INTRODUCTION
The term unmanned aerial vehicle (UAV) became common in the 1990s for describing autonomous robotic aircraft (Hoffmann et al., 2004; Rao et al., 2016). In recent years, UAV geographic applications have increased, acquiring data mainly with cameras (Zhang, 2008). Aerial photography allows the extraction of multiple pieces of information. For example, with a captured image, surface objects can be identified using a combination of texture, shape and tonality properties. Aerial photography with UAVs allows the identification of land cover that is not easily distinguishable in satellite imagery because of its spatial resolution. UAV mapping applications include topographic modeling, the inventory of fauna and forest, and land cover estimates (Greenwood, 2016; Fuentes et al., 2018; Fuentes, 2020).
UAVs have been used to capture images of coastal environments (Green et al., 2019) and, together with geographic information systems (GISs) and photogrammetry, also to classify and map land cover (types of sediment, vegetation and water bodies) and study their temporal changes (Gonçalves and Henriques, 2015). UAV-based methods have also been used to map coastline changes (Nikolakopoulos et al., 2019), evaluate the relationship between the activity of macrofauna excavation and the geomorphology of marshes (Brunier et al., 2020), monitor biomass in coastal saline flats (Doughty and Cavanaugh, 2019), classify coastal land cover (Meng et al., 2017), and to estimate mangrove above-ground biomass (Navarro et al., 2020). With UAVs, it has been possible to conduct studies of large areas in short periods of time and at low cost (Koh and Wich, 2012). UAVs can capture images with high spatial and temporal resolution through more frequent low-altitude flights (Mandujano and Rísquez, 2017). In the case of the marine-coastal environments of Colombia, there are currently national official maps at scales of 1:100,000 (Ideam et al., 2017) with a limited amount of detail for applications at smaller scales. In Colombia, these methods have recently been used to map rocky outcrops and mangrove forests (Castellanos et al., 2019), and coral reefs (Sierra-Escrigas et al., 2020). In addition, UAVs have been used to update the national topographic maps from the National Geographic Institute (Igac) in places where traditional methods are limited by climatic and geographic conditions (Vergara and Arenas, 2017).
Marine and coastal environments constitute approximately half of the national territory (Andrade, 2011), comprising multiple ecosystems and species of flora and fauna. Many of these environments provide ecosystem services associated with species and spaces under conservation management (mangroves, beaches, cliffs, soft seabeds, corals, seagrasses) and provisioning and support services of many local communities, particularly on the Pacific coast. The coast of the Colombian Pacific Ocean is one of the regions with the highest annual precipitation in the world (Rangel, 2004), surpassed only by Mawsynram and Cherrapunji in India, because the Pacific region experiences consistent cloud cover due to the displacement of masses of air from the Pacific Ocean and the geographical location of the Serranía del Baudo and the Eastern Cordillera of the Andes (Gutiérrez et al., 2019), which limits access to low-cloud cover satellite images.
Mudflats are nearly flat coastal areas in intertidal zones that are considered transitions and links between the sea and land. They consist of unconsolidated sediments, commonly sands and silts, with precipitated salts resulting from the deposition of estuarine silts, clays and marine animal detritus (Eisma, 1998; Tan et al., 2020). Mudflats are characterized as wetlands and periodically submerged by seawater (Bayliss et al., 1979). From the geological and geomorphological aspects, mudflats can be seen as exposed layers of mud from an environmental and ecological perspective, marshes host a large number of ecological niches and provide valuable habitats for many species, including microorganisms, crustaceans, worms, fish and birds (Phang and Friess, 2015). Mudflats or intertidal flats are a common environment along the Pacific coastlines, although rarely studied in Colombia. Mudflats are found at the mouths of rivers and in estuarine areas formed by sandy, clayey and silty sediments contributed by erosion and coastal accretion. River transport arranges these sediments into exposed or submerged mud masses according to tidal level and the influence of wave action (Gao, 2019). Mudflats include areas without vegetation, such as saline beaches, estuaries and sandy lowlands, areas with herbaceous vegetation, areas flooded with salt water and areas of fresh and brackish water flooded by the tide (Igac et al., 2007).
Mudflats represent areas with high biodiversity, including different species of benthic macroinvertebrates (Xue et al., 2019) and migratory birds that use them for food and resting stopovers (Burger et al., 1997). Particularly in Colombia, mudflats are the destination for the greatest number of shorebird and migratory species, as well as the largest number of individuals (Johnston-González et al., 2010). One of the most important mudflats for shorebirds is the shorebird reserve Bocana del Río Iscuandé, along the Pacific Coast of Department of Nariño (Cantera et al., 2009). The objective of this research was to test the potential of UAVs techniques for high spatial resolution mapping of coastal mudflats in the Bocana del Río Iscuandé, Nariño department, Colombia, under different weather conditions.
STUDY SITE
The Iscuandé River Delta is located in the municipality of Santa Bárbara de Iscuandé (2° 28’ N, 77° 59’ W) along the Pacific Coast of the Department of Nariño. This area is included in the Iscuandé-Sanquianga-Gorgona Coastal Marine Complex, which forms part of the Guapi-Iscuandé Integrated Management Unit, in the Sanquianga National Natural Park (Figure 1). This delta lies within the geomorphological unit of mangrove swamps, flooding twice daily at each high tide; its substrate is muddy and rich in organic matter, so it hosts mangrove forests and other organisms associated with this ecosystem, presenting an average tidal range of 3.7 m (Invemar et al., 2003). Its relief is determined by the alluvial shelf of the Pacific Ocean and is characterized by lowlands and floodplains adjacent to the sea, traversed by estuaries. Located in the neotropical Chocó Biogeographic region, the Bocana de Iscuandé receives an average annual rainfall of 3,318 to 5,909 mm yr−1 (Rangel, 2004). Among its landscapes and ecosystems are mangrove forests, floodplain forests, beaches, barrier islands and mudflats, with diverse communities of fish, mollusks, crustaceans, bivalves and shorebirds (Calidris et al., 2017). There are two mudflats along the shores of the Iscuandé coastline known locally as Bajo La Cunita (2°39’15” N, 78°03’00” W) and Bajo Quiñones (2°39’57” N, 78°00’28” W) (Figure 1). These mudflats are recognized as sites of biological importance at the national level and have been declared as a Regional important site within the Western Hemisphere Shorebird Reserve Network (WHSRN) in 2009 due to their significant populations of migratory birds (Calidris, 2017).
MATERIALS AND METHODS
During the months of August and November of 2019, the mudflats of La Cunita and Quiñones were visited between 9 and 10 am at low tide to obtain images of exposed mudflats and with optimal solar light conditions. The images were captured with two conventional UAVs. For the first flight in Quiñones, the DJI Phantom 3 UAV was used, with a 1/2.3” CMOS RGB sensor (12 effective MP) with a maximum resolution of 4,000 x 3,000 pixels. For the second flight in Quiñones and the two flights in La Cunita, a DJI Mavic Air vehicle was used, equipped with a 1/2.3” CMOS RGB sensor (12 MP effective) with a maximum resolution of 4056 x 3040 pixels. The images were georeferenced using a global navigation satellite system (GNSS) that includes vehicles in both the Global Positioning System (GPS, USA) and Global Navigation Satellite System (GLONASS, Russia), with horizontal and vertical accuracies of approximately ± 1.5 m and ± 0.5 m, respectively.
The flights were prepared with the online platform DroneDeploy, in which the area to be photographed was chosen using Google Maps and flight variables such as flight height and overlap were defined, that is, the percentage of the common area between photographs. The value of the height has an inverse relationship with the number of photos taken during the flight; that is, the greater the height, the fewer photos were taken, and vice versa. A large overlap between images is required to obtain high-precision results. Therefore, the image acquisition plan was carefully designed to have sufficient overlap as a function of the flight time. Taking into account atmospheric variables such as wind speed and cloudiness, a height of 90 m was established for the La Cunita flights and 150 m for Quiñones, with an overlap percentage of 65 % for both flights. A ground sampling distance (GSD) was set for all flights to be less than 15 cm pixel size, considering that the type of terrain to be mapped was a mainly flat intertidal zone. The same flight missions were executed in each area because DroneDeploy saves previous missions for use in the future.
Subsequently, images were processed in the UAV flight-specific photogrammetric software Pix4Dmapper version 4.2.27. The software automatically finds thousands of common points between images. Each characteristic point found in an image is called a keypoint. When keypoints coincide in two or more images, they are considered coincident and are used to generate a three-dimensional point. The more keypoints there are, the more accurately they can be calculated from a group of photographs taken from a UAV. From these photos, a point cloud is created as a function of the overlap percentage, which facilitates calculation of the parallax and subsequent three-dimensional reconstruction of the shapes present in the images, thus generating an orthophotograph of the overflight area. Prior to this step, the ground control points (GCP) of the data taken with the GNSS are added to improve the positioning of the images. As a result, four orthophotos were generated and the Universal Transverse Mercator output coordinate system (UTM 17 North) was chosen for the study area. The orthophotos resulting from the images from the DJI Phantom 3 vehicle were scaled to 14.93 cm/pixel, while the DJI Mavic Air drone images were scaled to values ranging between 4.32 and 5.39 cm/pixel (Table 1). In total, more than 1510 photographs were taken in the four flights over the two mudflats.
Orthophotos were uploaded to ESRI® ArcGIS version 10.5 and used to create a land cover layer with the following classes: sand spit, mangrove, grass, pond, shrubland (majagua) and tidal mudflats. The final mapping scale was of 1:5000 for Bajo La Cunita and 1:2500 for Bajo Quiñones. The difference in scale was related to the use of two vehicles with different maximum resolution sensors. Figure 2 summarizes the above methods.
After the orthophotos were produced (Figure 3), the final photointerpretation polygons were generated to construct the thematic cartography of the two zones.
RESULTS
La Cunita tidal flat, the largest in the territory, has an extensive area of muddy sediments at its eastern margin in which a network of surface channels is formed that drains in the west-east direction as a function of the slope (Figure 4). A sandbar borders the mass of muddy sediments on the west because this area is not flooded during high tide and protects the mudflat from wave action. There is a strip of majagua (Talipariti tiliaceum) over the sandbar, and some individuals of red mangrove (Rhizophora mangle) over the mudflat (Figure 5).
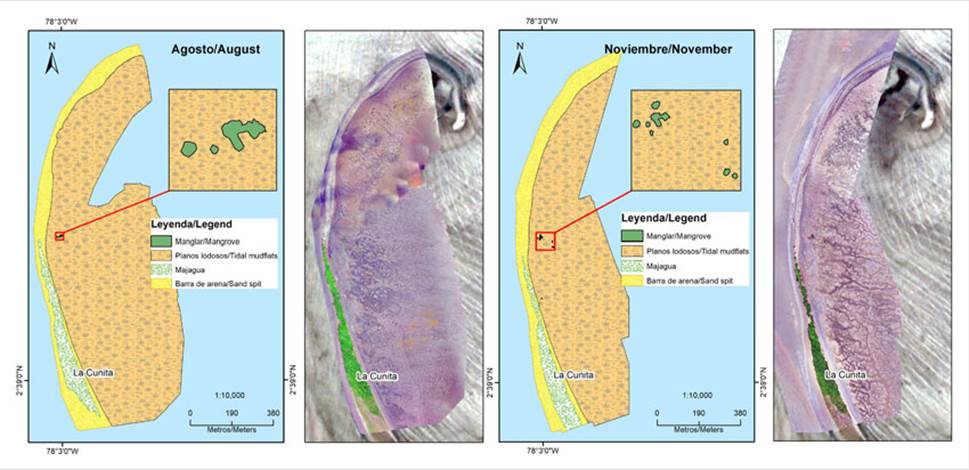
Figure 4 Map of Bajo La Cunita for August (2 left panels) and November (2 right panels) of 2019. The background image is a PlanetScope image from August 2019.
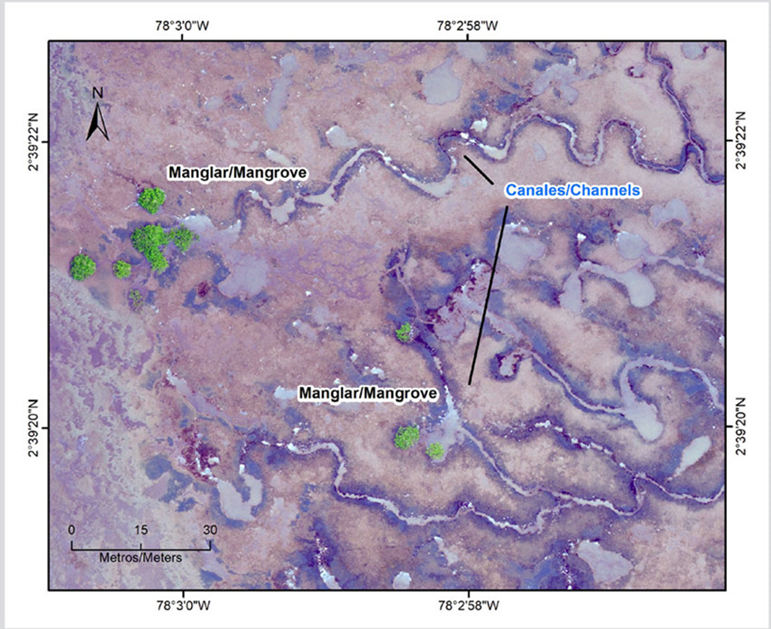
Figure 5 Channel system in the muddy zone and red mangrove individuals in Bajo La Cunita. The purple color shows an accumulation of decomposing organic matter and sediments.
There were several changes in the extension of coastal covers from August to November, including the accretion of the sand bar at the northern end, the decrease in size of the majagua shrubland strip, and the increase in the number of red mangroves (Table 2). Although the area of exposed muddy sediments apparently decreased, this effect is more likely due to sampling. Differences in tidal height and the difficulty of compositing image mosaics in areas close to the water make it difficult to obtain a perfect overlap, as well as to determine changes in the area of mudflats.
Table 2 Changes in land cover extent (ha) at La Cunita.

* Apparent decrease, see text for explanation
As the largest mudflat of the estuarine area, La Cunita houses the largest number of shorebirds by number of species and individuals, such as specimens of the family Scolopacidae along with species of the family Charadriidae. Birds seek food in the muddy substrate and rest and nest in the vegetated sand bars to reproduce.
Bajo Quiñones has a smaller total surface area and muddy area than La Cunita (Figure 6). The vegetation is dominated by a group of red mangroves (Rhizophora mangle) taller and more mature than those at La Cunita. Halophilous grasses are found next to the mangroves to the west and at the center of this area. To the north, there is a small seawater pond inhabited by crustaceans such as the blue crab (Cardissoma crasum) surrounded by mangroves. A sandbar traverses the mudflat from north to south, sloping eastward, similar to that one at La Cunita, but with a shorter length.
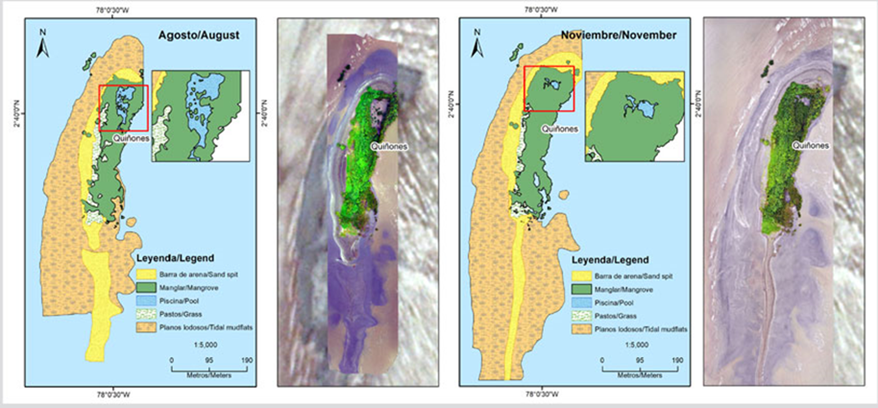
Figure 6 Map of Bajo Quiñones from August (2 left panels) and November (2 right panels) of 2019. The background image is a PlanetScope image from August 2019.
From August to November, the extent of exposed intertidal mudflats increases (note that the mosaic borders do not match entirely because the tidal height modifies the resulting image). Additionally, the width of the sand bar decreases, particularly at its southern tip (Table 3).
During this period, the mangrove area increases at the expense of grass and water (pond). In addition, there is sediment redistribution along the beach (Figure 7).
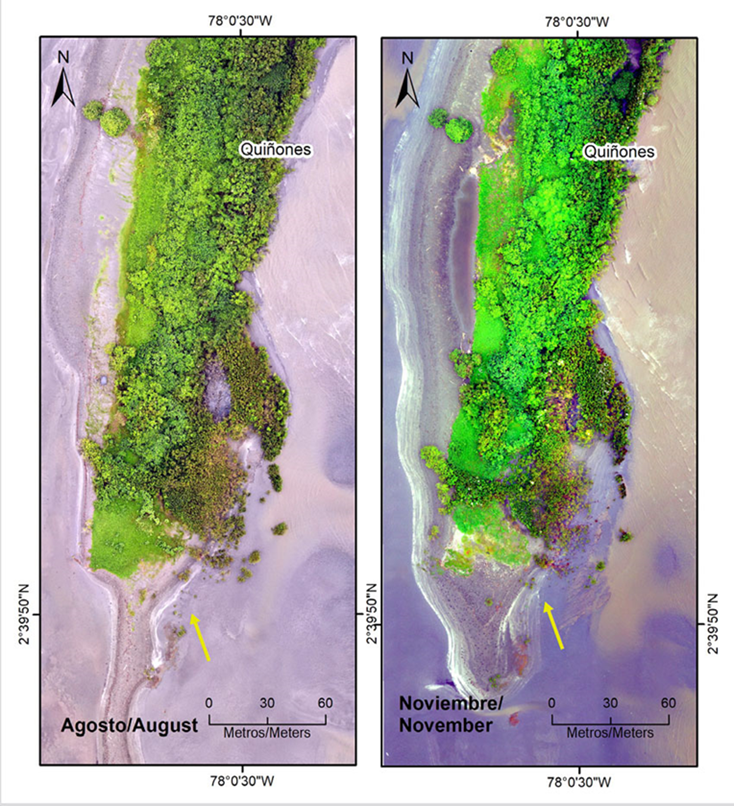
Figure 7 Changes in vegetation and sediment redistribution (yellow arrows) in Bajo Quiñones between August and November 2019.
This mudflat houses a smaller number of bird individuals due to the limited space available; however, larger birds such as the brown pelican (Pelecanus occidentalis) are present as they use large mangrove trees for resting.
DISCUSSION AND CONCLUSIONS
The use of UAVs for mudflat mapping allowed the detailed survey of environments that had not been mapped before due to their limited access and cloud cover conditions. It should be noted that the relatively small size of the study area (< 79 ha) allows the use of this technique, which would not be feasible for larger scale national surveys such as the official Continental and Coastal Ecosystems Map. Additionally, UAV mapping is useful to assess spatial changes over a short period of time, as shown by studies in other coastal areas (Samiappan et al., 2017a, 2017b; Gonçalves et al., 2018; Broussard et al., 2020; Gómez-Gutiérrez and Gonçalves, 2020) and in the present study. In coastal environments, high-accuracy elevation models and the orthophotos derived from UAVs datasets improve the temporal and spatial detail of geomorphological surveys, successful implementation of a UAVs survey increased the temporal resolution of the dataset and potentially reduced the cost of deployment. Demonstrating the high spatial and temporal resolution that UAVs provide when flying at very low altitudes and at the frequency desired by the researcher (Mandujano, et al. 2017). Photogrammetry from UAVs has become an important and possibly more affordable solution for coastal monitoring according to Gonçalves and Henriquez (2015). This assertion coincides with improved classification of mudflats through photointerpretation of very high-resolution images. The results are accurate to the extent that the elements in the photograph can be observed in detail through zooming along with the notes and records made in the field to more easily identify plant species and geographic features. The process, although more time-consuming, provides more accurate results compared to the classification of satellite imagery (Schmedtmann and Campagnolo 2015; Broussard et al., 2020).
In our case, the use of UAVs allowed a detailed characterization of the spatial and temporal dynamics of the La Bocana de Iscuandé mudflats, where we found differences in their sedimentological and morphological constitution. Specifically, we found differences in the type of materials, slope and tidal and wave activity, conditions that shape the development and evolution of these landscapes (Gao, 2019) and give them a high spatial dynamism over short periods of time. Such characteristics make them an ideal site to monitor with the aid of UAVs.
Limitations of this technique for our study area include the difficulty in obtaining high-precision ground control points (GCP), different spatial resolutions related to the use of different cameras, and noise from areas with moving water. With regards to GCPs, we did not use high-precision GCPs, as is typical for this technique, due to the difficulty in accessing the muddy areas exposed to the sea. Even so, the orthophotos did not show any significant distortions due to their limited spatial extent and flat topography. Their correct positioning was corroborated with orthorectified images from the 3-m-resolution Planet satellite with similar dates to our flights. The use of UAVs with different spatial resolutions and sensors, despite not compromising the final mapping detail, yielded some differences in pixel size so it is advisable to use a single vehicle and thus obtain orthophotos with similar pixel size. Finally, images had a noise effect from sunlight reflection in areas with moving seawater, even if they were acquired when the sun was not at its zenith. Photos with this effect could not be merged by the software, resulting in some differences in the extent of the final orthophotos. For future flights that include marine areas, the use of floating elements of contrasting colors such as buoys should be considered to use as control points, thus increasing the accuracy of the orthophotos.
UAVs are a practical tool for mapping spaces whose dimensions and meteorological conditions make them inconvenient to study with satellite images or conventional aerial photography, for example, remote coastal areas. Here, we used UAV images to characterize the dynamics of two mudflats over a period of four months, using very high spatial and temporal resolutions. The increased flight frequency and more precise terrestrial control points, together with more studies about their geology, biology and environmental quality, will generate critical data for conservation planning in places of great ecological importance, particularly for migratory birds.