INTRODUCTION
Seagrasses represent less than 2 % of global cover, however, they are ten times more efficient at sequestering carbon than other terrestrial biological systems (Mcleod et al., 2011; Murray et al, 2011, Ramírez-García et al., 2019), which makes them important carbon sinks in coastal areas, this process is called Blue Carbon (Nellemann and Corcoran, 2009; Pendleton et al., 2012, Macreadie et al., 2019). Seagrass meadows store the carbon produced in their tissues (autochthonous) and from different sources (allochthonous) (seston, macroalgae, etc.), accumulating in their sediments (Duarte et al., 2005, 2010; Kennedy et al., 2010; Fourqurean et al., 2012). It is estimated that seagrasses globally sequester between 20.73 to 50.69 Tg C/year (Charpy-Roubaud and Sournia, 1990; Duarte and Cebrián, 1996; Duarte et al., 2005, 2010), which corresponds between 10 and 18 % of carbon sequestration in the oceans, with a daily estimate of 1.21 ± 0.3 g/m2 in the rhizomes and roots of the underground biomass (Duarte and Chiscano, 1999).
The fact of sequestering carbon is due to its ability to produce biomass, fulfilling a primary role in its storage in anoxic sediments, even for decades (Duarte et al., 2005), it means that if the seagrass meadow is more extensive, it will have greater carbon sequestration. Authors such as Fourqurean et al. (2012) estimate that the average carbon stored globally is 2.52 ± 0.48 Mg C/ha and of this, two-thirds correspond to the stored in rhizomes and roots, which may ultimately be buried for even millennia, turning it into a large sink of long-term carbon (Pergent et al., 1994; Mateo et al., 1997; Ricart et al., 2020). In Colombia, there are few studies related to this theme, mainly focusing on the evaluation of structural attributes without highlighting their importance as carbon reservoirs, for this reason, we sought to quantify the biomass and its role in the carbon present in two of the most representative seagrass meadows from the Colombian Caribbean, San Andrés Island and La Guajira.
STUDY AREA
The study was carried out in two regions of the Colombian Caribbean where the presence of seagrass meadows is marked. The first one is the area of La Guajira, where more than 80 % of the total seagrass in the country is found (Díaz et al., 2003). The other region was in the western Caribbean, on the island of San Andrés, a site that is part of the SeaFlower Biosphere Reserve since 2000 and has been part of a Marine Protected Area (AMP) since 2005 (Gómez-López et al., 2012).
La Guajira
This area constitutes the northernmost point of the Colombian continental platform, it has amplitudes that vary between 8 and 17 km with a bottom that increases progressively. In turn, it has an extension of seagrass meadows of 56 424 ha, basically of Thalassia testudinum (K.D. Koenig, 1805) that extends between 0 and 15 m deep (Gómez-López et al., 2014).
The sediments of La Guajira generally present carbonate content, composed mainly of remains of mollusks (bivalves and gastropods) and echinoderms (brittle stars and other organisms). The sedimentary deposits are mainly sandy, with sandy-muddy fractions towards the Sierra Nevada and muddy in Hondita Bay (Corpoguajira and Invemar, 2012). The intensity of the northeast and east winds in the area continually removes large amounts of sediment deposited in the shallow bottoms that affect the water´s transparency. Also, produces an upwelling effect, with the outcropping of subsurface waters (Gutiérrez-Leones et al., 2015).
The data in this area was carried out in two sectors: the Media Guajira, at Musichi, where an extensive meadow was sampled at a depth of 1 m; and Carrizal, a station sampled at 0.8 m. In the Alta Guajira, 6 meadows were sampled, one in Cabo de la Vela (0.5 m depth), 2 in Portete Bay (1.5 m) and 3 in Hondita Bay (1 m) (Fig. 1).
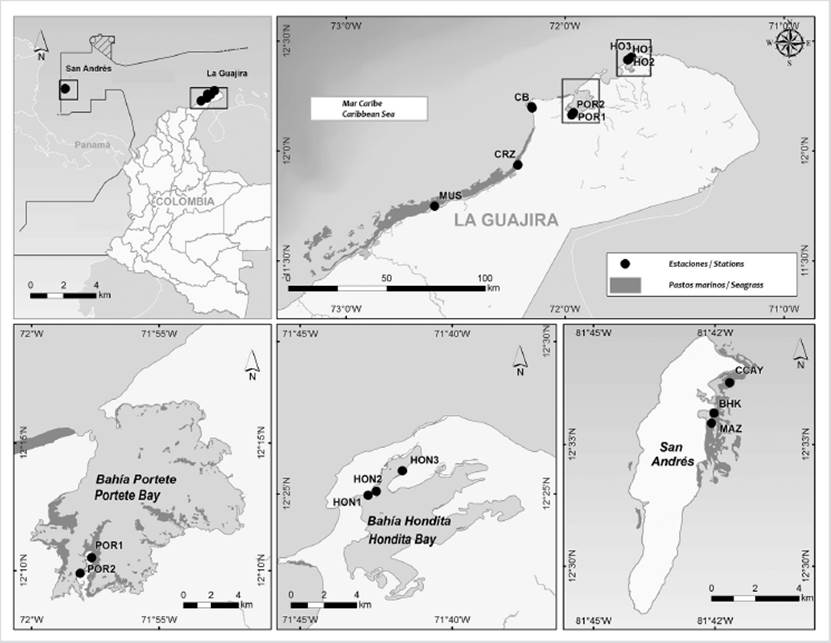
Figure 1 Sampling stations located in La Guajira and San Andrés, (Guajira with POR: Portete Bay, CB: Cabo de la Vela, CRZ: Carrizal, HON: Hondita Bay; San Andrés with CCAY: Cotton Cay, BHK: Hooker Bay, MAZ: Mar Azul) (Geographic Information Services Laboratory - Invemar, 2020).
San Andres Island
Is located in the western area of the Caribbean Sea, at about 800 km from the coast of Colombia and about 110 from Nicaragua, it has a length of approximately 12 km and a width of 4 km in its broader sector. On the windward side, it has a barrier reef that separates a side exposed to the waves and winds, and a second environment that is generally calm, where a lagoon basin of variable extension is present (Díaz et al., 1996, 2000; Gamboa et al., 2010). Fragments of corals, sea urchins, and other animals have been deposited there, as well as calcareous macroalgae of species such as Penicillus spp., Caulerpa spp., and Halimeda spp., this last, as the most representative (Díaz et al., 2003), rising the bioclastic sands that dominate as the most common type of sediment. In this sector, there are formations of seagrass meadows that consolidate the sediment layer with an approximate extension of 399 ha (Gómez-López et al., 2014).
Stations were located in Cotton Cay (2 m), Mar Azul, and Hooker Bay (both approximately at 1 m), all of them located inside the pre-reef lagoon, next to the windward side of the island (Fig. 1) and constituted predominantly by T. testudinum.
MATERIALS AND METHODS
During the second semester of 2017, using a 50 x 50 cm PVC quadrant, density data (stems/m2) were taken at each of the sampling sites following the protocols described in the SeagrassNet manual (Short et al., 2008). Biomass extraction was carried out in sectors with a high density of seagrass (> 200 stems/m2) using a PVC corer (8 cm diameter and 40 cm long) inserted through the sediment, taking special care with the leaves, and reaching the rhizomes and roots of the plants. The corer was covered at the top and extracted from the bottom, taking 3 replicates per station. Each of these was cleaned to remove particulate sediment and placed in hermetic bags in a refrigerator with ice for transfer to a laboratory.
Laboratory phase
The weight of the wet biomass (g) was obtained from each station, separating the vertical leaves and rhizomes (aerial section) and the horizontal roots and rhizomes (underground section). The samples were sent to the marine environmental quality laboratory of Invemar, where they were cleaned with 10 % HCl to obtain the weight of the biomass of the plant and minimize the error due to other associated components. Subsequently, each sample was placed in previously labeled paper bags and dried at 60 °C for 72 hours to obtain a constant weight of the dry biomass value (g DW).
Numerical procedures
To calculate the biomass (g DW/m2) at each station, the initial value of the weight corresponding to the samples was extrapolated to 1 m2. Additionally, after determining the data did not correspond to a normal distribution, the results were compared with a Kruskal-Wallis test and a Tukey multiple comparison test, to determine differences between the stations at the level of their aerial, underground and total biomass, using the Statgraphics Centurion XVI program.
The carbon content was determined using the methodology described by Fourqurean et al. (2012) where the calculated value corresponds to 35 % of the dry weight of the biomass. This procedure was carried out with each sampled station, to finally convert the values to Mg C/ha and thus extrapolate the value at the seagrass extension in the two sampling areas (La Guajira and San Andrés).
RESULTS
Aerial biomass in Hondita Bay 3 and 2 presented the highest data, with values of 923.2 and 1068.4 g DW/m2, followed by Cotton Cay and Carrizal, with values above 700 g DW/m2. In the underground biomass, Hondita Bay 2 and Hooker Bay stood out with values of 866.8 g DW/m2 and 1057.1 g DW/m2 respectively, followed by Cotton Cay, with 725.5 g DW/m2. Six of the stations presented total biomass that exceeds 1000 g DW/m2, while the Hondita Bay 1 and Portete Bay 1 and 2 stations registered the lowest reported values.
Table 1. Variables of aerial, underground (at 50 cm depth) and total biomass (average + standard deviation, g DW/m2) and density (shoots/m2) for each one of the stations.
Densities presented higher values in Mar Azul (400 stems/m2) and Hondita Bay 2 (384 stems/m2) and lower values in Portete Bay 1 and 2 (below 280 stems/m2). The highest values of aerial biomass correspond to La Guajira and the underground to San Andrés. In the comparison between the stations, significant differences were evidenced at the level of aerial biomass (Kruskal Wallis = 19.24, value p = 0.03), underground biomass (Kruskal Wallis = 23.53, value p = 0.008), as well as in the total (Kruskal Wallis = 20.53, p-value = 0.02).
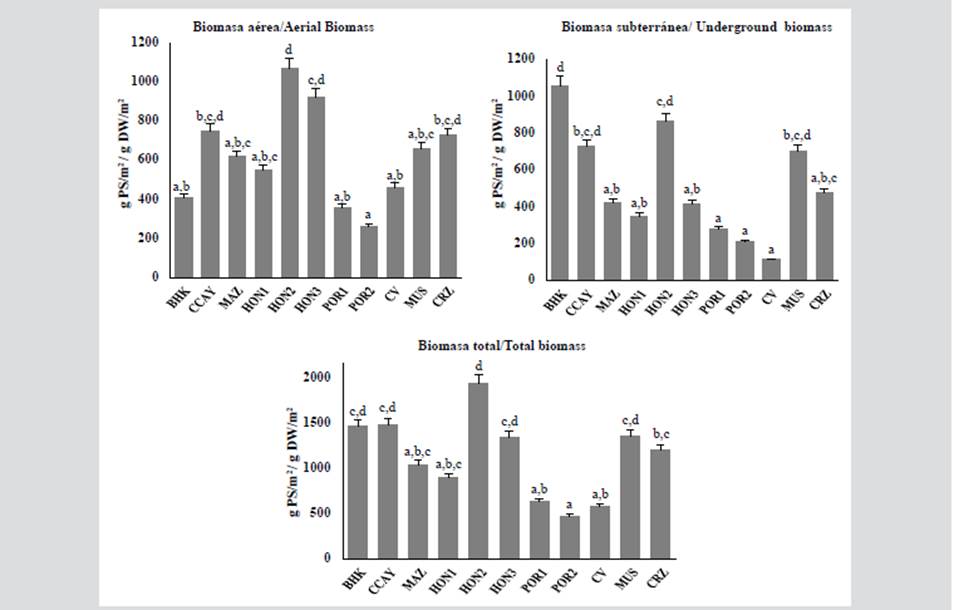
Figure 2 Values of aerial, underground and total biomass for each of the stations, POR: Portete Bay, CV: Cabo de la Vela, CRZ: Carrizal, HON: Hondita Bay, CCAY: Cotton Cay, BHK: Hooker Bay and MAZ: Mar Azul (different letters represent significant differences between stations, p < 0.05).
San Andrés presented a greater presence of carbon in its total biomass (4.6 Mg C/ha) compared to La Guajira (3.5 Mg C/ha), however, La Guajira presented a higher value at the aerial section, especially in Hondita Bay 2 (3.7 Mg C/ha), while in San Andrés the underground one stood out, especially in Hooker Bay (3.7 Mg C/ha). Stations such as Portete Bay 1, Portete Bay 2, and Cabo de la Vela, presented the lowest values in both sections (below 1.6 Mg C/ha). The stations with the highest presence of carbon in both sections combined were Cotton Cay in San Andrés with 5.2 Mg C/ha and Hondita Bay 2 for La Guajira with 6.8 Mg C/ha. Stations such as Portete Bay 1 and 2 presented the lowest values (less than 2.2 Mg C/ha).
Taking into account the average values of carbon registered in both regions and the seagrass extension of each one, it was estimated that the carbon stock associated with the biomass of seagrasses is 197484 Mg C for La Guajira and 1835.4 Mg C for San Andrés.
DISCUSSION
The differences in the biomass may be due to factors related to the conditions of each site, in the specific case of La Guajira, the resuspension of sediments is frequent in most cases (Corpoguajira and Invemar, 2012), which makes its waters extremely cloudy, a factor that stimulates the increase of the leaf area to obtain light and carry out the photosynthesis process, increasing its aerial biomass (Kraemer y Hanisak 2000; Díaz et al., 2003). This is reaffirmed by monitoring studies carried out by Invemar (Gómez-López et al., 2018), where it was observed that those with greater length correspond to La Guajira (23.3 cm), while in San Andrés is comparatively lower (18.4cm).
In San Andrés, seagrass meadows have conditions of eutrophication as a result of their proximity to the populated center (Gómez-López et al., 2012). This situation generates an increase in muddy and muddy fractions, a decrease in available oxygen, and the proliferation of opportunistic algae (Burkholder et al., 2007). In this sense, species such as Halimeda spp, Chaetomorpha spp, and Cladophora spp, are characteristic of the area (Gómez-López et al., 2012, Albis-Salas and Gavio, 2015), and can grow on top of the seagrasses, limiting in some cases, leaf growth (Duarte and Cebrián, 1996). In turn, the greater development of underground biomass in this area may be linked to the high source of sediments available in its rhizoid system, even forming terraces that can reach a height between 30 cm to 1 m above the bottom. This rhizoidal system gives them a better fixing mechanism against pressure from physical factors such as removal by boats and the presence of winds and currents due to the effect of the Northeast Winds (Albis-Salas et al., 2006).
Comparing the results with previous studies carried out in the same stations (Table 1), the values shown in this study seem to be similar, adding to them the cases of Hondita Bay 1, Hondita Bay 2, Hondita Bay 3, and Carrizal, which did not have previous records. The exception corresponds to Cotton Cay since the value reported for April 2018 varies considerably comparing the 1473.7 g PS/m2 of the present study. This could be due to the variation of the sampling season since the first case corresponds to a dry season and the second to a rainy one (Gómez-López et al, 2012). This variation has been documented in other localities of the Colombian Caribbean, where notorious changes have been registered in the structure parameters of the seagrasses between the two semesters of the year because this phenomenon generates variations in nutrients, salinity, and availability of light in the water column (Gavio et al., 2010). An example of this is documented by Gómez-López and Alonso (2016) in the Ballena and Manaure seagrass in La Guajira, as well as those of Triganá and Pinorroa in Chocó Darién.
Table 1 Variables of aerial, underground (at 50 cm depth) and total biomass (average + standard deviation, g DW/m2) and density (shoots/m2) for each one of the stations.
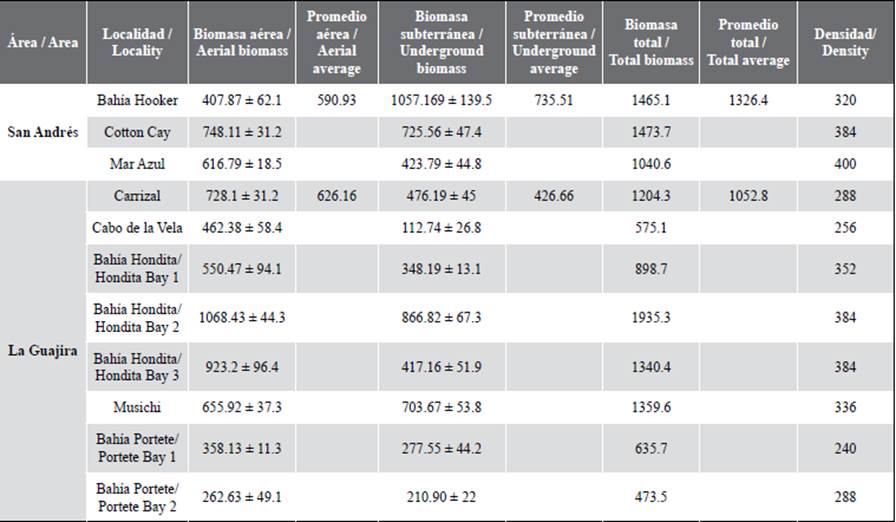
The average values of total biomass were found at an intermediate point comparing studies in other countries (Table 2). The variations between these sites could be due to physicochemical components in the water column, the type of substrate in which they develop (Lee et al., 2007; Ralph et al., 2007), and variables such as temperature and salinity, which can generate changes in the composition and structure of the meadow. In the case of this study, these variables do not seem to intervene negatively in biomass, because the average values of temperature (La Guajira: 28.6 °C; San Andrés: 29.1 °C) and salinity (La Guajira: 36.6 ups; San Andrés: 35.9 ups) (Invemar, 2020), are close to the optimal ranges reported for T. testudinum, of approximately 30 °C for temperature, and between 24-35 for salinity (Mazzotti et al., 2007). Areas with lower biomass may be the result of high temperatures and euri or stenohaline waters, causing deleterious effects due to the proliferation of phytoplankton and macroalgae, or to the natural dynamics of the meadow in its annual cycle (Burkholder et al., 2007).
Regarding the carbon present in the biomass of the two study areas, it should be noted that there are few studies focused on determining this data, especially in the Caribbean region, however, the values recorded in this study exceed the world average of 2.5 + 0.4 Mg C/ha reported by Fourqurean et al. in 2012. It should be noted that this is an average of studies carried out in different regions of the world and with different species of seagrasses, so the result could vary significantly depending on the species, as in the case of Posidonia oceanica meadows in the Mediterranean that reported average values of 7.2 + 1.5 Mg C/ha (Fourqurean et al., 2012). Particularly, in the case of T. testudinum meadows, there is data present in the Mexican Caribbean with values of 14.5 Mg C/ha (Arellano-Méndez et al., 2015) and 0.39 + 0.05 Mg C/ha (Congdom et al., 2017), and for the tropical western Atlantic with the Fourqurean et al. (2012) reported values of 0.84 + 0.1 Mg C/ha. These results are contrasting with the one reported in this study, showing the variability of the behavior of this species and how the geographical distribution and the intrinsic patterns can affect the results. Also, shows how important it is to continue investigating the related theme of Blue Carbon.
The carbon values associated with biomass tend to be comparatively lower when compared to the values calculated for the sediment (Ricart et al., 2020), whose global average is 194.2 + 20.2 Mg C/ha (Fourqurean et al., 2012), but their importance does not depend only in this function, taking into account that they also play a fundamental role in the provision of some important ecosystem services, especially climate regulation. This means that the maintenance of the seagrass structure, allows it to more efficiently carry out its function as a carbon reservoir in sediments (Pergent et al., 1994; Romero et al., 1994, Mazarrasa et al., 2015).
At level of the Colombian Caribbean, this becomes more important, taking into account the data recorded in Serrano et al. (2021), which shows an approximation of the carbon values in the sediments of the country’s seagrasses, highlighting that the stocks in the sediments of La Guajira and San Andrés exceed global averages, reaching 240 Mg C/ ha. This, added to the study from Guerra et al. (2020) for San Andrés, where the value of carbon in the sediments ranged between 91 and 144 Mg C/ha, and the information reported by Congdon et al. (2017) and Gullström et al. (2018), where it was shown that the carbon content in sediments is higher in areas where coverage and biomass tend to be greater, endorses the fact that the protection of the aerial and underground biomass system has a direct impact on the reservoirs of sediments, and therefore in the optimization of carbon capture processes.
CONCLUSIONS
Significant differences were evidenced in the stations at the level of aerial, underground, and total biomass, showing the variation that exists according to the particular conditions in which the seagrass develops.
The aerial section predominated in La Guajira, presumably due to turbidity factors that stimulate its growth to optimize light capture, while in San Andrés the underground section predominated because the stations are located in areas of the muddy substrate, requiring stronger fixing mechanisms to the bottom.
Total biomass values are similar to the values reported by various authors in the same study areas, and at an intermediate point concerning other Caribbean areas such as Costa Rica, Mexico, and Florida.
Carbon values (Mg C/ha) in San Andrés and La Guajira exceed the world average data calculated with data from several species, however, they presented a variation for those reported in other areas of the Caribbean corresponding to the same species (T. testudinum).
The carbon stocks reported for San Andrés and La Guajira correspond to estimates based on the data compiled in this study, however, future studies are required to take into account the variability of coverage throughout both regions.
Although the values of carbon reservoirs associated with biomass are comparatively lower concerning sediments, the presence and maintenance of the structure in the grassland represent a primary factor in the maintenance of this reservoir.