INTRODUCTION
The Gulf of Guayaquil constitutes the most singular geomorphological feature of the entire profile of the Ecuadorian coast. It is an oceanic and a coastal region divided into two large estuarine systems with physiographic, geological and oceanographic properties, characteristics represented by its high productivity, low diversity and pronounced environmental stress, dominated by unpredictable fluctuations in salinity (Comisión Asesora Ambiental [CAAM], 1995).
Estuaries play a prominent role in the life cycle of many marine populations (Ketchum, 1992). They are identified as important breeding sites, given the appropriate availability of areas for shelter and/or feeding, which generates favorable conditions for the settlement of larvae and juveniles of different aquatic organisms of economic value (Barletta et al., 1998, 2003; Able et al., 1999) such as crustaceans, mollusks, and fish. The latter is considered one of the most important groups since they play significant ecosystem roles such as the transformation of the debris energy potential, energy transfer between different levels of the trophic networks and energy balance between neighboring ecosystems (Yánez-Arancibia and Nugent, 1977; Beck et al., 2001).
Estuarine fish communities represent a combination of freshwater and marine species, living on the edge of their distribution, estuarine residents, and migratory species that traverse the estuary for reproductive or trophic purposes (Maes et al., 1998). The distribution of their planktonic stages is determined jointly by various physicochemical factors, tidal cycle and currents, since the larvae when suspended in the water column are to a greater degree related to the movements of the tidal cycles. Likewise, they are subject to the annual maturation cycles of adults together with their reproductive strategies, since more than 80 % of the coastal fish use the estuary in one of its stages of maturity and/or development (Yáñez-Arancibia and Nugent, 1977). The vast majority enters the estuary as larvae, from adjacent marine environments; other fish spawn within the estuary to complete their life cycle and leave it as juveniles (Whitfield, 1999). Therefore, the composition of the ichthyoplankton in an estuary fluctuates over time, with little relation to the composition of adults when it comes to non-resident species. Given this, knowledge of the temporal and spatial distribution of ichthyoplankton is essential, not only for understanding adult population fluctuations and their potential spawning areas but also for understanding the general functioning of the estuarine ecosystem (Moser and Smith, 1993a; Cowan and Shaw, 2002; Ottersen et al., 2010) and the recurrence of species in this system.
In Ecuador, various taxonomic investigations of marine fish larvae have been carried out, establishing their distribution and abundance. The works by Cajas and Hinostroza (1981), Peribonio et al. (1981), García (1983), Ortega et al. (1996), Luzuriaga de Cruz et al. (1998), Torres et al. (2003-2004) and Calderón et al. (2018) emphasize the Gulf of Guayaquil as a productive area, being particular to the south of the Puná Island where the increased dominance of fish eggs and larvae primarily of commercial interest, and that it extends to the north of the gulf towards the coast and to the south of the ocean, where according to Jiménez and Bonilla (1980) there is a relationship between the distribution pattern of phytoplankton with that of ichthyoplankton. Recently, Salcedo and Coello (2018), in their work on the dynamics of plankton against the province of El Oro, report the dominance of fish larvae of the family Engraulidae with two periods of abundance, from June to August and from October to December, a result of the concentrations of Anchovia macrolepidota, the characteristic species of this ecosystem. This research work determined the density and distribution patterns (spatial and temporal) of fish larvae in the adjacent interior and exterior estuary of the Gulf of Guayaquil, evaluating their relationship with local conditions of temperature, salinity, and transparency, during June to December 2012, months that characterize the dry and transitional season in Ecuador.
MATERIALS AND METHODS
Study area
The Guayas province is located in the southwest of Ecuador and is influenced by several marine currents, especially the cold of Humboldt during the dry season, from July to November, and the warm El Niño in the rainy season, from January to May; while June and December are considered transitional months (Twilley et al., 2001; Prado et al., 2015), which produce a tropical savanna and tropical monsoon type climate with average temperatures of 25 °C and annual rainfall between 500 to 1000 mm (Twilley et al., 2001). In the coastal margin of this province, the Gulf of Guayaquil (3° S. 80° W), the Southeast Pacific main estuary, is located, which is divided into two specific areas: a so-called exterior estuary, an open oceanic region influenced by the masses of tropical Pacific waters, which begins at the height of the point of Santa Elena and has as its limit at the platform along the meridian 81° W to the western side of the Puná island (80° 15' W), with a predominance of coastal cords and high and unstable cliffs (Ayón, 1987). On the other hand, the interior estuary, known as the Guayas River estuary, is made up to the northwest by the Salado estuary and to the southeast by the Churute estuary, which receive the contributions of the Taura and Churute rivers (Cucalón, 1996), governed mainly by the tides, the river discharge and the effort of the wind, with an abundance of mangroves that give it the appearance of a large swamp (CAAM, 1995). In general terms, the depth of the Gulf of Guayaquil on the continental shelf ranges from 180 m at the western edge to less than 20 m in the interior (Montaño-Armijos and Sanfeliu-Montolio, 2008).
Sampling
The material comes from 112 samples of zooplankton collected in 16 sampling stations, located 1 mn (1.8 km) away from the coastal margin in front of the province of Guayas and northwest of the island of Puná, during the dry season period (July to November) and transition (June and December) 2012. The stations were selected for their importance as areas of fish reproductive activity and categorized according to their location: the interior zone or inland estuary, represented by eight (8.9, 10,11,12,13,14 and 15) shallow and close stations influenced by estuaries and rivers, and the exterior zone or exterior estuary by eight (1,2,3,4,5,6,7 and 16) stations with greater marine influence (Figure 1).
Vertical records of temperature and salinity were made in each station with a Sea-Bird brand CTD profiler, Seacat S19V2 model, and the transparency of the water was determined with a Secchi (m) disk. The zooplankton samples were collected at high tide using surface trawls (0-1 m deep), daytime (between 08:00 and 11:00 am), circular, with a duration of 5 min and at a speed of 3.7 km/h on board a boat with an outboard motor. A simple conical network 30 cm in diameter, 1.2 m in length, and 300 μm of mesh opening was used. At the mouth of the network, a General Electric® flowmeter was attached to quantify the volume of filtered water and standardize the abundance per unit volume. The zooplankton samples were fixed in a formalin solution with seawater at a final concentration of 4 % and transported to the Ichthyoplankton Laboratory of the National Fisheries Institute (Guayaquil, Ecuador).
Laboratory analysis
All of the samples were reviewed. The number of individuals/100 m3 of sea surface was separated, counted, and standardized, using the method described by Smith and Richardson (1979). Larvae were identified down to the lowest possible taxonomic level, following the criteria established in Moser (1996), Beltrán-León and Ríos (20002001), Richards (2005) and Calderón (2011).
Analysis of data
Differences in hydrographic variability and abundance of fish larvae were evaluated, considering the study areas (internal and external) as a factor of variability in space, and using the Mann Whitney parametric test (MW-U) (Sheskin, 2004) through the Statgraphics Plus 5.1 program.
With the quantitative data, the relative abundance of the families and species of fish larvae by months and study areas was calculated. The estimation of the specific richness (S), diversity (H') and equity (J') of the larval community was described utilizing the community attribute indices of Shannon-Weaver (Shanon and Weaver, 1963) and Pielou using the base logarithm 2 (Tsirstsis and Karydis, 1998; Krebs, 1999) with a bit/Ind unit and developed with the open-access statistical software Past 3.0. Also, the "inverse to distance" interpolation method was used with the QGIS® 3.0 computer program to represent the spatial variation of the abundance of fish larvae and the oceanographic conditions in the area for the study period.
To establish possible differences in the community structure of fish larvae between zones, nonparametric multidimensional analysis (MDS-Anosim) was used and to determine the contribution of the species between zones, the SIMPER percentage of similarity was obtained. All multivariate analyzes were performed using the Past 3.0 software (Hammer et al., 2001). Finally, the degree of relationship between the established structure of the fish larvae assembly and the physical-chemical conditions for each study area was evaluated, using redundancy analysis (RDA) developed with the free access statistical software PcOrd 6.0 (McCune and Grace, 2002). This multivariate association test maximizes the degree of correlation between the abundance of fish larvae and the physical-chemical parameters.
RESULTS
Environmental conditions
The variability of the sea surface temperature (SST) allowed identifying significant differences (MW-U: 2632; p < 0.05) between the study areas (Figure 2; Table 1) and the highest average records in the interior estuary (25.7 °C), mainly in the stations near the Morro canal (station nine, 10 and 11) (Figure 3a). The temporal variation of the SST showed an average of 25.2 °C (± 0.71) with the minimum value (24.3 °C) in August and the maximum (26.5 °C) in June. Overall, the months of the transition period (June and December) recorded the highest values of sea surface temperature (26.1-25.5 °C) (Figure 2a and Figure 2b).
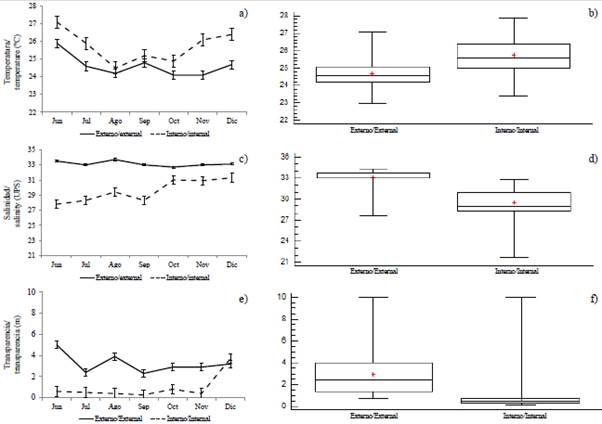
Figure 2 Monthly variation and by zones: a and b) temperature, c and d) salinity, e and f) transparency, in the exterior and interior estuary of the Gulf of Guayaquil against the province of Guayas between June and December 2012. The horizontal line corresponds to the median and the point to the average.
Salinity presented significant differences (Figure 2; Table 1) between sampling areas (MW-U: 89; p < 0.05), with the lowest records in the interior estuary, mainly in stations eight and ten. In the exterior estuary, the highest salinity values were recorded in stations two and three, located near the coast of Santa Elena and with the greatest marine contribution (Figure 3b). The average salinity from June to December was 31.4 (± 0.7) with ranges from 30.7 to 32.2 ups, with the minimum in June, July, and August, and the maximum in November (Figure 2c and Figure 2d).
Table 1 Physical-chemical parameters recorded in the exterior and interior estuary of the Gulf of Guayaquil against the province of Guayas between June and December 2012. |i: average, D.s.: standard deviation, Min: minimum recorded value. Max: maximum registered value. W: Wilcoxon value, p: probability value.

The transparency of the water showed an average of 2.0 m (± 2.4), with significant differences (Figure 2, Table 1) between zones (MW-U: 120; p < 0.05), with the lowest records in the interior estuary, mainly in the stations located at the mouth of the Morro channel (8, 9, 10) and the Cascajal channel (11 and 12) (Figure 3c). In the months studied, transparency showed the lowest record (1.3 m) in July and the highest (2.6 m) in June (Figure 2g and Figure 2h). In general, the external zone was observed with a lower temperature, but with greater salinity and transparency to the internal zone of the Gulf of Guayaquil (Figure 3, Table 1).
Community structure of fish larvae
A total of 321,638 larvae/100 m3 were quantified, with significant differences between the sampling areas (MW-U: 450; p < 0.05) (Figure 4). The spatial distribution showed higher average larval densities towards the exterior estuary (8,458 larvae/100 m3, ± 25,215), with maximums (122,648 larvae/100 m3) in station seven and minimums (16,376 larvae/100 m3) in station five. The interior estuary, with an average of 2,272 larvae/100 m3 (± 4,967), registered the highest density in station eight, located at the mouth of the Canal del Morro, with 8,676 larvae/100 m3 and lower density in station nine with 906 larvae/100 m3 (Figure 4).
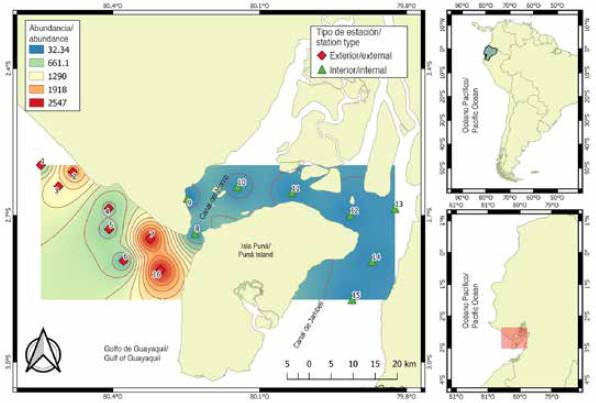
Figure 4 Spatial variability of the abundance of fish larvae in the exterior and interior estuary of the Gulf of Guayaquil against the province of Guayas.
Larval abundance showed temporal variations, with significant differences between months (KW = 14.24; p < 0.05). The highest larval densities corresponded to December (121,220 larvae/100 m3) and July (107,631 larvae/100 m3), while the lowest was recorded in November (6,690 larvae/100m3). The general pattern exhibited greater abundances towards the exterior estuary of the Gulf of Guayaquil and framed towards the beginning of the dry season (July) and the transitional season (December) (Figure 5a and Figure 5b). The larval species that characterized the external and internal zone were Anchoa sp. and Bairdiella sp. with high values during December, while Gobiosoma sp. and Haemulon sp. registered maximum densities during July, and Anchovia macrolepidota in August (Table 2).
Table 2 Taxonomic list of fish larvae found in the exterior (E) and interior (I) estuary of the Gulf of Guayaquil, from July to December 2012. The relative abundance (Ar. %) and months of the occurrence of the larvae collected from the two study areas.
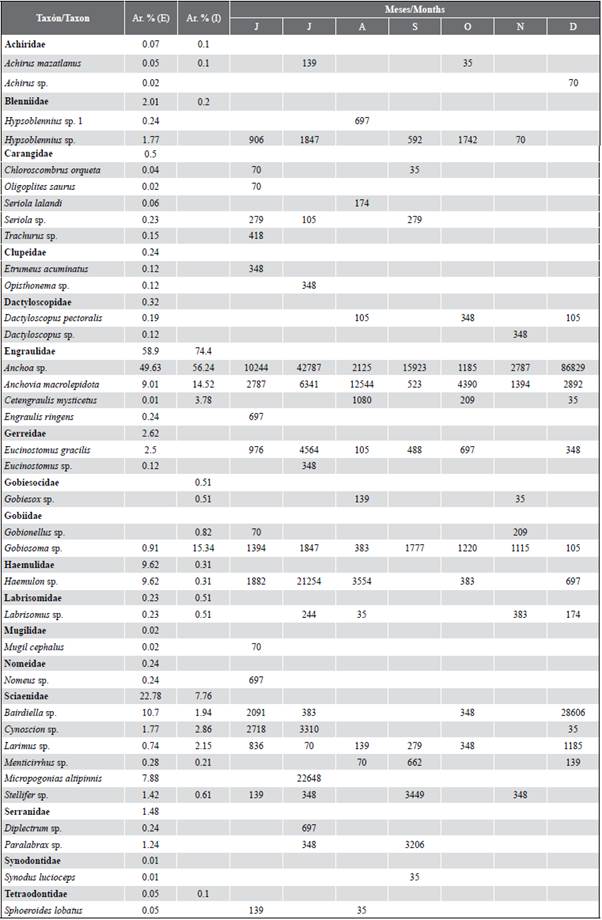
A total of 17 families and 36 species were identified, of which 34 concurred in the stations of the exterior estuary and 16 in the interior estuary (Table 2). A total of three families (Engraulidae, Gobiidae, and Sciaenidae) were consistently present during all the months of the study. The samples in the external zone were dominated by larvae of the families Engraulidae (58.9 %), Sciaenidae (22.8 %) and Haemulidae (9.6 %), represented by the species Anchoa sp. (49.6 %), Bairdiella sp. (10.7 %) and Haemulon sp. (9.6 %), respectively. While in the interior estuary, they were Engraulidae (74.4 %) and Gobiidae (16.1 %), the most abundant groups and represented in this sense by Anchoa sp. (56.2 %), Anchovia macrolepidota (14.5 %) and Gobiosoma sp. (15.3 %). In general, the taxa Anchoa sp. (50.3 %). Bairdiella sp. (9.7 %) Anchovia macrolepidota (9.6 %), Haemulon sp. (8.6 %), and Gobiosoma sp. (7.0 %) represented 85 % of the total larval density for the two study areas (Table 2).
The specific richness varied from 9 species in November to 20 in June with a temporal average of 13 species (± 3.89). Significant differences were observed between zones (MW-U: 325; p < 0.05), showing the greatest wealth in the exterior estuary with a similar trend as the recorded larval abundance (Figure 5c and Figure 5d).
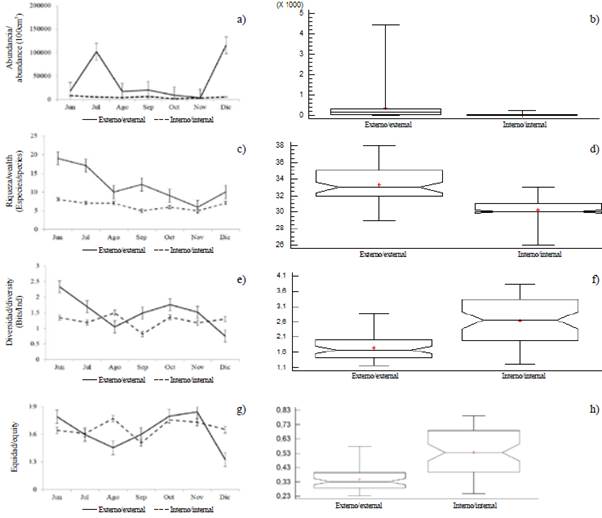
Figure 5 Monthly variation of a and b) abundance, c and d) wealth, e and f) diversity, g and h) equity of ichthyoplankton in the exterior zone and interior estuary of the Gulf of Guayaquil against the province of Guayas between June and December 2012. The horizontal line corresponds to the median and points to average.
Larval diversity (H') varied significantly between study areas (MW-U: 2655; p < 0.05), with minimum values being recorded in the internal estuary during September (0.81 bits/ind) and maximum in August (1.5 bits/ind). In the exterior estuary during June (2.32 bits/ind), the highest diversity was observed and in December (0.74 bits/ind), the lowest (Figure 5e and Figure 5f). In general, the diversity observed in the internal zone had more constant behavior over time compared to those registered in the external zone (Figure 5e and Figure 5f).
The minimum equity (0.31) was observed in December and the maximum in October (0.77). There were significant differences between zones (MW-U: 2702, p < 0.05), showing greater homogeneity in the estuarine zone (Figure 5g and Figure 5h).
When exploring the similarity relationships (MDS Anosim) between the ichthyoplankton communities, significant differences (R: 0.62; p < 0.05) were established between the external and internal zones of the Gulf of Guayaquil. However, it is observed that there are species found in both zones and that showed a greater heterogeneity in the internal stations with the stress of 0.11 (Figure 6). When performing the percentage similarity analysis (SIMPER) to explore aspects of the internal structure of the interior and exterior estuary areas, differences were noted between the two, distinguishing Anchoa spp., Haemulon sp., Anchovia macrolepidota as the species that contributed the most to separating the areas (Table 3).
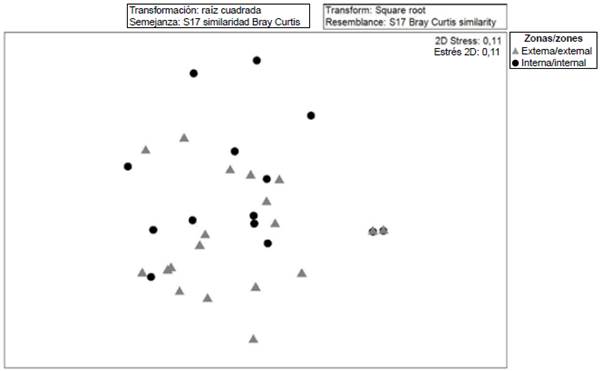
Figure 6 Diagram of multidimensional scaling (MDS) analysis of the community structure of fish larvae in the exterior and interior estuary of the Gulf of Guayaquil against the province of Guayas between June and December 2012.
Relationship between fish larvae and environmental variables
The redundancy analysis (RDA) allowed studying the relationship between the structure of ichthyoplankton and the environmental variables of the interior and exterior estuary. The RDA for the exterior zone explained 14.0 % of the total variance in the first two axes, with a Pearson correlation of r = 0.50, indicating the positive correlation of the species and the environmental variables (Table 4). The first axis explained 12.1 % of the variance and presented an eigenvalue of 0.54. The transparency variable (m) registered a negative correlation, reaching the value of r = -0.63. Axis two only contributed 1.9 % and presented an eigenvalue of 0.50, registering salinity with a negative correlation of r = -0.53 (Table 4). The scatter diagram shows that species such as Etrumeus acuminatus, Achirus mazatlanus, Sphoroiedes lobatus, Oligoplites saurus Diplectrum sp. were associated with increased water transparency. While species such as Gobiosoma sp., Labrisomus sp., Larimus sp., Cynoscion sp., Synodus lucioceps, Eucinostomus gracilis, Seriola lalandi and Stellifer sp. were related to high salinity values (Figure 7a).
Table 4 Redundancy analysis on the spatial relationship of the de ictioplancton species in the exterior and interior estuary of the Gulf of Guayaquil against the province of Guayas between June and December 2012.
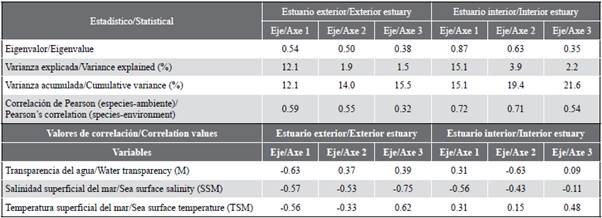
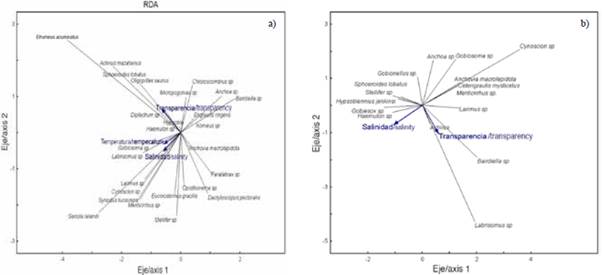
Figure 7 Scatter diagram of fish larvae in the exterior estuary a) and interior estuary b) of the Gulf of Guayaquil in front of the province of Guayas between June and December 2012. Temperature (SST). Salinity (SSM). Transparency (M).
For the internal zone, the RDA explained variance in the first two axes of 19.4 %, with a Pearson's correlation coefficient of r = 0.71. The first axis was the one that contributed the most with 15.5 % of the explained variance and an eigenvalue of 0.87 (Table 4). The salinity variable registered a negative correlation with a value of r = -0.56. Axis two only contributed 3.9 % of the total and presented an eigenvalue of 0.63. The transparency variable registered a negative correlation of r = -0.63 to this axis (Table 4). The scatter diagram shows that Gobiesox sp. and Haemulon sp. were associated with high concentrations of salinity. Taxes such as Bairdiella sp., Labrisomus sp. and Larimus sp. were related to the stations with the highest transparency. In contrast, Anchoa sp., Anchovia macrolepidota, Gobiosoma sp. and Cetengraulis mysticetus were associated with low salinity values (Figure 7b).
DISCUSSION AND CONCLUSIONS
In the present study, the differences in the hydrographic conditions of the Gulf of Guayaquil in the exterior and interior estuary are clear, evidenced by the relationships of temperature, salinity, and transparency. An external zone, where the oceanic influence is evident -with a lower temperature, greater salinity, and transparency- to the internal zone of the gulf, where the depth is reduced by the great sedimentary contribution, the mixing processes increase and the salinity decreases, especially during the dry season, as a result of the tidal flow (semi-day) influenced by the supply of freshwater from the Guayas River (Stevenson, 1981) and, as a whole, regulated by seasonal fluctuations of the dry season, with cold temperatures from July to November, normal oscillation, given the intensification of the cold Humboldt current that advances from the south to the coast of Ecuador, displacing its cold, saline and nutrient-rich waters for these months.
Our results showed characteristics similar to those observed in other studies carried out in the Gulf of Guayaquil or adjacent areas (Cajas and Hinostroza, 1981; Ortega and Elías, 1996; Torres et al., 2003; Elías et al., 2007; Calderón et al., 2018; Salcedo and Coello, 2018), with relatively high larval densities in the external area of the gulf, with a predominance of engulid larvae and low larval diversity. The greatest larval abundance was registered in the exterior part of the gulf, specifically in the stations adjacent to the Morro channel, where the temperature, salinity, and transparency of the water were more stable; therefore, they could have favored the development of fish eggs and larvae (Saborido, 2008). Another element that could contribute to said distribution is the combination of environments in external sites, such as rocky and/or sandy bottoms and the greater marine influence, which allow a greater diversity of adults to group, whether for reproductive purposes, food or refuge (Whitfield, 1999), thus affecting the composition and abundance of ichthyoplankton. For example, species such as Hypsoblennius sp. 1 appeared in these sites, which mainly inhabit rocky bottoms and were only found in external sites. As one enters the inland estuary, it is observed that larval densities decrease as a response of several factors, among them the bottom topography, which affects the tides and turbulence since the depth decreases, the mixing processes and photosynthesis reduces due to the presence of suspended and dissolved particles (Prado and Buchelli, 2012), which results in reduced food and less presence of fish larvae.
One distinguishing characteristic of fish larvae assemblages in tropical estuaries is the dominance of few species, which generally appear associated with a high number of species in low abundance that occasionally frequent the estuary (Whitfield, 1989, 1999; Morais, 1994; Barletta-Bergan et al., 2002; Barletta and Barletta-Bergan, 2009). This condition is observed in the Guayaquil Gulf since it was dominated by larvae of three fish families: engulid, scianid and gobiidae, coinciding with that described by Calderón et al. (2018) and Salcedo and Coello (2018).
Likewise, in heterogeneous systems, there is a high variety of fish species with different requirements and/or spawning patterns, where some species reproduce throughout the year and others exhibit one or two reproductive peaks (Pittman et al., 2004). Based on this, in the gulf, the temporal variability found can reflect the different reproductive patterns that species that inhabit this system as adults present. The engulids, mainly represented by Anchoa sp., stood out with maximum densities in the entire area -both in the internal and external zones- and months of study, which suggests that their presence as a larval stage is not seasonal, and that probably in this study area reproduces several times a year, being able to spawn in the external zone, so that its larvae enter and develop in the interior estuary (Day et al., 1989; Whitfield, 1999). Likewise, its distribution would be explained by being a family-like Gobiidae of brackish-marine habitat (Whitehead et al., 1988; Whitehead and Rodríguez-Sánchez, 1995), which are regularly reported in estuaries or bays (Silva-Segundo et al., 2008). Meanwhile, those larval stages that were only recorded in a sampling area and/or month -such as scianids, demersal, estuary-dependent species, with spawning in coastal areas, estuaries and bays (Ocaña-Luna and Sánchez Ramírez, 2003) and that migrate from the laying area to the mouths of the breeding areas- could correspond to species with a single annual reproductive period in the external area of the gulf.
Diversity varied in space and time. The largest larval diversity was recorded in the interior estuary, observing an inverse relationship between species abundance and richness. This suggests an important exchange and mix of marine and estuary species. In relation, Day et al. (1989) and McLusky and Elliot (2004) pointed out that it is common that in areas with marine influence, the richness of zooplankton organisms is greater, particularly in the mouth, forming a mixed community. On the other hand, other authors point out that internal areas favor larval aggregation by providing shelter and feeding conditions, related to greater turbidity, large mangrove roots and high sedimentation due to the discharge of water from the estuaries (Barletta-Bergan et al., 2002; Barletta and Barletta-Bergan, 2009). However, it is not necessarily a reflection of larval diversity in each zone, since not all larval stages occupy this area permanently since a high number of them are carried by tidal currents (Neira and Potter, 1992; Ramos et al., 2005), since their locomotion is limited in the early stages of life (Jiménez, 2008).
Regarding species richness, it is possible to identify an increase in the stations with the greatest marine influence (exterior estuary), but in the boca del Canal del Moro (station eight and nine) the pulse is maximum, reflecting high traffic of species, and that coincides with the distribution of salinity, a variable that plays an important role in the distribution and species reproductive processes. This not only evidences the specialized biological and ecological strategies that species possess -such as Anchoa sp., which has conditions to move in areas of great environmental variability (Yáñez-Arancibia et al. , 1985)-, but also reveals this site as an important recruitment area for many species, which take advantage of the favorable conditions offered by this system, such as high biological productivity and the refuge of the environment as breeding and laying area.
Although it is known that generally in tropical estuarine areas, the spatio-temporal distribution of adult and juvenile fish is influenced by changes in environmental factors (such as temperature and salinity) (Laroche et al., 1997) and by precipitation and changes in tides. Each estuary presents particular morphological characteristics such as type of substrate, distance to the ocean, etc., factors that also influence the structure of fish communities (Pérez- Ruzafa et al., 2007).
Based on this, utilizing the redundancy analysis, it was observed that for the Gulf of Guayaquil the larval relationships occurred mainly around the turbidity and salinity of the water. In general, larval and juvenile stages are considered to be more tolerant of environmental variability for many marine species than adults (Holliday, 1971). Larvae species such as Gobiosoma sp., Labrisomus sp., Larimus sp., Cynoscion sp., Synodus lucioceps, Haemulon sp., Eucinostomus gracilis, Seriola lalandi, Stellifer sp., Gobiesox sp., Anchoa sp., Anchovia macrolepidota, and Cetengraulis mysticetus, which are considered mostly estuarine species, were associated with high and low salinity. This suggests that these species show greater tolerance to salinity ranges, which is why many of them were present both in the estuarine environment and under the marine influence whereas species such as Etrumeus acuminatus, Achirus mazatlanus, Sphoeroiedes lobatus, Oligoplites saurus, Bairdiella sp., Diplectrum sp., Engraulis ringens, and Opisthonema sp., which mostly belong to the marine component such as Etrumeus acuminatus, Engraulis ringens and Opisthonema sp., remained in one zone or area. Specifically, in the external zone they were positively associated with the highest transparency, reflecting the preference of these species for specific physicochemical characteristics.
Therefore, it is possible to hypothesize that in an estuarine environment, the temporal variation of environmental conditions would be modulating the distribution, abundance, and, in general, the community of fish larvae. Likewise, these seasonal and temporal fluctuations represent the reproductive strategies of each species linked to their habitat (Sánchez, 1997), assuming the availability of food for their survival (Whitehead et al. , 1988), since frequently the abundance of fish larvae is closely related to the biomass of zooplankton (Kaunda-Arara et al., 2009).
According to the results obtained, the Gulf of Guayaquil, at least during the sampled period, presents an assembly of fish larvae with a taxonomic composition composed mainly of the presence of estuarine species with the dominance of a family (Engraulidae). This dominance could be the result of the effect of various physical-chemical factors, among which salinity, transparency and the reproductive events of the species present in the area stood out, what allows to infer that in general this section of the gulf (external area) is an important area as a nursery for species. It would be interesting to carry out more studies and with longer samplings to check the behavior of the system in a longer window of time.