INTRODUCTION
Microphytobenthos is a generic term that groups eukaryote microalgae, cyanobacteria, and other photosynthetic organisms that colonize the benthic substrate (MacIntyre et al., 1996; Dalu et al., 2018). This community plays an important role in water ecosystems because, together with microalgae and submerged macrophytes, they transform chemical energy into biomass and constitute the basis of benthic trophic networks (Madigan et al., 1999). In fact, the primary production of microphytobenthos represents the major source of available energy for the higher trophic levels that live on the bottom, exceeding that of the phytoplankton in many cases (Blanchard et al., 2001). In addition, it contributes to sediment stabilization through the formation of a biofilm that is used by organisms as an energy source and transport mechanism, favoring sediment depositing, erosion decrease, and microorganism protection from herbivory and the action of pathogenic bacteria (Kang et al., 2003; Stal, 2010; Launeau et al., 2018). Moreover, the study of dinoflagellates, of which just under 200 purely benthic species have been found (Hoppenrath et al., 2014), has gained interest in recent years due to its implications during harmful algal blooms (Aguilar et al., 2014).
The abundance and composition of microphytobenthos vary substantially between locations, and these community attributes are affected by environmental and biological variables at different spatial and temporal scales (Van der Grinten et al., 2004; Jesus et al., 2006). Aggregation is a spatial feature of the distribution of these organisms, where communities are distributed into patches of highly variable size and composition, forming a mosaic at higher scales (Spilmont et al., 2011). Temporarily, there are variations that may range between inter-daily and annual (Round, 1971; Pinckney and Zingmark, 1993).
In the Caribbean Sea, few studies about the composition of the marine microphytobenthos have been conducted, with scarce references to its abundance; the studies on the benthic diatoms by Navarro et al. (1989) in the island Caja de Muertos in Puerto Rico, López-Fuerte et al. (2017, 2019) and Hernández et al. (2013) in México, and Loza et al. (2011) in Cuba are highlighted. Other studies are related to benthic dinoflagellates that cause harmful algae blooms, among which those conducted by Delgado et al. (2006) in Cuba, Rodríguez et al. (2010), Quintana and Mercado-Gómez (2014), Arbeláez et al. (2017) in Colombia, and Almazán-Becerril et al. (2012) in México stand out. In Venezuela, four studies stand out regarding epiphytic dinoflagellates present in the Thalassia testudinum prairies in the country’s north-western coasts (Valerio and Díaz-Ramos; 2007, 2008) and benthic dinoflagellates in different substrates in the Cariaco Gulf (Navarro-Vargas et al., 2014) and Margarita Island (Marchán-Álvarez et al., 2017). However, the diversity of benthic microalgae and their density, as well as their spatial and temporal variations, are little known.
For these reasons, a description of the spatial and temporal variations of the composition and abundance of microphytobenthos was considered, as well as those related to the environmental variables in the coastal area between the locations Chirimena and Puerto Francés, Miranda State, Venezuela, as a contribution to the knowledge on biological diversity, considering the relevance of this community for the marine ecosystem.
MATERIALS AND METHODS
Study area
The study area is located in the central-northern continental region of Venezuela and covers the villages from Chirimena until Puerto Francés, in Miranda State (Figure 1). It is a heterogeneous area with deep and shallow coasts, influenced by streams and rivers such as the Tuy and the coastal lagoon of Tacarigua (Herrera and Bone, 2011). Physiographically, this area is in the final foothills of the Coastal Mountain Range and is characterized by the presence of many beach-forming inlets. The main winds are the northern trade winds (direction NE-SW), and the mean water temperature is 26 ºC (Solé and Vera, 1997; Crespo et al., 2020).
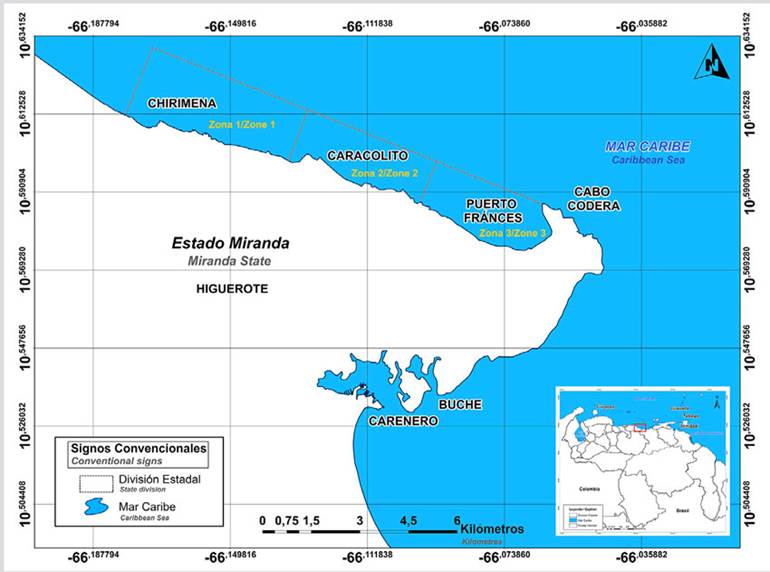
Figure 1 Location of the sampling areas in the coastal area between Chirimena and Puerto Francés, Venezuela.
This area is characterized by a tropical climate with dry summer (As), according to the Köppen climatic classification, with a mean temperature of 29 ºC and an annual rainfall of 1327 mm, with the highest precipitations in November (Crespo et al., 2020). Regarding marine currents, the area presents a different pattern due to the topographic feature of Cabo Codera, but, in general, the current pattern goes in the east-west direction (Pereira et al., 2020).
Sampling
The samples for each treatment were collected based on a two-stage and multistage cluster design with sub-samples. The study area was divided into three zones separated from each other by large areas with sandy bottoms. The first zone is Chirimena (10° 36’ 42” N and 66° 10’ 37.15” W - 10° 36’ 01.3” N and 66° 08’ 08.3” W); the second one Caracolito (10° 35’ 30.7” N and 66° 06’ 06.7” W), which borders the first zone; and Puerto Francés (10° 34’ 52” N and 66° 03’ 40.8” W) which borders Caracolito (Figure 1).
Monthly sampling was performed from June 2014 to March 2015. To randomize the sampling stations, three criteria were established. The first one was making a grid with five rows and five columns in each zone; the dimension of each cell was 50 m long towards the sea and 870 m along the coast. The second criterion was establishing three blocks of time (morning: 8:00-11:00, noon: 11:00-14:00, and afternoon: 14:00-18:00). Moreover, the third one was the bottom type with the levels: sandy and rocky. Four samples were randomly collected from each zone, and four samples in each time block were included for the whole study area.
Microphytobenthos samples were obtained using a 0.25 m2 square divided into 25 equal-size squares. Once at the sampling place, three random samples were taken using a 5 cm-diameter cylindric sampler that was buried at 1 cm in the sandy substrate, and the layer of substrate and organisms was obtained by suction. In the case of the rocky substrate, circular movements were made with the sampler to scratch the superficial layer covering the rock. It was quickly covered on both sides and was transported to the vessel to be fixed with a 5 % v/v neutralized formalin and Lugol solution.
The variables estimated were temperature, salinity (Method 2520 B, American Public Health Association 1998), pH (Method 4500-0 H, American Public Health Association, 1998), and dissolved oxygen (4500-0 G, American Public Health Association, 1998). The nutrients were: phosphates, nitrites, and nitrates (Strickland and Parsons, 1972). Rainfall data were provided by the metheorological station of Petróleos de Venezuela S.A. in Carenero.
Sample analysis in the laboratory
50 g of sediment per sample were extracted, and distilled water was added to separate microphytobenthos via ultrasound. Then, it was left for sedimentation for four hours to separate the microalgae and cyanobacteria from the sediment particles. A fraction of the sample was digested with 33 % v/v hydrogen peroxide at 90 ºC, while the other fraction did not pass through this process to avoid degradation of dinoflagellates, cyanobacteria, and other groups of microalgae, which were identified and counting in this study. The digested fraction was suspended in filtered seawater and 5 % v/v neutralized formalin. Microalgae were identified to the lowest possible taxonomic level, and their abundance was estimated from the Utermöhl method (1958) based on the analysis of 360 samples of sediment by using sedimentation chambers and a Leica® inverted microscope. Cyanobacteria were counted from their filaments, while the eucaryote forms were counted by cells, irrespective of whether they formed colonies or filaments. Analyses of chemical and physical variables were conducted following the standard methods established in the literature (Strickland and Parsons, 1972; American Public Health Association, 1998). Taxonomic localization was conducted with the specialized literature (Cupp, 1943; Peragallo and Peragallo, 1965; Ferguson, 1968; Saunders and Glenn, 1969; Sournia, 1986; Balech, 1988; Round et al., 1990; Chretiennot-Dinet et al., 1993; Moreno-Ruíz et al., 1996; Tomas, 1996, 1997; Yamaji, 1996; Berárd-Therriault et al., 1999; Witkowski et al., 2000; Siqueiros-Beltrones and Hernández-Almeida, 2006; Siqueiros-Beltrones and López-Fuerte, 2006; López-Fuerte et al., 2010; Siqueiros-Beltrones et al., 2014; Al-Yamani and Saburova, 2019), and the name of each species was verified using the algaebase.org website (Guiry and Guiry, 2021).
Statistical analysis
As part of the characterization of the community, hypothesis tests were conducted with abundance data for each species from a bifactorial model (month and zone) without interactions, applying Analysis of Similarities (ANOSIM) to prove whether the same community structure existed during the ten months of sampling, the three zones, and the two types of substrates (H0). In addition, Shannon’s diversity index was estimated, and the niche width for those species, which, together, represented 95 % of the abundance (Ignatiades, 1994). In the cases where significant differences were found with a type I error (α) = 0.05, a posteriori Least Significant Difference (LSD) tests were applied. The SIMPER test was applied with the per-species abundance data to determine the similarity percentage between sample groups (months and zones), as well as Analysis of Non-metric Multidimensional Scaling (NMDS) to determine sample distribution (Clarke et al., 2014). To evaluate the relations between the biological (species abundance) and abiotic variables determined in this study, the Bioenv multivariate analysis (Clarke et al., 2014) was used. The statistical analyses were carried out in the PAST program version 4.03 (Hammer et al., 2001).
RESULTS
Environmental characteristics of the study area
During the sampling period, a mean temperature of 26.3 ± 1.5 °C was obtained, with a minimum of 24 °C in July and January, and a maximum of 28 °C in September and November. The dissolved oxygen reported a mean of 6.56 ± 0.59 mg/L, with notable spatial differences between June and October. Salinity showed a mean of 37.64 ± 2.01, with higher values between June and August (39.3 ± 0.6), while the lowest was obtained in November, with a mean of 34.2 ± 3. The pH showed a mean of 8.1 ± 0.1, with a similar behavior in the three sampling areas (Table 1).
Table 1 Environmental variables measured in the marine zone between Chirimena and Puerto Francés between June 2014 and March 2015.
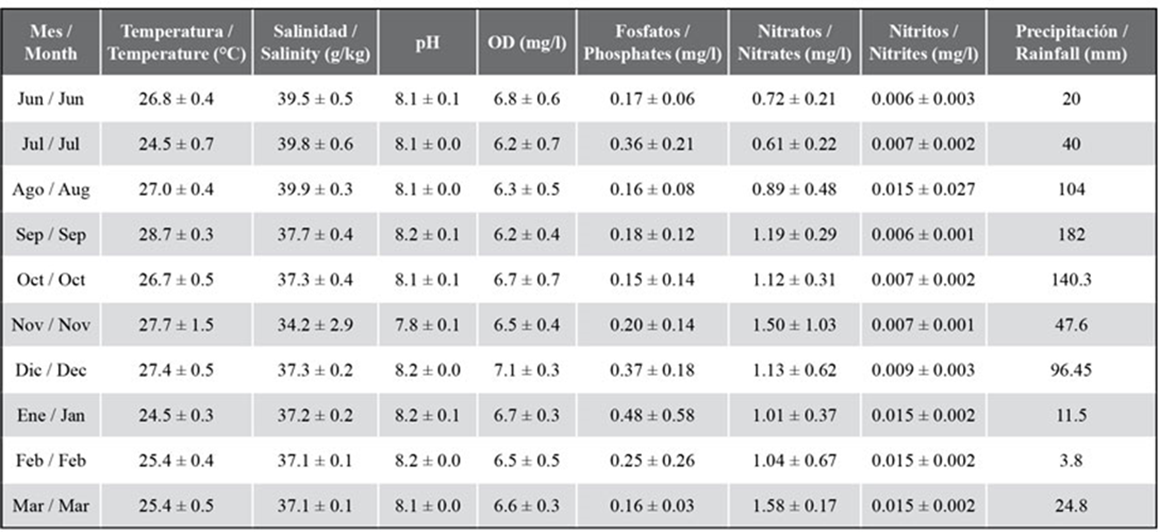
The mean phosphate concentration was 0.25 ± 0.19 mg/L, with similar temporal variations in the three sampling zones. The lowest concentrations were detected in June, August, September, and March, with 0.18 mg/L, and the highest in July and December (0.42 mg/L). The mean concentration of nitrites was 0.009 ± 0.004 mg/L, with non-significant spatial differences. Constant concentrations were temporarily obtained between June and December (0.007 ± 0.001 mg/L), and then the concentration was duplicated (0.015 ± 0.002 mg/L) and stayed constant until March. The mean concentration of nitrates was 1.08 ± 0.49 mg/L with maxima in August, November, and February (1.53 ± 0.18 mg/L). As for rainfall, the lowest values were obtained in June, July, and from January to March, with monthly total lower than 60 mm, and the highest in August, September, October, and December, reaching 182 mm in September (Table 1).
Taxonomic composition and abundance of microphytobenthos
The microphytobenthos comprised 327 species distributed into 241 diatoms (74 % of the identified species), 64 dinoflagellates, 11 cyanobacteria, 7 euglenophyta, 3 chlorophyta, and 1 ochrophyta (Table 1, Figure 2). A third part of the species, belonging to the diatom group, was present during the whole study period, with some of them being the most important in terms of abundance: Nitzschia sp.1, Navicula pavillardi, Amphora angustissima, Thalassiosira aestivalis, and various species of the genus Cocconeis. The most frequent dinoflagellate species were: Prorocentrum norrisianum, Ostreopsis siamensis, Gyrodinium estuariale, and Dinophysis acuminata, the cyanobacteria: Merismopedia sp. and Trichodesmium thiebautii, and the Euglenophyta: Eutreptiella gymnastica, Notosolenus ostium, and Petalomonas sp. It is important to highlight the presence of species generating harmful algae blooms such as Dinophysis spp., Coolia monotis, Eutreptiella sp., and Ostreopsis spp. Their abundance orders of magnitude are shown in Appendix 1.
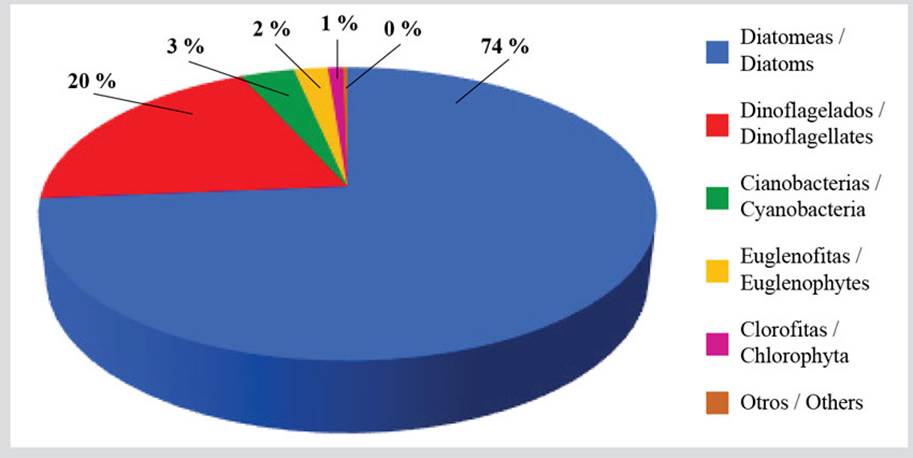
Figure 2 Percentage richness of the benthic microalgae species in the coastal zone between Chirimena and Puerto Francés, Venezuela.
In general, a monthly variation on richness in two blocks was evidenced. The first one showed a slightly higher richness from June, with 203 species, until October, with 216 species, and started to decrease, with richness remaining constant, with a mean of 196 species between January and March (Figure 3). Usually, this variation is due to fluctuations in the diatom and dinoflagellate groups because from October and November, where an important decrease of the salinity is evidenced, there was a change in the composition of species, as seen in Appendix 1. The other groups showed scarce richness variations (Figure 3). The niche amplitude index for each species (Appendix 2), which, in this case, is in the interval between 1 and 120 as a function of the number of stations and sampling months, indicates the presence of 25 species out of the 104 included in the analysis which shows a narrow niche amplitude (< 20), while only 15, where more abundant diatoms stand out, show a greater niche amplitude (< 60).
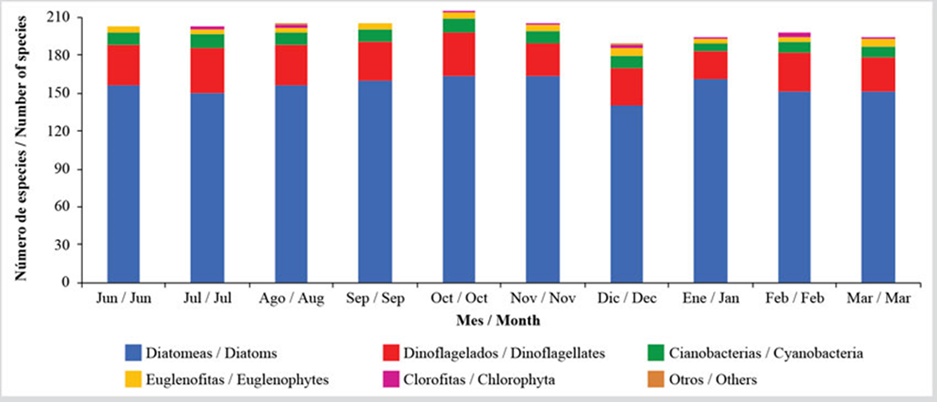
Figure 3 Temporal variation of the benthic microalgae richness in the coastal zone between Chirimena and Puerto Francés between June 2014 and March 2015.
Regarding the established zones, zone 1 showed a mean richness of 240 species without considerable differences between the rocky and sandy substrates; zone 2 showed the highest one with major variation between both substrates, obtaining 252 species in the sandy substrate and 237 in the rocky one; and zone 3 showed a slightly lower richness with a mean of 232 species. Concerning the abundance, the highest values were obtained in July and August (at the beginning of the rainy season) with maxima close to 10 × 1010 cells/m3. As of September, a mean density of 24.53 ± 3.84 × 109 cells/m3 was obtained, which indicates a stability in the abundance of microphytobenthos, even in periods of greater sediment instability, when the recorded abundances varied more with the substrate type than with the zone.
While performing the group analysis, it was observed that the relative abundance varied by a greater magnitude in diatoms and cyanobacteria during the evaluated months. This is due to the increased density of cyanobacteria in July and August, when averages of 27.56 × 109 cells/m3 and 22.23 × 109 cells/m3 were reached, which represent important increases regarding to the other months, showing a mean of 4.85 ± 2.18 × 109 cells/m3. In the case of diatoms, which represented the most abundant microphytobenthos group, with 79 %, a mean of 21.42 ± 10.18 × 109 cells/m3 was obtained, with constant values in most months, except August, when a mean density of 49.33 × 109 cells/m3 was reached. The other groups showed few variations, with mean abundances of 7.33 ± 2.93 × 108 cells/m3 in the case of dinoflagellates, 2.31 ± 1.22 × 108 cells/m3 of Euglenophyta, and 4.81 ± 4.33 × 107 cells/m3 of Chlorophytes (Figure 4).
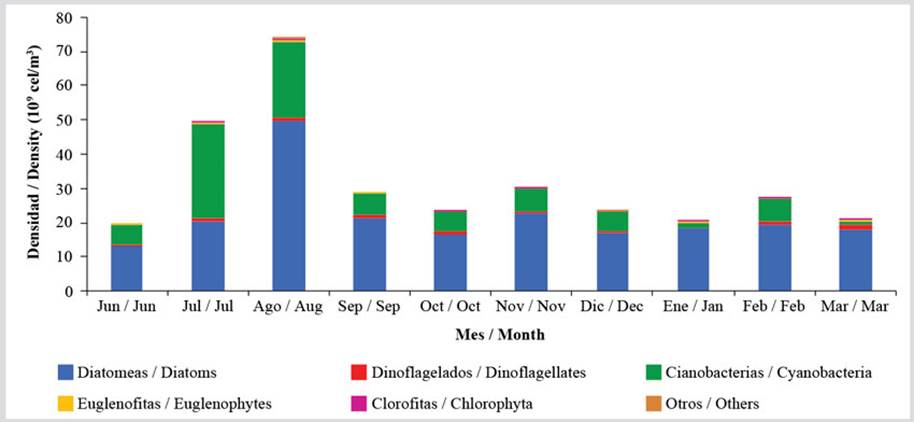
Figure 4 Temporal variation of the means richness of the benthic microalgae groups in the coastal zone between Chirimena and Puerto Francés between June 2014 and March 2015.
On the other hand, the biological diversity index showed values higher than 2.5 bits/cell, being lower in July and August, with values close to 2.8 bits/cell, and higher between September and March, with a mean of 3.3 bits/cell. In the Analysis of Similarities (ANOSIM), no significant differences were found between zones (R = 0.13; P= 0.006), but significant differences were found between months (R = 0.677; P= 0.00001), out of which only June, July, and March were different from the others. The SIMPER test indicated that samples showed similitudes between 38.7 % in June and 51.1 % in March, with fluctuations between months. When comparing the two months, June and July showed a mean similarity with the other months of 35.9 and 37.3 %, respectively, and, between the other months, slightly higher similarities were observed, fluctuating between 40 and 47 %. The species that contributed to these variations in the community were Cyanobacteria Merismopedia and Trichodesmium thiebautii, and diatoms Nitzschia sp1., Cocconeis sp., Amphora angustissima, Opephora pacifica, Thalassiothrix longissimi, as well as various species of the genus Navicula.
Regarding community differentiation as a function of the type of substrate, ANOSIM showed significant differences (R = 0.263; P= 0.00001), evidenced in the Analysis of Non-metric Multidimensional Scaling (nMDS), where a clear differentiation between both groups could be observed, suggesting that the community could vary as a function of the type of substrate (Figure 5). The SIMPER test indicates a 44.88 % similarity between the samples of the sandy substrate, where species such as Nitzschia sp1., Amphora angustata, Merismopedia spp., Cocconeis sp., Navicula pavillardii, Carinasigma recta, Thalassiosira aestivalis, Nitzschia frustulum, Stauroneis acuta, and Cocconeis sublittoralis contributed with 70 %. In the case of the rocky substrate, the similarity between the samples was 43 %. Here, besides the aforementioned species for the sandy substrate, Paralia sulcata, Thalassiothrix longissima, Navicula arenicola, Nostoc spp., Fragilaria capucina, and Trichodesmium thiebautii made their contributions.
DISCUSSION
Diatom richness was higher than that estimated in Caja de Muertos Island in Puerto Rico (Navarro et al., 1989), the Mexican Caribbean (López-Fuerte et al., 2019), the Gulf of Mexico (López-Fuerte et al., 2017), and Baja California Sur (Siqueiros-Beltrones et al., 2014). However, it is similar to that obtained by Siqueiros-Beltrones et al. (2017) in waters of the Mexican Pacific. Likewise, a dominance of the genera Amphora, Diplonesis, Cocconeis, Nitzschia, and Navicula was found, both in terms of richness and abundance, which seems to be general in the microphytobenthic community (Navarro et al., 1989; Facca et al., 2002; Patil and Anil, 2015; Al-Harbi, 2017; López-Fuerte et al., 2017, 2019; Siqueiros-Beltrones et al., 2017).
Regarding the dinoflagellates, the studies related to phanerogam prairies show a richness of 20 species in the Colombian and Venezuelan Caribbean (Valerio and Díaz-Ramos, 2007, 2008; Rodríguez et al., 2010; Quintana and Mercado-Gómez, 2014; Arbeláez et al., 2017) where only the species Cabra aremorica, Coolia malayensis, Gambierdiscus caribaeus, Prorocentrum belizeanum, P. concavum, P. emarginatum, and Sinophysis microcephala were not found. Regarding other substrates, the richness was higher than that found in a reef in the Mexican Caribbean (Almazan-Becerril et al., 2012) and in various substrates in Venezuela’s eastern zone (Navarro-Vargas et al., 2014; Marchan-Álvarez et al., 2017), but close to that obtained in a sandy beach in France (Gómez and Artigas, 2014). However, the species of benthic dinoflagellates identified in other works in the Venezuelan coasts were found in this study (Valerio and Díaz-Ramos, 2007, 2008; Navarro-Vargas et al., 2014; Marchan-Álvarez et al., 2017).
In general terms, the number of species identified in this research indicates high complexity in the community structure, where some functional groups are represented by many species. This facilitates energy transfers through trophic pathways. Consequently, there are more complex interactions that tend to increase the stability of the trophic network and therefore the capacity for resilience (Sokolowski et al., 2012). In fact, it is known that the existence of complex competitive networks in microphytobenthos with the heterogeneity of habitat and microniches could explain the diversity of this community (de Jong and Admiraal, 1984).
Regarding abundance, termporal variability remains homogeneous, with maximum values between July and August due to increases in cyanobacteria and diatoms respectively, reaching a maximun density within the range of 105 cells/cm3. These values are in the lower limit indicated by MacIntyre et al. (1996) and Schmidt et al. (1998), who considered mean abundances between 105cells/cm3 and 107cells/cm3 in the first 10 mm of sediment, reaching 109cells/cm3 in some cases, based on studies conducted in the USA, Norway, the Netherlands, Spain, and Denmark. However, Round (1971) distinguishes densities between 103 and 104 cells/cm3, and Cibic et al. (2007) quantified abundances between 104 and 105 cells/cm3 in the Trieste Gulf, in the north-east of the Adriatic Sea, which are like those found in this study.
In comparison with other studies, densities were obtained which were 100 times lower than those found in Venice Lagoon in Italy (Facca et al., 2002) and in a saline wetland dominated by cyanobacteria in Rio de Janeiro, Brazil (Masuda and Enrich-Prast, 2016). In the case of the Caribbean Sea, comparisons cannot be made with other studies, since the few that calculated density associated it with macroalgae or marine phanerogam biomass, as they provide heterogeneity of space for the settlement and colonization of Epiphytes, growing the probability of increased microalgae richness and abundance. However, said density can be compared to that of dinoflagellates obtained by Marchan-Álvarez et al. (2017), which was 20 times lower.
It is important to consider that this sampling includes nuclei of 1 cm depth, which could substantially affect the estimations, considering that the photic zone of sediments is around 2 mm. Although, in sandy sediments, microalgae can reach 16 cm of depth, but with an important decrease in density from the first 5 mm (Joint, 1978).
Regarding the relationship of this community with environmental conditions, the global and regional climatic trends include changes in temperature, sea level, tidal range, rainfall, river inputs, turbidity, and storm frequency and intensity, which are factors with a potential effect on biomass and long-term microphytobenthic primary production (van der Wal et al., 2010). Despite this, many studies evidence the importance of local factors, suggesting that the taxonomic composition of microphytobenthos is the result of a complex interaction of factors both biologic and environmental; they include light, salinity, nutrients, herbivory, and sediment type (Van der Grinten et al., 2004; Jesus et al., 2006).
In Venezuelan central coast, the temperature is mostly stable, and nutrients are available throughout the year, so the sediment instability during a period of emergence and the decrease in light penetration due to the rivers’ sediment load in the rainy season could be the most important factors in the distribution and abundance of these organisms. It has been proven that, in coral reefs, the temperature can determine up to 70 % of the microphytobenthic population variability (Blackford, 2002). Genera Nitzschia, Gyrosigma, Navicula, and Amphora, which are widely distributed in the coastal zones worldwide, including this zone, are associated with high temperatures (Cibic et al., 2009). It has also been observed that, while the abundance of most diatom genera increases with temperature, the genera Pinnularia and Diploneis prefer lower temperatures (Cibic et al., 2007). However, in tropical environments where temperatures have variations lower than 5 ºC, it is difficult to observe this type of relationship between the species and specific environmental variables, which could explain the scarce relationship obtained between the composition and distribution of the community with the estimated environmental variables.
Regarding salinity, its effect will depend on the adjustment capacity of each species, which varies even between same-genus species (Cibic et al., 2012). Different taxonomic studies have shown compositions of very diverse species with temporal variation in their domain, which indicates an intense competition in these communities, which favors some species (Admiraal, 1984). In the case of tropical regions, the relationships of microphytobenthic components with environmental variables have been scarcely studied, so more research is required. In a study conducted in the intertidal zone of a Korean estuary, it was observed that genera Amphora and Navicula were more abundant when the salinity decreased, which coincides with this study’s findings (Du et al., 2009).
With regard to the sediment type, the components of the microphytobenthic community vary considerably. For instance, in sandy sediment, where there is high hydrodynamic stress, microalgae stick firmly to the sediment particles and resist the abrasion, with the episammic monorhaphid diatoms such as Cocconeis spp. and Achnanthes spp. being dominant, as well as cyanobacteria of the genus Merismopedia (Paterson and Hagerthey, 2001). Likewise, the rocky substrates show a high diversity due to the complexity provided by the basal structure and the organisms colonizing this substrate, although more research is required on the subject (Fuji et al., 1991; Maggi et al., 2017). However, Siqueiros-Beltrones et al. (2014) found significant differences in the community structure of these substrates, which were not evidenced in this study.
Finally, new reports of microalgae species for Venezuela are indicated in this work, which contribute to the knowledge of the region’s biological diversity. Although they show few seasonal variations in density, they showed a significant replacement of species when rainfall began, whereby environmental factors such as light, concentration of suspended matter, salinity, and sediment stability could influence this community. However, a complex interaction between variables influencing the microphytobenthos community structure and the other primary producers was evidenced, which must be carefully studied.