bustos-Montes, P Pabón-Quintero, C. J. Polo-Silva and A. Sanjuan-Muñoz, A. 2019. Feeding habits of Pterois volitans: A real threat to Caribbean coral reef biodiversity. 269-314. In: Makowski C. y Finkl, C. (Eds.). Impacts of Invasive Species on Coastal Environments, Coastal Research Library. Vol 29. Springer, Cham. [ Links ]
Acosta, A., D, González, E. Navas y L. Sánchez. 2018. Estado de las formaciones coralinas del Parque Nacional Natural Tayrona años 2008 - 2018. Inf. Técn. Mon. Ecosist. ITF # 1. Invemar. Santa Marta. 94 p. [ Links ]
Agresti, A. 2002. Categorical data analysis. Wiley-Interscience a John Wiley y Sons, inc., Publication (Eds.). 2 editions. Florida. 721 p. [ Links ]
Airoldi, L. 2003. The effects of sedimentation on rocky coast assemblages. Oceanogr. Mar. Biol. Annu. Rev., 41: 161-236. [ Links ]
Airoldi, L. and M. Virgilio. 1998. Responses of turf-forming algae to spatial variations in the deposition of sediments. Mar. Ecol. Prog. Ser., 165: 271-282. [ Links ]
Airoldi, L ., F. Rindi and F. Cinelli. 1995. Structure, seasonal dynamics and reproductive phenology of a filamentous turf assemblage on a sediment influenced, rocky subtidal shore. Bot. Mar.., 38(3): 227-237. [ Links ]
Alvarado, E. y A. Acosta. 2009. Lesiones naturales y regeneración de tejido en ramets del coral Montastrea annularis (Scleractinia: Faviidae) en un arrecife degradado del Caribe Colombiano. Rev. Biol. Trop., 57(4): 939-954. [ Links ]
Aronson R. B. and W. F. Precht. 2001. White-band disease and the changing face of Caribbean coral reefs. Hydrobiologia, 460: 25-38. [ Links ]
Barnes, D. J. 1973. Growth in colonial scleractinians. Coral Reef Project - Papers in memory of Dr. Thomas F. Goureau, 12 (Eds.). Queensland - Australia. 19 p. [ Links ]
Barott, K. L., J. Smith, E. Dinsdale, M. Hatay, S. A. Sandin and F. Rohwer. 2009. Hyperspectral and physiological analyses of coral-algal interactions. PLoS One, 4(11): e8043. [ Links ]
Barott, K. L ., G. J. Williams, M. J. A. Vermeij, J. Harris, J. E. Smith, F. L. Rohwer and S. A. Sandin . 2012. Natural history of coral-algae competition across a gradient of human activity in the Line Islands. Mar. Ecol. Prog. Ser ., 460: 1-12. [ Links ]
Bayraktarov, E. 2013. Effects of seasonality and upwelling on coral reef functioning in Tayrona National Natural Park, Colombian Caribbean. Ph.D. Thesis, University of Bremen, Germany. 247 p. [ Links ]
Bayraktarov, E. and C. Wild. 2014. Spatiotemporal variability of sedimentary organic matter supply and recycling processes in coral reefs of Tayrona National Natural Park, Colombian Caribbean. Biogeosciences, 11: 2977-2990. [ Links ]
Bayraktarov, E ., M. L. Bastidas and C. Wild , 2014a. The physical environment in coral reefs of the Tayrona National Natural Park (Colombian Caribbean) in response to seasonal upwelling. Bol. Invest. Mar. Cost., 43(1): 137-157. [ Links ]
Bayraktarov, E ., V. Pizarro and C. Wild . 2014b. Spatial and temporal variability of water quality in the coral reefs of Tayrona National Natural Park, Colombian Caribbean. Environ. Monit. Asses., 186(6): 3641-3659. [ Links ]
Bender-Champ, D., G. Díaz-Pulido and S. Dove. 2014. Warming and acidification promote cyanobacterial dominance in turf algal assemblages. Mar. Ecol. Prog. Ser ., 517: 271-284. [ Links ]
Birrell, C. L., L. J. McCook and B. L. Willis. 2005. Effects of algal turfs and sediment on coral settlement. Mar. Pollut. Bull., 51: 408-414. [ Links ]
Birrell, C. L ., L. J. McCook , B. L. Willis and G. Díaz-Pulido . 2008. Effects of benthic algae on the replenishment of corals and the implications for the resilience of coral reefs. Oceanogr. Mar. Biol. Annu. Rev ., 46: 25-63. [ Links ]
Bruggemann, J. H., M. W. Kuyper and A. M. Breeman. 1994. Comparative analysis of foraging and habitat use by the sympatric Caribbean parrotfish Scarus vettula and Sparisoma viride (Scaridae). Mar. Ecol. Prog. Ser ., 112: 51-66. [ Links ]
Bula-Meyer, G. 1990. Altas temperaturas estacionales del agua como condición disturbadora de las macroalgas del Parque Nacional Tairona, Caribe colombiano: Una hipótesis. An. Inst. Invest. Mar. Punta Betín, 19-20: 9-21. [ Links ]
Burkepile, D. E. and M. E. Hay. 2010. Impact of herbivore identity on algal succession and coral growth on a Caribbean reef. PLoS One, 5(1): e8963. [ Links ]
Bythell, J. C., E. H. Gladfelter and M. Bythell, 1993. Chronic and catastrophic natural mortality of three common Caribbean reef corals. Coral Reefs, 12: 143-152. [ Links ]
CARICOMP. 2001. Methods manual levels 1 and 2: Manual of Methods for mapping and monitoring of physical and biological parameters in the coastal zone of the Caribbean. CARICOMP Data Management Center, Univ. West Indies (Eds.). Kingston. 93 p. [ Links ]
Chornesky, E. A. 1983. Induced development of sweeper tentacles on the reef coral Agaricia agaricites: a response to direct competition. Biol. Bull., 165(3): 569-581. [ Links ]
Colwell, R. K. 2013. EstimateS version 9.1 Statistical estimation of species richness and shared species from samples (software and user's guide). Freeware for Windows and Mac OS, http://viceroy.eeb.uconn.edu/estimates. [ Links ]
Connell, J. H. 1997. Disturbance and recovery of coral assemblages. Coral Reefs , 16(1): 101-113. [ Links ]
Connell, S. D., M. S. Foster and L. Airoldi. 2014. What are algal turfs? Towards a better description of turfs. Mar. Ecol. Prog. Ser., 495: 299-307. [ Links ]
Corado-Nava, N. A., D. Rodríguez, D. y G. Rivas. 2014. Efecto de la colonización de los céspedes algales en el crecimiento de Pocillopora capitata (Anthozoa: Scleractinia) en el Pacífico tropical mexicano. Rev. Mex. Biodivers., 85: 1086-1092. [ Links ]
Cyronaka, T. and B. D. Eyrea. 2016. The synergistic effects of ocean acidification and organic metabolism on calcium carbonate (CaCO3) dissolution in coral reef sediments. Mar Chem,: doi: 10.1016/j.marchem.2016.05.001. [ Links ]
De la Fuente, S. 2011. Regresión logística. Facultad de Ciencias Económicas y Empresariales, Universidad Autónoma de Madrid (Eds.). Madrid. 29 p. [ Links ]
Díaz, J. M., L. M. Barrios, M. H. Cendales, J. Garzón-Ferreira, J. Geister, M. López-Victoria, G. H. Ospina, F. Parra-Velandia, J. Pinzón, B. Vargas-Ángel, F. A. Zapata y S. Zea. 2000. Áreas coralinas de Colombia. Invemar. Ser. Publ. Esp. 5 Santa Marta. 179 p. [ Links ]
Díaz-Pulido, G. and J. Garzón-Ferreira . 2002. Seasonality in algal assemblages on up welling-influenced coral reefs in the Colombian Caribbean. Bot. Mar., 45: 284-292. [ Links ]
Díaz-Pulido, G . and L. J. McCook . 2002. The fate of bleached corals: patterns and dynamics of algal recruitment. Mar. Ecol. Prog. Ser ., 232: 115-128. [ Links ]
Franco-Herrera, A. 2005. Oceanografía de la ensenada de Gaira: El Rodadero, más que un centro turístico en el Caribe colombiano. Fund. Univ. Bogotá Jorge Tadeo Lozano, Bogotá. 56 p. [ Links ]
Fricke, A., M. Teichberg, S. Beilfuss and K. Bischof. 2011. Succession patterns in algal turf vegetation on a Caribbean coral reef. Bot. Mar., 54(2): 111-126. [ Links ]
Friedlander, A., R. Navas, M. Prada, A. Rodríguez-Ramírez and S. Zea . 2014. Part II. Reports for individual countries and territories - Colombia. 196 -201. In: Jackson J. B. C., M. K. Donovan, K. L. Kramer y V. V. Lam (Eds.). 2014. Status and trends of Caribbean coral reefs: 1970 - 2012. Global Coral Reef Monitoring Network, IUCN, Gland, Switzerland. Washington. 306 p. [ Links ]
Garzón-Ferreira, J. and A. Rodríguez-Ramírez. 2010. SIMAC: development and implementation of a coral reef monitoring network in Colombia. Rev. Biol. Trop ., 58(1): 667-680. [ Links ]
Garzón-Ferreira, J . and S. Zea . 1992. A mass mortality of Gorgonia ventalina (Cnidaria: Gorgoniidae) in the Santa Marta area, Caribbean coast of Colombia. Bull. Mar. Sci., 50 (3): 522-526. [ Links ]
Garzón-Ferreira, J ., M. C. Reyes-Nivia y A. Rodríguez-Ramírez , A. 2002. Manual de métodos del SIMAC - Sistema Nacional de Monitoreo de Arrecifes Coralinos en Colombia. Inf. Final Invemar. Santa Marta. 61 p. [ Links ]
Gladfelter W. B. 1982. White-band disease in Acroporapalmata: implications for the structure and growth of shallow reefs. Bull Mar Sci., 32:639-643. [ Links ]
Gómez Cubillos, C. 2018. Interacciones coral-césped de algas y sus efectos en la comunidad coralina en el área de Santa Marta, Caribe colombiano. Tesis M.Sc., Ciencias-Biología, Línea Biología Marina, Universidad Nacional de Colombia, Sede Caribe. [ Links ]
Gómez-Lemos L.A and G. Díaz-Pulido . 2017. Crustose coralline algae and associated microbial biofilms deter seaweed settlement on coral reefs. Coral Reefs , 36(2): 453-462. [ Links ]
Gorgula, S. K. and S. D. Connell. 2004. Expansive covers of turf-forming algae on human-dominated coast: The relative effects of increasing nutrient and sediment loads. Mar. Biol., 145(3): 613-619. [ Links ]
Gowan, J. C., J. S. Tootell and R. C. Carpenter. 2014. The effects of water flow and sedimentation on interactions between massive Porites and algal turf. Coral Reefs , 33(3): 651-663. [ Links ]
Guisande, C., A. Vaamonde y A. Berreiro. 2013. Tratamiento de datos con R, Statistica y SPSS. Ediciones Díaz de Santos (Eds.). 997 p. [ Links ]
Haas, A., M. el-Zibdah and C. Wild . 2010. Seasonal monitoring of coral-algae interactions in fringing reefs of the Gulf of Aqaba, Northern Red Sea. Coral Reefs , 29: 93-103. [ Links ]
Hamilton, S. L., J. E. Smith , N. N. Price and S. A. Sandin . 2014. Quantifying patterns of fish herbivory on Palmyra Atoll (USA), an uninhabited predator-dominated central Pacific coral reef. Mar. Ecol. Prog. Ser ., 501: 141-155. [ Links ]
Harris, J. L. 2015. The ecology of turf algae on coral reefs. Ph.D. Thesis, University of California. 201 p. [ Links ]
Harris, J. L., L. S. Lewis and J. E. Smith . 2015. Quantifying scales of spatial variability in algal turf assemblages on coral reefs. Mar. Ecol. Prog. Ser ., 532: 41-57. [ Links ]
Heyward, A. J. and A. P. Negri. 1999. Natural inducers for coral larval metamorphosis. Coral Reefs , 18(3): 273-279. [ Links ]
Hughes, T. P. 1994. Catastrophes, phase shifts, and large-scale degradation of a Caribbean coral reef. Science, 265(5178): 1547-1551. [ Links ]
Hughes, T. P. and J. H. Connell. 1999. Multiple stressors on coral reefs: A long-term perspective. Limnol. Oceanogr., 44(3-2): 932-940. [ Links ]
Hughes, T. P ., M. J. Rodríguez, D., R. Bellwood, D. Ceccarelli, O. Hoegh-Guldberg, L. McCook, N. Moltschaniwskyj, M. S. Pratchett, R. S. Steneck and B. Willis. 2007. Phase shifts, herbivory and the resilience of coral reefs to climate change. Curr. Biol., 17: 360-365. [ Links ]
Johnson, M. D., S. Comeau, C. A. Lantz and J. E. Smith . 2017. Complex and interactive effects of ocean acidification and temperature on epilithic and endolithic coral-reef turf algal assemblages. Coral Reefs 36(4): 1-12. [ Links ]
Jompa, J. and L. J. McCook . 2002. The effect of herbivory on competition between a macroalga and a hard coral. J. Exp. Mar. Biol. Ecol., 271: 25-39. [ Links ]
Jompa J. and L. J. McCook . 2003a. Contrasting effects of turf algae on corals: massive Porites spp. are unaffected by mixed-species turfs, but killed by the red alga Anotrichium tenue. Mar. Ecol. Prog. Ser ., 258: 79-86. [ Links ]
Jompa J . and L. J. McCook . 2003b. Coral-algal competition: macroalgae with different properties have different effects on corals. Mar. Ecol. Prog. Ser ., 258: 87-95. [ Links ]
Karlson, R. H. 1980. Alternative competitive strategies in a periodically disturbed habitat. Bull. Mar. Sci ., 30(4): 894-900. [ Links ]
Kennelly, S. J. 1987. Inhibition of kelp recruitment by turfing algae and consequences for an Australian kelp community. J. Exp. Mar. Biol. Ecol ., 112: 49-60. [ Links ]
Lang, J. C . and E. A. Chornesky. 1990. Competition between scleractinian reef corals: a review of mechanisms and effects. 209-252. In: Dubinsky, Z. (Ed.) Ecosystems of the world, 25. Coral reefs. Amsterdam. 521 p. [ Links ]
Ledlie, M. H., N. A. J. Graham, J. C. Bythell, S. K. Wilson, S. Jennings, N. V. C. Polunin and J. Hardcastle. 2007. Phase shifts and the role of herbivory in the resilience of coral reefs. Coral Reefs , 26: 641-653. [ Links ]
Lessios, H. A., D. R. Robertson and J. D. Cubit. 1984. Spread of Diadema mass mortality through the Caribbean. Science, 226: 335-337. [ Links ]
Littler, M. M., D. S. Littler and B. L. Brooks. 2006. Harmful algae on tropical coral reefs: bottom-up eutrophication and top-down herbivory. Harmful Algae., 5:565-585. [ Links ]
López-Victoria, M., S. Zea and E. Weil. 2006. Competition for space between encrusting excavating Caribbean sponges and other coral reef organisms. Mar. Ecol. Prog. Ser ., 312: 113-121. [ Links ]
Loya. Y., K. Sakai, K. Yamazato, Y. Nakano, H. Sambali and R. van Woesik. 2001. Coral bleaching: The winners and losers. Ecol. Lett., 4: 122-131. [ Links ]
Maguire, L. and J. Porter. 1977. A spatial model of growth and competition strategies in coral communities. Ecol. Modell., 3: 249-271. [ Links ]
Márquez, J. C. and S. Zea . 2012. Parrotfish mediation in coral mortality and bioerosion by the encrusting, excavating sponge Cliona tenuis. Mar. Ecol., 33: 417-426. [ Links ]
Martínez, S. y A. Acosta . 2005. Cambio temporal en la estructura de la comunidad coralina del área de Santa Marta - Parque Nacional Natural Tayrona (Caribe colombiano). Bol. Invest. Mar. Cost ., 34: 161-191. [ Links ]
McCook, L. J. 2001. Competition between corals and algal turfs along a gradient of terrestrial influence in the nearshore central Great Barrier Reef. Coral Reefs , 19: 419-425. [ Links ]
McCook, L. J., J. P. Jompa and G. Díaz-Pulido . 2001. Competition between corals and algae on coral reefs: a review of evidence and mechanisms. Coral Reefs , 19: 400-417. [ Links ]
McManus, J. W. and J. F. Polsenberg. 2004. Coral-algal phase shifts on coral reefs: ecological and environmental aspects. Prog. Oceanogr., 60: 263-279. [ Links ]
Meesters, E. H., A. Bos and G. J. Gast. 1992. Effects of sedimentation and lesion position on coral tissue regeneration. 671-678. In: Proceedings of the Seventh International Coral Reef Symposium (Eds.). Guam-Micronesia. 640 p. [ Links ]
Meesters, E. H ., I. Wesseling and R. P. M. Bak. 1996. Partial mortality in three species of reef-building corals and the relation with colony morphology. Bull. Mar. Sci ., 58(3): 838-852. [ Links ]
Navas-Camacho, R., D. L. Gil-Agudelo, A. Rodríguez-Ramírez , M. C. Reyes-Nivia and J. Garzón-Ferreira . 2010. Coral diseases and bleaching on Colombian Caribbean coral reefs. Rev. Biol. Trop ., 58 (Suppl. 1): 95-106. [ Links ]
Nugues, M. M., L. Delvoye, P. Rolf and M. Bak. 2004. Coral defense against macroalgae: differential effects of mesenterial filaments on the green alga Halimeda opuntia. Mar. Ecol. Prog. Ser ., 278: 103-114. [ Links ]
Ogilvie, M. M. 1896. Microscopic and systematic study of madreporian types of corals. Philosophical Transact. Roy. Soc. London, series B, 187, 270 p. [ Links ]
Ott, B. and J. B. Lewis. 1972. The importance of the gastropod Coralliophila abbreviata (Lamarck) and the polychaete Hermodice carunculata (Pallas) as coral reef predators. Can. J. Zool., 50: 1651-1656. [ Links ]
Preskitt, L. B., P. S. Vroom and C. M. Smith. 2004. A rapid ecological assessment (REA) quantitative survey method for benthic algae using photoquadrats with scuba. Pac. Sci., 58(2): 201-209. [ Links ]
Ramírez, G. 1983. Características físico-químicas de la bahía de Santa Marta (agosto 1980 - Julio 1981). An. Inst. Invest. Mar. Punta Betín ., 13: 111-121. [ Links ]
Reyes, J., N. Santodomingo y P. Flórez. 2010. Corales escleractíneos de Colombia. Invemar, Serie de Publicaciones Especiales, No. 14. Santa Marta. 246 p. [ Links ]
Richardson, C. A., P. Dustan y J. C. Lang. 1979. Maintenance of living space by sweeper tentacles of Montastraea cavernosa, a Caribbean reef coral. Mar. Biol., 55: 181-186. [ Links ]
Rogers, C. S., T. H. Suchanek y F. A. Pecora. 1982. Effects of hurricanes David and Frederic (1979) on shallow Acropora palmata reef communities: St. Croix, U.S. Virgin Islands. Bull. Mar. Sci ., 32(2): 532 - 548. [ Links ]
Roy, R. E. 2004. Turf algal/sediment (TAS) Mats: A chronic stressor on scleractinian corals. Ph.D. Thesis, University of Texas at Austin. 191 p. [ Links ]
Sandin, S. A., J. E. Smith , E. E. Demartini, E. A. Dinsdale, S. D. Donner, A. M. Friedlander, T. Konotchick, M. Malay, J. E. Maragos, D. Obura, O. Pantos, G. Paulay, M. Richie, F. Rohwer, R. E. Schroeder, S. Walsh, J. B. C. Jackson, N. Knowlton and E. Sala. 2008. Baselines and degradation of coral reefs in the Northern Line Islands. PLoS One ., 3 ( 2): e1548. [ Links ]
Schoener, T. W. 1983. Field experiments on interspecific competition. Amer. Nat., 122(2): 240-285. [ Links ]
Sokal, R. R. and F. J. Rohlf. 1981. Biometry. The principles and practice of statistics in biological research. 2nd edition. W. H. Fremen. San Francisco. 867 p. [ Links ]
Sousa, W. P. 1979. Experimental investigations of disturbance and ecological succession in a rocky intertidal algal community. Ecol. Monogr., 49(3): 227-254. [ Links ]
Steneck, R. S. 1997. Crustose corallines, other algal functional groups, herbivores and sediments: complex interactions along reef productivity gradients. 695-700. In: Lessios, H.A. and I. G. Macintyre (eds.) Proc. 8th Intern. Coral Reef Symp. Vol. 1. Smithsonian Tropical Research Institute, Panama. 2119 p. [ Links ]
Swierts, T. and M. J. A. Vermeij. 2016. Competitive interactions between corals and turf algae depend on coral colony form. PeerJ., 4: e1984 https://doi.org/10.7717/peerj.1984. [ Links ]
Titlyanov, E. A., I. M. Yakovleva and T. V. Titlyanova. 2007. Interaction between benthic algae (Lyngbya bouillonii, Dictyota dichotoma) and scleractinian coral Porites lutea in direct contact. J. Exp. Mar. Biol. Ecol ., 342: 282 - 291. [ Links ]
Underwood, A. 1997. Experiments in ecology: their logical design and interpretation using analysis of variance. Cambridge University Press. New York. 504 p. [ Links ]
Vega-Sequeda, J., A. Rodríguez-Ramírez y M. C. Reyes-Nivia. 2008. Formaciones coralinas del área de Santa Marta: estado y patrones de distribución espacial de la comunidad bentónica. Bol. Invest. Mar. Cost ., 37(2): 87-105. [ Links ]
Vermeij, M. J. A., M. L. Dailer, S. M. Walsh, M. K. Donovan and C. M. Smith . 2010. The effects of trophic interactions and spatial competition on algal community composition on Hawaiian coral reefs. Mar. Ecol., 31: 291-299. [ Links ]
Werding, B. y H. Sánchez. 1988. Deterioro observado en las formaciones coralinas de la Bahía de Santa Marta, Colombia. An. Inst. Invest. Mar. Punta Betín , 18: 9-16. [ Links ]
Werding, B . and H. Sánchez . 1989. The coral formations and their distributional pattern along a wave exposure gradient in the area of Santa Marta, Colombia. Medio Amb., 10(2) 61-68. [ Links ]
Zea, S. 1993. Cover of sponge and other sessile organism in rocky and coral reef habitats of Santa Marta, Colombian Caribbean Sea. Carib. J. Sci., 29(1-2): 75-88. [ Links ]
Zea, S. 1994. Patterns of coral and sponge abundance in stressed coral reefs at Santa Marta, Colombian Caribbean. 257-264. In: Van Soest, R., Van Kempen, T. and J. C. Bracekman (eds.), Proc. 4th Intern. Porifera Congress. Amsterdam. 544. [ Links ]
Received: November 16, 2018; Accepted: September 02, 2019