INTRODUCTION
Currently, algae turfs are the most abundant benthic component in most coral reefs (Gorgula and Connell, 2004; Fricke et al., 2011; Harris, 2015), a phenomenon confirmed for some continental and insular reefs of the Colombian Caribbean (Gómez-Cubillos et al., 2019; in press).
This global trend is in response to various disturbances of anthropogenic origin (Jackson et al., 2014), which in sum have reduced the capacity of corals to recover, but which have favored the proliferation of algae turfs at the expense of reef builders (Roy, 2004; Barott et al., 2012; Bender-Champ et al., 2014). This phenomenon has been considered a “phase change”, where coral reefs are now dominated by algal communities (Jompa and McCook, 2003a; McManus and Polsenberg, 2004), particularly by turfs, ubiquitous assemblages that can act as facilitators of this change (Barott et al., 2012), since once the turfs are established after coral mortality, they limit the natural regeneration of coral tissue and larval recruitment (Hughes, 1966).
Turfs are mixed assemblages of algae, densely aggregated, with a canopy height of no more than 2 cm and composed of a wide variety of groups that include cyanobacteria and red, green, and brown algae (Bender-Champ et al., 2014; Connell et al., 2014; Harris, 2015). Depending on the habitat where they develop, they exhibit a great variety of morphologies, being the filamentous forms (corticated and not corticated), the small foliaceous, and the articulated calcareous ones the most frequent (Connell et al., 2014; Cetz-Navarro et al., 2015). Compared with other functional forms such as fleshy algae (calcareous and non-calcareous) and crusty algae, turfs have competitive advantages that allow them to colonize the available space more quickly (Díaz-Pulido and McCook, 2002), as they have high growth rates (Littler et al., 2006), be less vulnerable to disturbances (Hay, 1981) and thrive in environments with nutrient overload and high sedimentation (Airoldi and Virgilio, 1998; Gorgula and Connell, 2004). Likewise, experimental studies have shown that, in warmer and more acidic waters, turfs obtain better photosynthetic rates and higher growth; that is, their physiology is positively influenced by the warming and acidification of the oceans (Kroeker et al., 2013; Ober et al., 2016; Johnson et al., 2017).
Turfs play important ecological roles in reefs since they are the main source of primary productivity (Bellwood et al., 2004; Carpenter and Williams, 2007), they facilitate the recycling of nutrients (Copertino et al., 2005; Gowan et al., 2014) and are habitat and food for numerous species of invertebrates and fish (Harris, 2015). However, it has also been shown that they have a negative impact since as a populated substrate they limit the successful establishment of coral larvae and other organisms (Garzón-Ferreira et al., 2002; Airoldi, 2003; Birrell et al., 2005, 2008; Hughes et al., 2007). Also, they are the main competitor against hard corals (Roy, 2004; Barott et al., 2012; Harris, 2015; Swierts and Vermeij, 2016) because, when the coral skeletons are exposed due to previous injuries, the turfs quickly colonize them, and once they are established, they interact with the adjacent coral tissue causing their slow and progressive death, a mechanism that varies according to the coral species involved (Gómez-Cubillos, 2018).
Additionally, Roy (2004) suggests that in areas with high sediment load and resuspension, there is an additional problem for corals, since, at the edges of living coral tissue interacting with turfs, the algal filaments slow down water flows, trapping sediments and forming algae mats with sediments, which discourage the action of herbivores and reduce the competitive capacity of different forms of coral growth to evade and/or directly combat turfs (Zhiheng et al., 2019), which in the future it generates progressive death of the adjacent coral tissue. In this regard, Nugues and Roberts (2003) found that, in the reefs of Santa Lucia, the increases in sedimentation allow the turfs to cover the corals more quickly, and that the sediments retained by the turfs suffocate and kill the adjacent tissue, mainly during the rainy season. The foregoing shows that turfs with sediments play a key role in coral mortality, and justifies the need to structurally characterize the community of algal turfs to establish whether the structure of these assemblages influences their ability to retain sediments.
In this sense, it is possible to suggest that the abundance of algae turfs is evidence of the disturbances that affect reefs, since they are maintained and proliferate in conditions that are stressful for corals and other calcifying organisms (Díaz-Pulido and McCook, 2002; Airoldi, 2003; Barott et al., 2012; Bender-Champ et al., 2014). Also, the dynamics of coral-turf interactions represent an indicator of the resilience and future of coral communities, since not only the presence of turf affects corals, but also their species composition (Jompa and McCook, 2003b).
Even though in recent years the number of world reports on the presence of algal turfs expanding at the expense of hermatypic corals has increased (Connell et al., 2014; Harris, 2015), these assemblages have been poorly characterized due to to their high diversity, the reduced size of the species that compose them, their morphological plasticity and alternation of life cycles (Díaz-Pulido and McCook 2002; Fricke et al., 2011). Therefore, their study is limited to generalized records as a homogeneous functional group, and not as a community, which fulfills various ecological functions depending on its composition (Harris, 2015). However, Díaz-Pulido and McCook (2002) and Fricke et al. (2011) estimated that in reefs of the Great Barrier Reef and Curaçao, the composition of algal turf assemblages may include at least 35 different taxa.
Recognizing the ecological importance and knowledge gaps about turfs, in this study the structural characterization of the assemblages that grow on dead parts of living colonies of four species of morphologically contrasting massive corals was carried out, in two reefs of the Tayrona National Natural Park (Tayrona NNP), Colombian Caribbean. This to relate the structural complexity of these assemblages with the sediment retention capacity, and to lay the foundations to interpret the conditions in which these turfs advance or recede in the frontier of interaction with the living tissue of the corals that have colonized (Gómez-Cubillos, 2018).
This research contributes to the knowledge about the diversity of algae in the country and presents the first quantitative references on the structure and quantity of sediments retained by algae turfs that grow on coral skeletons in Colombian reefs.
STUDY AREA
The coral reefs that border the rocky coastline of the Tayrona NNP (Figure 1, 11° 12′-11° 22′ N and 73° 57′-74° 15′ W) are not very wide, of a fringing type, and mainly made up of massive corals. hemispherical. Despite a large number of hard bottoms suitable for the establishment of reefs, the coral formations in this protected area are poorly developed, and their growth is limited compared to other areas of the Colombian Caribbean, due to the strong influence of a climatic regime. Bimodal that oscillates between coastal outcrop during droughts and continental discharges during rains (Zea, 1994). Four climatic seasons are distinguished: major outcrop (December to April), no minor outcrop (May to June), minor outcrop or summer of San Juan (July to August), and no major outcrop (September to November) (Bayraktarov and Wild, 2014).
This climactic sequence determines the algal composition, especially due to the proliferation of macroalgae between January and April, which then decrease or disappear with the increase in water temperature between September and November (Bula-Meyer, 1990), leaving the hard substrates that do not have been colonized by macroinvertebrates, covered by turfs and crusted algae (Díaz-Pulido and Garzón-Ferreira, 2002).
The history of local disturbance that these reefs have experienced in the last 50 years has been a key factor in changes in coral cover (see compilation in Friedlander et al., 2014) and increase in algal cover, mainly of algae turfs (Vega-Sequeda et al., 2008), which are estimated to have had an average annual increase of 5.6 % in the last 14 years (Acosta et al., 2018), and which are currently the most abundant benthic component (> 39 %) and the main competitor (58.7 %) for space with scleractine corals (Gómez-Cubillos et al., 2019).
MATERIALS AND METHODS
Structural characterization of algal turfs
Between November 2016 and September 2017, the algal community was monitored growing on 120 massive coral colonies in two reefs, located in Ensenada Granate (depth 10-16 m) and Bay of Nenguange (depth 4-6 m) (Figure 1; Table 1). In each reef, 20 colonies of four morphologically contrasting coral species were marked (Montastraea cavernosa and Pseudodiploria strigosa in both seasons, Stephanocoenia intersepta in Granate, and Siderastrea siderea in Nenguange) that had part of their skeleton covered by turf, and where the presence was evident of active coral-turf interactions (Gómez-Cubillos et al., 2019).
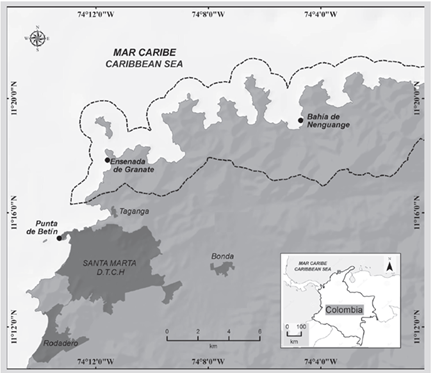
Source: Felipe Valencia, GIS specialist
Figure 1 Location of the stations in the Tayrona NNP, Colombian Caribbean. The dotted line corresponds to the polygon that delimits the protected area.
The characterization of the turf assemblages was carried out using the methodology proposed by Connell et al. (2014) and Harris (2015). In the first campaign, near the edge of interaction with living coral tissue, two cores of a coral skeleton covered with turf were extracted per colony, using a hammer and a 27 mm diameter metal impact punch (5.7 cm2) (Márquez and Zea, 2012). The first core was used to determine the composition and abundance by algal taxon, canopy height, cover, and apparent filament density, and the second core was used to determine algal biomass.
In the second campaign, during the macroalgal growth season, the macroalgae present (if any) were manually collected on the coral-turf interaction edges of all the marked colonies. In the last campaign, when the assemblies were made exclusively of turfs, on each interaction edge, a rectangular rubber frame (rim tire) of 4.5 cm2 (3 cm long × 1.5 cm wide, simulating a surgical field), and with a 20 cm3 syringe the sediments accumulated by the turfs in the area delimited by the device were carefully sucked out.
Turf-extracted cores and macroalgal samples were fixed in 4 % formalin solution in seawater, neutralized with hexamethylene-tetramine (20.g·L-1), and stored in labeled plastic jars. The contents of the syringes were transferred to 100 ml plastic containers, and the sediments were allowed to settle for 48 h in distilled water to remove salts, then the excess water was removed and the residue was processed.
To estimate the composition and coverage (%) by algae species, the samples were wet-checked with a Leica-S6E stereoscope (1.0-4.0 X). By nucleus, coverage (%) was estimated by individual morphotype, categorizing in increments of 5 % (Fricke et al., 2011) concerning the total area of the nucleus. A sample of each morphotype was preserved in individual plastic vials with neutralized 4 % formalin, and photographs were taken with a Leica-DM750 optical microscope with a MiniVDD-CM500 digital camera. Most of the morphotypes were identified to the genus level using the taxonomic keys of Taylor (1960), Littler and Littler (2000), and Wynne (2011, 2017). This taxonomic level was only reached due to the reduced size of the filaments and/or the absence of fertile structures or other morphologically important characteristics for their identification at the species level.
To estimate the height of the canopy, it was decided to use a stainless steel ruler with a millimeter scale, and measure three algal filaments at random. The values obtained were averaged to give a single value per core. Then, by the nucleus, the coverage (%) of the categories of algae turfs, crusted algae, and the bare skeleton was visually estimated (Purcell and Bellwood, 2001), and the apparent density of turf filaments or degree of aggregation of thallus and branches was estimated. From the categories: I) densely aggregated mats, with a homogeneous appearance, made up of short filaments (~ < 2 mm); II) moderately dense mats with short and long filaments (~ 2-5 mm), and III) sparse and tall mats (~ > 5 mm) of erect and prostrate branches.
To determine the algal biomass, the method proposed by Fricke et al. (2011). Each core was wet-checked using a stereoscope (1.0-2.0 X). First, the organisms other than turf were removed (annelids, sponges, crusted algae, among others), and with dental instruments (explorers, curettes, and teaspoons) the filaments of the turf were scraped. The material obtained was decalcified with 10 % HCl for 1 h, and the solution was sieved (180 µm and 70 µm filters) to remove coral and terrigenous remains. The residue was weighed with an OHAUS-PA313 analytical balance on a previously weighed paper filter. The sample was kept in an oven for 3 days at 60 °C and then weighed. The algal biomass (g·cm-2) was calculated by dividing the value of the dry weight of the sample by the sampled area (5.7.cm-2).
To determine the amount of sediment accumulated by turfs, the sediment residue obtained by decantation was separated into two fractions (sand and silt) using a 63 µm sieve. Each fraction was dried in an oven for 2 days at 105 °C and weighed. The total amount of sediment per sample and per fraction (g·cm-2) was calculated by dividing the weight of the dry sample by the sampled area (4.5 cm-2).
Analysis of data
The variables evaluated were characterized by descriptive statistics and box-and-whisker diagrams. Then, for the continuous quantitative variables, the assumptions of normality (Shapiro-Wilk) and homogeneity of variances (Levene’s) (Underwood, 1997) were tested.
By reef and type of substrate (coral species), a matrix was made with the frequency (percentage relationship between the number of times the morphotype is recorded and the total number of nuclei per coral species evaluated) and coverage (%) of the genera of Identified turf algae. The coverage data were transformed using the arcsine formula (Sokal and Rohlf, 1981).
To establish differences in the composition of turf assemblages between reefs and substrates, with the transformed coverage matrix and the Bray-Curtis similarity index, a two-way Analysis of Similarity (ANOSIM) and a Percentage of Similarity Analysis (SIMPER) were performed (Clarke and Warwick, 2001), using the Primer 6 version 6.1.13 program at a 95 % confidence level.
Utilizing non-parametric tests of Mann-Whitney (M-WU), Kruskal-Wallis (KW), and posthoc (Agresti, 2002) statistical differences between reefs and substrates were determined regarding the height of the canopy, algal biomass, and total amount of accumulated sediment. With contingency tables and Chi2 independence tests (Sokal and Rohlf, 1981) the association between the reef and the substrate was identified with the variables expressed in percentages (coverage by categories, the density of filaments, and a fraction of sediments).
Using Spearman correlations (González and Pérez, 2009), the interdependence between the total amount and the fraction of accumulated sediments on the edges of coral-turf interaction was identified in a general way and by a reef, with the variables coverage (%) by taxa, canopy height, and algal biomass. The analyzes were performed with the Statgraphics Centurion XVI program version 16.1.18. at a 95 % confidence level.
RESULTS
Composition, presence of diagnostic taxa, and persistence of the assembly
Thirty taxa were identified at the genus level, belonging to 13 orders and 18 families (Table 2). The Rhodophyta were the most diverse algae (20 genera), followed by the Chlorophyta (five genera), the Phaeophyceae (four genera), and the cyanobacteria (one genus and three morphotypes). Also, the presence of the Anotrichium and Corallophila genera, taxa recognized as harmful to corals, was recorded (Díaz-Pulido and McCook, 2002; Jompa and McCook, 2003b).
Table 2 Taxonomic list of genera and possible species [tentative identification] that make up the assemblages of algae that interact with the living tissue of massive corals in reefs of the Tayrona NNP, Colombian Caribbean. In each reef, the first date corresponds to algae turfs (coralline skeleton nuclei), and the second to macroalgae that covered the interaction edges during the macroalgal flowering season. † Tax already registered in the Tayrona PNN by Díaz-Pulido and Díaz-Ruíz, 2003. ‡ Presence of reproductive structures.
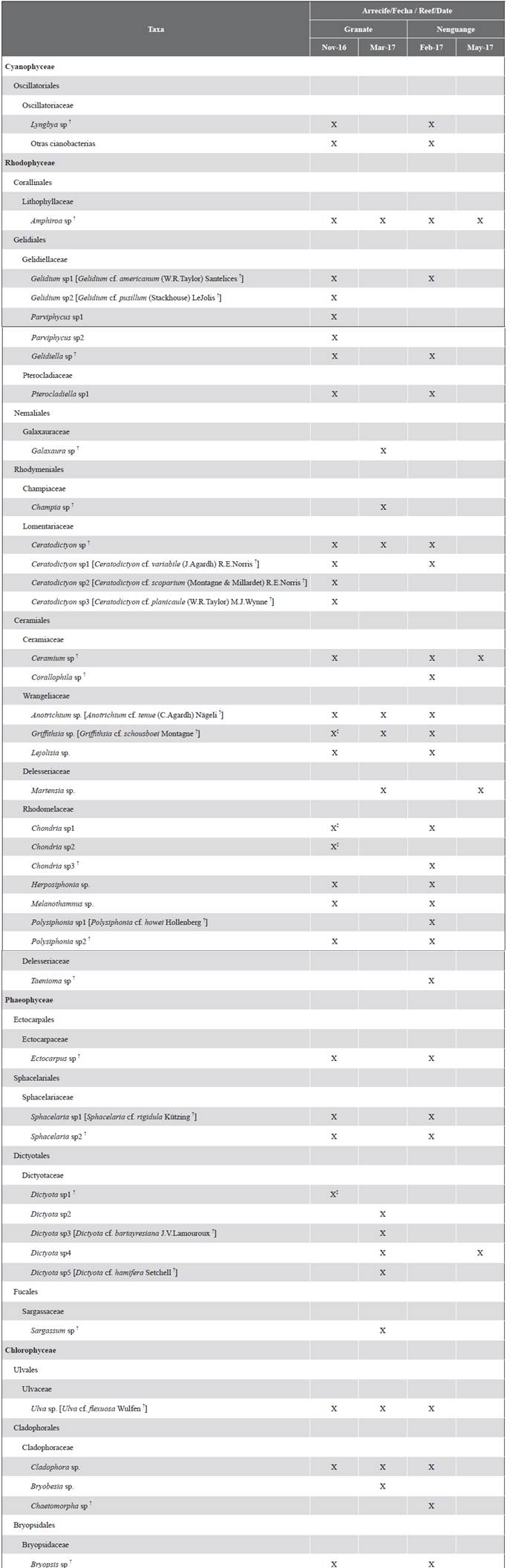
In Granate, the turfs were composed of 23 genera (Table 2). In all substrates or coral skeletons, Amphiroa and Gelidium were the most frequent genera, and in 61 % of the skeletons of S. intersepta the genus Dictyota was found. The genera Corallophila, Taenioma, and Chaetomorpha were not recorded on this reef (Figure 2A).
In Nenguange, the turfs were made up of 24 genera (Table 2). In general, Polysiphonia, Chondria, and Herposiphonia were the most frequent genera in the three types of substrates. Additionally, on skeletons of M. cavernosa, the genera Ceramium, Sphacelaria, Cladophora, and Gelidium, and skeletons of P. strigose, the genera Amphiroa and Gelidiopsis registered a frequency higher than 50 %. The genera Parviphycus and Dictyota were not recorded on this reef (Figure 2B).
ANOSIM showed that between reefs there are differences in the coverage (%) of the genera that make up the assemblages of turf (nuclei), but with overlapping groups (Rglobal = 0.46; significance level 0.1 %), while There are no statistical differences between substrates (Rglobal= 0.12; significance level 0.1 %).
The SIMPER per reef (Table 3), showed that in Granate the turf assemblages were made up of more than 80 % of foliaceous (Dictyota), erect corticated (Gelidiales), and articulated calcareous (Amphiroa) algae, but of small size (Figure 2A). On the contrary, in Nenguange the main contribution was made by filamentous algae (Polysiphonia, Herposiphonia, and Sphacelaria) and small erect corticates (Chondria and Gelidiales) (Figure 2B). Also, it was identified that 10 genera contributed more than 50 % of the dissimilarity between stations (Table 3).
Table 3 Turf algae genera that define similarity within and dissimilarity between seasons. Results obtained from SIMPER. Conventions: AP = average abundance. SP = average similarity. Sim = Similarity. SD = Standard deviation. CTB = Percentage contribution. ACM = Accumulated percentage.
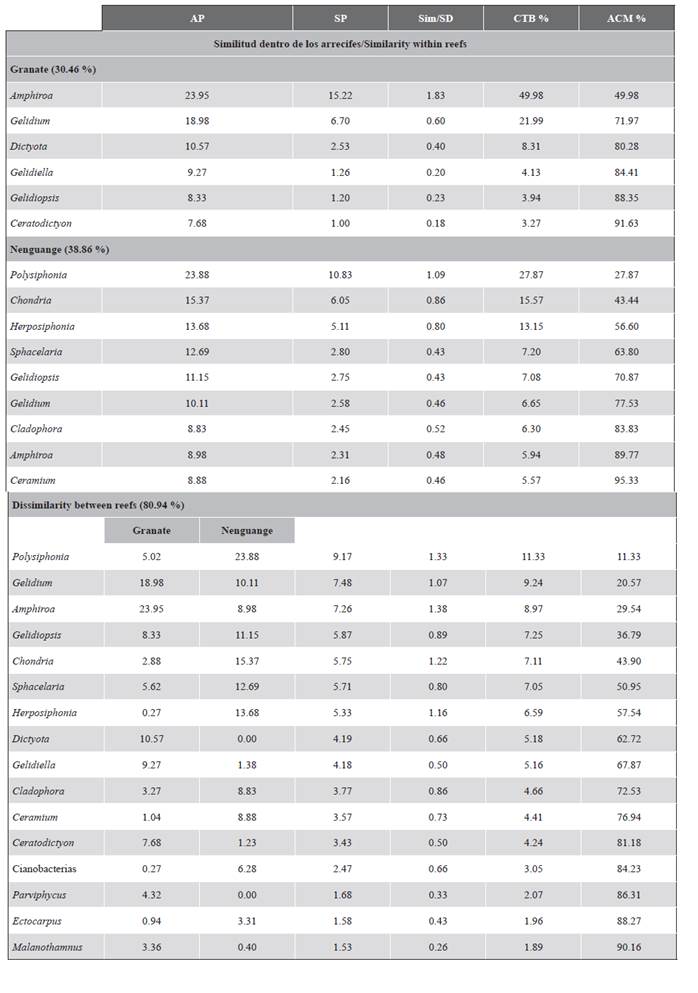
In 2017, during and immediately after the months of the coastal outcrop (March to May), when a visual inspection of the marked colonies was made and the macroalgae that covered the interface with living coral tissue were manually collected, only in Granate a transition from turfs to macroalgae beds. This new assemblage in Granate consisted of 13 taxa (Table 2), of which 8 genera had already been found in the turf samples, but now with bushy growth; five additional genera were recorded (Galaxaura, Champia, Martensia, Sargassum, and Bryobesia), and Dictyota and Amphiroa were confirmed to be the dominant genera (Figure 2C). It is clarified that in September 2017 these macroalgae disappeared, re-dominating the algal turfs (which were not quantified again). In Nenguange, on the other hand, only one colony of P. strigosa was colonized by macroalgae in May 2017, with turfs being the dominant assemblage throughout the research period (February to September 2017).
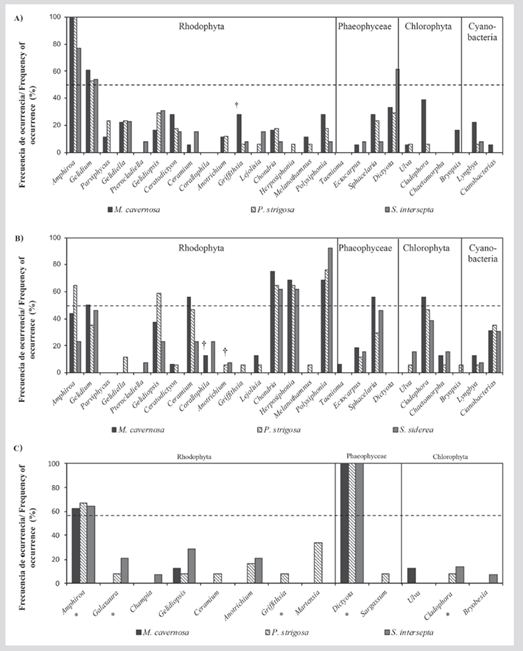
Figure 2 Frequency of occurrence (%) of the genera that make up the algal assemblages that grow on coral skeletons, in the reefs A) Garnet (n = 48 colonies; Nov 2016); B) Nenguange (n = 46; Feb 2017) and C) Garnet (n = 33; Mar 2017). A) and B) correspond to the turfs found in the cores, and C) to the manually collected macroalgae that covered the interaction edges during the macroalgal bloom (Nenguange is not included because the macroalgal bloom there was minimal). The crosses (†) indicate taxa recognized as damaging to corals and the asterisks (*) the genera found only during macroalgal bloom. The dotted line represents the frequency cutoff at 50 %.
Canopy height
No significant differences were found in canopy height between reefs (M-W U = 8575.0; nGranate= 144; nNenguange= 138; P < 0.05). In Granate the average height of the turf was 5.1 ± 0.20 mm (variations between 2.0 and 16.0 mm), and in Nenguange it was 4.5 ± 0.17 mm (variations between 1.0 and 12.0 mm).
According to the substrates, in Granate, no differences were found (KW = 0.6; P > 0.05), but in Nenguange there were (KW = 14.1; P < 0.05), since the turfs on skeletons P. strigosa were statistically higher than on skeletons of M. cavernosa and S. siderea (Figure 3).
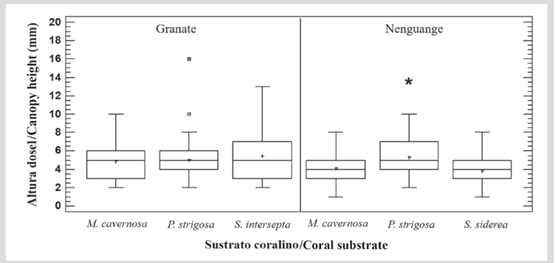
Figure 3 Canopy height (mm) of turfs growing on coral skeletons in portions adjacent to interactions with living coral tissue, in the Granate and Nenguange reefs (PNN Tayrona). The horizontal bars indicate the medians, the boxes the first and third quartiles, the whiskers the data range, the cross (+) the average, the squares the extreme values, and the asterisk (*) indicates which substrate had a significantly different average.
Coverage and apparent density of filaments
In general, the percentage of coverage in the cores of the three categories defined a priori (algae turfs, crusted algae and bare skeleton) varied between reefs (Chi2= 6061.5; P < 0.05; GL = 186) and between substrates (Granate: Chi2= 3110.4; P < 0.05; GL = 94 and Nenguange: Chi2= 1888.8; P < 0.05; GL = 90).
However, the individual analysis by categories showed that the turf cover, although it varies between reefs (KW = 23.4; P < 0.05) does not vary between substrates (Granate: KW = 1.8; P = > 0.05 and Nenguange: KW = 0.7; P = > 0.05). In Nenguange, turfs occupied 84.3 ± 2.3 % (M. cavernosa= 78.8 ± 5.3 %, P. strigosa= 87.1 ± 2.7 and S. siderea= 87.1 ± 2.6 %), while, in Granate, they represented 61.9 ± 3.5 % (M. cavernosa = 61.7 ± 5.8 %; P. strigosa = 67.1 ± 5.7 % and S. intersepta= 55.4 ± 6.8 %) (Figure 4A).
Differences between reefs were also found for crusted algae (K-W = 10.4; P < 0.05). Between substrates, differences were only found in Granate (KW = 6.8; P = < 0.05), where this coverage represented 24.2 ± 4.1 %, being significantly higher on skeletons of S. intersepta (36.2 ± 8.2 %), compared to M. cavernosa (25.0 ± 6.4 %) and P. strigosa (14.1 ± 6.3 %). In Nenguange no differences were found between substrates (KW = 1.7; P = > 0.05), and these algae represented only 6.5 ± 1.9 % of the nuclei coverage (M. cavernosa = 10.6 ± 4.2 %; P. strigosa = 4.7 ± 2.7 % and S. siderea = 3.8 ± 1.8 %) (Figure 4A).
For the bare skeleton category, no differences were found between reefs (KW = 0.9; P > 0.05) or between substrates (Granate: KW = 3.7; P = > 0.05 and Nenguange: KW = 0.9; P = > 0.05). In Granate, this category represented 14.0 ± 2.7 % of the nuclei coverage (M. cavernosa = 13.3 ± 5.2 %; P. strigosa = 18.8 ± 4.6 % and S. intersepta = 8.5 ± 3.6 %), and in Nenguange 9.1 ± 1.7 % (M. cavernosa = 10.6 ± 3.8 %; P. strigosa = 8.2 ± 2.1 % and S. siderea = 8.5 ± 2.7 %) (Figure 4A).
The independence tests showed that the filament density depends on the reef (Chi2 = 296.5; P < 0.05; GL = 10) and the coral substrate (Granate: Chi2 = 36.2; P < 0.05; GL = 4 and Nenguange: Chi2 = 82.4; P < 0.05; GL = 4). In Granate, moderately dense rugs (45.8 %) and tall scattered rugs (39.6 %) dominated, while dense and short rugs only represented 14.6 %. In contrast, in Nenguange dense and short turfs dominated (65.2 %), while moderately dense and sparse tall rugs accounted for only 21.7 % and 13.0 %, respectively (Figure 4B).
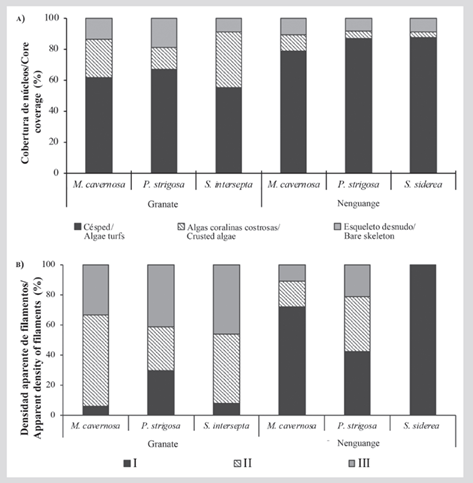
Figure 4 A) Cover (%) of algae turfs, crusted algae, and bare skeleton, and B) density of algae filaments (%) in cores collected near coralturf interfaces, in the Granate and Nenguange reefs (Tayrona PNN). Conventions for density: I - short and densely aggregated filaments (~ < 2mm); II - moderately long and bushy filaments (~ 2-5 mm), and III - tall and sparse filaments (~ > 5 mm). The % coverage corresponds to the combined value for all nuclei per substrate (coral species). The % density is the result of the number of designated nuclei in each category concerning the total of nuclei per substrate.
Algal biomass
No significant differences were found in algal biomass between reefs (M-WU = 1120.0; nGranate= 49; nNenguange= 46; P > 0.05), but there were between substrates (Granate: KW = 10.9; P < 0.05 and Nenguange: KW = 19.5; P < 0.05). In Granate, the algal biomass was higher on skeletons of P. strigosa, and in Nenguange it was significantly lower on skeletons of S. siderea (Figure 5).
In Granate, the average algal biomass was 0.012 ± 0.002 g·cm-2 with variations between 0.077 and 0.002 g·cm-2 (M. cavernosa= 0.007 ± 0.001 g·cm-2; P. strigosa= 0.021 ± 0.005 g·cm-2 and S. intersepta = 0.008 ± 0.003 g·cm-2), and in Nenguange it was 0.010 ± 0.001 g·cm-2 with variations between 0.036 and 0.002 g·cm-2 (M. cavernosa = 0.012 ± 0.002 g·cm-2; P. strigosa= 0.013 ± 0.002 g·cm-2 and S. siderea = 0.004 ± 0.001 g·cm-2) (Figure 5).
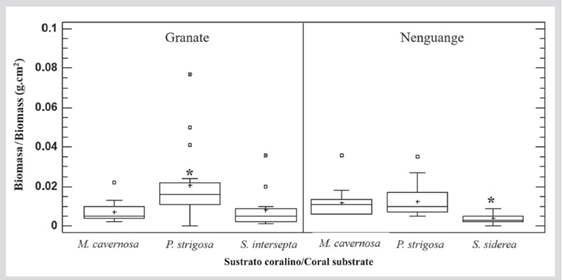
Figure 5 Algal turf biomass (g·cm-2) that grows on coral skeletons in the Granate and Nenguange reefs (PNN Tayrona). The horizontal bars indicate the median, the boxes the first and third quartiles, the whiskers the range of the data, the cross (+) the average, the squares the extreme values, and the asterisks (*) indicate the substrates with significantly different averages.
Sediment accumulated at the edges of coral-turf interaction
Statistical differences were found in the total amount of accumulated sediments between reefs (M-WU = 777.5; nGranate = 60; nNenguange = 58; P < 0.05) and substrates (Granate: KW = 20.9; P < 0.05 and Nenguange: KW = 6.3; P < 0.05), being on average higher in Granate (0.075 ± 0.003 g·cm-2, with variations between 0.045 and 0.173 g·cm-2) than in Nenguange (0.056 ± 0.003 g·cm-2, with variations between 0.020 and 0.147 g·cm-2), and significantly more abundant on P. strigosa skeletons (Granate = 0.094 ± 0.007 g.cm2; Nenguange = 0.065 ± 0.006 g.cm2) than on skeletons of M. cavernosa (Granate = 0.067 ± 0.003 g.cm2; Nenguange = 0.053 ± 0.003 g.cm2), S. intersepta (Granate = 0.062 ± 0.003 g.cm2) and S. siderea (Nenguange = 0.049 ± 0.002 g.cm2) (Figure 6A).
According to the grain size, the independence test determined that the proportion between fractions also depends on the reef (Chi2 = 1172.2; P < 0.05; GL = 117) and the substrate (Granate: Chi2 = 506.1; P < 0.05; GL = 59 and Nenguange: Chi2 = 633.5; P < 0.05; GL = 57). On average, silt-clays are more abundant (Granate = 86.1 ± 1.3 %; Nenguange = 83.0 ± 1.6 %) than sands (Granate = 13.9 ± 1.3 %; Nenguange = 17.0 ± 1.6 %), and on skeletons of S. intersepta (Granate) and S. siderea (Nenguange) a lesser amount of coarse material accumulates compared to M. cavernosa and P. strigosa (Figure 6B).
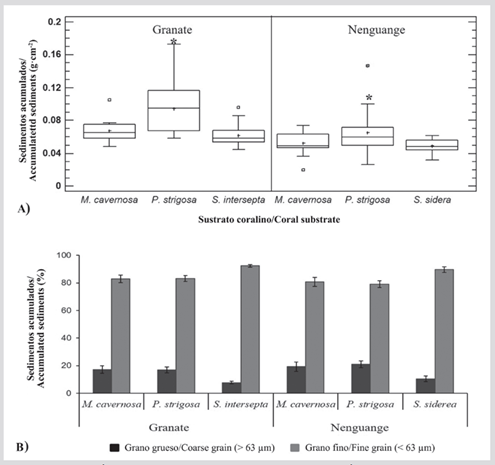
Figure 6 Sediments (g·cm-2) accumulated at the edges of the coralturf interaction, in the reefs of Granate and Nenguange Tayrona NNP: A) Total sediments, the horizontal bars indicate the median, the boxes the first and third quartiles, the whiskers the data range, the cross (+) the average, the squares the extreme values and the asterisks (*) the substrates with significantly different average. B) According to the grain size (%) as coarse fraction/sand (> 63 μm) and fine fraction/silt-clays (< 63 μm).
Association between accumulated sediments with the structure of turfs
In general, it was found that there is a positive correlation between the total amount of sediments accumulated at the edges of coral-turf interaction with the algal biomass (r = 0.23; P < 0.05; n = 91), particularly when the fraction of the sediment is fine (r = 0.32; P < 0.05; n = 91).
According to the reef, in Granate a positive correlation was found between the biomass with the total amount of sediments (r = 0.34; P < 0.05; n = 47) and the amount of silt-clays (r = 0.31; P < 0.05; n = 47), but in Nenguange, the variables that were positively correlated were the height of the canopy with the accumulated silt-clays (r = 0.31; P < 0.05; n = 44).
According to the cover by genus, in the two reefs it was found that the amount of total sediments has a positive correlation with the cover of articulated and corticated calcareous algae (rAmphiroa = 0.24; rGelidiella = 0.24; P < 0.05; n = 91), but it has a negative correlation with the cover of filamentous algae (rCeramium = -0.23; rPolysiphonia = -0.26; P < 0.05; n = 91) and cyanobacteria (r = -0.31; P < 0.05; n = 91).
According to the grain size, it was found that a greater accumulation of fine sediments has a positive correlation with a greater coverage of Ceramium (r = 0.24; P < 0.05; n = 91), while a greater accumulation of coarse sediments have a positive correlation with a greater coverage of articulated, corticated and leafy calcareous algae (rAmphiroa= 0.25; rGelidiella = 0.31; rDictyota = 0.23; P <0.05; n = 91), but it has negative correlation with the coverage of some filamentous genera (rCeramium = -0.39; rHerposiphonia = -0.37; rChondria= -0.42; rPolysiphonia = -0.37; rCladophora = -0.22; P < 0.05; n = 91) and cyanobacteria (r = -0.35; P < 0.05; n = 91).
DISCUSSION
Although algae turfs appear homogeneous at first glance, there is evidence that these assemblages are highly variable on a spatial scale (Harris et al., 2015), depending on the location, depth, and disturbances that affect the reef (Bonaldo and Bellwood, 2001); variables that together are determinants for the succession processes in these communities (Díaz-Pulido and McCook, 2002; Fricke et al., 2011). Although Harris et al. (2015) suggest that this structural variability is greater on a small scale (cm) than between separate reefs (from m to km). This research showed that between stations (separated by km) and type of colonized substrate (colonies separated by m), There are important structural differences and that this variability, expressed in terms of structural complexity, gives algal turfs certain competitive advantages in interactions with living coral tissue, such as, for example, a greater capacity to retain and accumulate sediments in their fronds.
Among reefs, the most important structural variables were the composition and cover of the algal genera, the relative cover by categories (turfs, crusted algae, and bare skeleton), the density of filaments, and the total amount and by a fraction of sediments. While, among colonized substrates, the coverage by categories, particularly of crusted algae, the density of filaments, biomass, and the total amount and by a fraction of sediments varied.
In general, the turfs that colonize massive coral skeletons in Granate form discontinuous mats of tussock algae that share the substrate with patches of crusty algae. These turfs are made up of tall, erect, and prostrate branches of articulated calcareous algae, small corticates, and juvenile hardwoods, especially Dictyota. This genus determines the transitional character of these assemblages, since, during the months of the coastal outcrop, mats with high and dense canopies develop and form, which invade the edges of coral-turf interaction, until environmental conditions again favor the dominance of the turfs. In contrast, the algal turfs in Nenguange appear to be perennial or permanent over time, they develop as short, very dense, and dense mats, with little presence of crusted algae, and are made up of filamentous and small corticates algae.
Initially, these differences can be attributed to the topographic characteristics of each reef, its proximity to the area of influence of the urban/port area of Santa Marta and the depth of sampling. According to Fricke et al. (2011), as the depth increases the assemblages of turfs tend to be structurally more complex. However, Harris et al. (2015) argue that herbivory is the process that exercises the greatest control over the abundance and distribution of these algal communities.
In this regard, Burkepile and Hay (2010) mention that turfs made up of simple taxa, generally filamentous forms that are easily digested, indicate strong grazing pressure, a process that keeps turfs in early stages of succession, a hypothesis that would explain why in Nenguange the turfs are shorter, dense and dominated by small filamentous algae. For their part, Díaz-Pulido and McCook (2002) and Fricke et al. (2011) argue that in reefs with reduced herbivory, turfs increase in coverage and biomass, and tend to progress towards more complex morphological assemblages, including macroalgal juvenile stages. This assumption would support the results found in Granate, where mats dominated discontinuous of tall turfs between crusted algae, formed by articulated calcareous algae, small corticates, and juvenile hardwoods. These hypotheses coincide with the results obtained by Díaz-Ruíz (2010), who affirms that in the reefs of the Tayrona NNP, herbivory is a key factor that regulates the coverage, composition, and succession of the algal communities, and that particularly in Granate a greater abundance of turfs is due to lower grazing pressure.
Although this study was limited to evaluating the structure of the turfs that interact with living coral tissue, it is important to mention that, in all the samplings, annotations were made on the presence of damselfish territories of the genus Stegastes in the 120 evaluated colonies, finding that these territories are more frequent in Nenguange (59 %) than in Granate (16 %) and that these fish prefer to cultivate their gardens on colonies of S. siderea and S. intersepta (Gómez-Cubillos, 2018).
This finding suggests that the presence of damsel territories is a key factor that modulates the structural complexity of turfs. In this regard, Jones et al. (2006) and Harris (2015) indicate that these fish select easily digestible filamentous taxa for their gardens on calcified algae and juvenile macroalgae, and that generally these turfs are kept trimmed and in early or intermediate stages of succession (Fong and Paul, 2011). Furthermore, the presence in the two reefs of a bare skeleton or limestone rock visible in the nuclei analyzed, can be interpreted as recent herbivore bites, which according to Wanders (1976) reflects a selective grazing pressure towards certain taxa, perhaps directed towards the filamentous forms that grow under tall, complex and less palatable fronds.
For the Tayrona NNP, Acosta et al. (2018) report a progressive annual decline in fish biomass (g/m2), particularly in the Granate and Nenguange reefs. Therefore, it is expected that the potential effect of these changes in the structure of fish communities, especially in the abundance of herbivores, can promote faster successions in turfs, making them less palatable and resistant to grazing, denser and morphologically more complex, and gives them a greater capacity to accumulate and retain sediments, probably with higher microbial loads (Morrison, 1988; Harris, 2015), which in sum would make these turfs more damaging in interactions with living coral tissue.
On grazing dynamics, Arthur et al. (2005), argue that turfs exposed to low herbivory may eventually be replaced by macroalgae. However, this research confirms that in Granate the genus Dictyota in its juvenile stage is an important component in the assemblages of turf, but once the water temperature decreases during the coastal outcrop (Bula-Meyer, 1990), these algae develop and form dense mats. Therefore, the transitional character of these assemblages should not be assumed as an indicator of low pressure due to grazing, but rather as a seasonal response of the algae to oceanographic changes during these months. On the contrary, in Nenguange, the permanent condition of the turfs over time, the absence of macroalgae during the entire research period, and the low cover of crusted algae on the coral skeletons suggest that certain environmental conditions exist on this reef and/or biological, still unknown, that are limiting the recruitment and settlement of macroalgae and crusted algae, and that probably the turfs or certain taxa that comprise it, act as inhibitors for the development of canopy algae and with crusty growth, a phenomenon already described by Kennelly (1987) and Airoldi (2003) in subtropical environments.
Another important variable in the differentiation of turfs is their capacity for vegetative growth (Harris et al., 2015). In this study, only 6 % of the samples analyzed had reproductive structures observed in algae of the genera Griffithsia, Chondria, Sphacelaria, and Dictyota. This indicates that, in the two reefs, vegetative propagation was the most frequent growth mechanism of the turfs during the evaluated period, without ignoring that there may be reproductive seasonality mediated by the coastal outcrop. According to Sousa (1979) and Airoldi et al. (1995), this form of propagation is an important competitive advantage, because turfs can defend the territory gained, by preventing the development of other algae and organisms that colonize with spores and planula, allowing them to capture a greater amount of sediment, which in turn, would serve as a substrate to anchor and advance laterally, covering the adjacent polyps, and allows them to tolerate physical stress due to burial and abrasion, recovering and persisting despite disturbances.
Although there are no quantitative references in the literature on the amount of sediment that turfs accumulate at the edges of interaction with living coral tissue, this study showed that the turfs in reefs of the Tayrona NNP can accumulate up to 0.173 g.cm-2 of total sediments, a figure that represents on average up to 21 and 14 times its biomass in Granate and Nenguange, respectively. Also, it is confirmed that there is a strong association between these two variables, that is, the higher the biomass, the greater the amount of retained sediments, particularly fine sediments. This great capacity that turfs have to retain sediments may be the main competitive advantage in interactions with living coral tissue, as stated by Zhiheng et al. (2019), given that the sediment particles that trap the algal filaments when mixing with detritus and the mucus produced by the corals, form masses that hinder the photosynthetic activity of the zooxanthellae of the border polyps (Hauri et al., 2010), thus causing progressive death of the adjacent coral tissue (Jompa and McCook, 2003b; Roy, 2004).
According to Casey et al. (2014) and Harris (2015), turfs composed of morphologically more complex taxa and resistant to herbivory, and with abundant fine sediments accumulated in their fronds, creating hypoxic environments in the coral-turf interface that generate stress in the coral tissue, and they favor the colonization of microorganisms, some of them associated with coral diseases. Therefore, the temporary monitoring of signs such as loss of integumentary continuity, discoloration, and necrosis of adjacent polyps, facilitates the evaluation of a stress condition in the coral-turf interface (Gómez-Cubillos, 2018), probably caused by loaded turfs with sediment. Finally, it is clarified that according to the results of this study and other investigations carried out by the authors, it is estimated that the deleterious capacity of turfs with sediments at the edges of interaction with corals depends not only on the number of accumulated sediments but also on the sediment type since the annual rates of lateral loss of coral tissue increase significantly when a load of fine sediments (< 63 µ) with organic material increases.
In sum, the structural description of the algal turfs that interact with massive corals on reefs of the Tayrona NNP provided valuable ecological information, because it allowed demonstrating that the structure of these assemblages is variable in space-time (seasonal replacement by macroalgal growth in Granate), facilitated making inferences about grazing pressure and allowed to quantify the capacity of turfs to accumulate sediments.
This information collectively highlights the competitive advantages of these assemblages in interactions with living coral tissue. Therefore, it is suggested to consider the abundance of algal turfs as an independent indicator in the methodology of the Indicator Protocol Condition Trend of Coral Areas in Colombia (Ramírez-Rincón et al., 2014), to provide useful information in understanding the current state of reef systems, make Spatio-temporal comparisons and show changes in reef communities, due to the dynamics of these assemblages.