Serviços Personalizados
Journal
Artigo
Indicadores
-
Citado por SciELO
-
Acessos
Links relacionados
-
Citado por Google
-
Similares em SciELO
-
Similares em Google
Compartilhar
Ingeniería y Universidad
versão impressa ISSN 0123-2126
Ing. Univ. vol.19 no.1 Bogotá jan./jun. 2015
https://doi.org/10.11144/Javeriana.iyu19-1.btea
Biomass: Technical and Environmental Alternative in the Thermoelectric Generation Process1
Biomasa residual: alternativa técnica y ambiental en el proceso de generación termoeléctrica2
Álvaro Restrepo3
Edson Bazzo4
1Submitted on: February 20th, 2014. Accepted on: November 20th, 2014. This paper is derived from the research project: use of rice straw in co-firing process with pulverized coal, developed by the Combustion and Thermal Systems Engineering Laboratory - LabCET, UFSC - Florianópolis - SC - Brazil.
2Fecha de recepción: 20 de febrero de 2014. Fecha de aceptación: 20 de noviembre de 2014. Este artículo se deriva de un proyecto de investigación denominado Utilização da palha de arroz em processo cofiring com carvão pulverizado, desarrollado por el Laboratório de Combustão e Engenharia de Sistemas Térmicos LabCET - UFSC - Centro Tecnológico -Florianópolis - SC - Brasil.
3Ingeniero Mecánico de la Universidad Autónoma de Occidente, Cali, Colombia. Magíster en Ingeniería Mecánica de la Universidad de los Andes, Bogotá, Colombia. Doctor en Ingeniería Mecánica de la Universidad Federal de Santa Catarina, Florianópolis, Brasil. Profesor asociado de la Facultad de Ingeniería Mecánica de la Universidad Tecnológica de Pereira. Pereira, Colombia. Correo electrónico: arestrep@utp.edu.co.
4Ingeniero Mecánico de la Universidad Federal de Santa Catarina, Florianópolis, Brasil. Magister en Ingeniería Mecánica de la Universidad Federal de Santa Catarina, Florianópolis, Brasil. Doctor en Ingeniería Mecánica de la Universidad Federal de Santa Catarina, Florianópolis, Brasil. Profesor asociado del programa de Ingeniería Mecánica de la Universidad Federal de Santa Catarina. Correo electrónico: e.bazzo@ufsc.br.
Para citar este artículo / To cite this article
Á. Restrepo and E. Bazzo, "Biomass: technical and environmental alternative in the thermoelectric generation process", Ing. Univ., vol. 19, no. 1, pp. 67-86, Ene.-Jun., 2015. http://dx.doi.org/10.11144/Javeriana.iyu19-1.btea
Abstract
This paper presents a technical (based on the exergy amount) and environmental analysis concerning an existing 50 MWe steam power plant located in the south of the Santa Catarina state - Brazil, designed to operate with pulverized coal and modified to operate in co-firing process with coal-biomass. In addition to the power plant, the study considered an extended boundary that involves the processes related to the obtaining, transportation, and handling of both coal and biomass. The exergy analysis was focused in the second law of thermodynamics, while the environmental analysis followed the Life Cycle Assessment (LCA) methodology, taking into account 1 MWh as functional unit and the global warming impact category, following the IPCC GWP index, over 100 years. For both cases (only coal and co-firing), the exergetic analysis indicated that the power plant is responsible for over 95 % of the exergy consumption. Results indicated that for the case of operating only with coal, 1,230 kg of CO2 e per MWh are emitted, whereas for operation in co-firing, with a share of 10% of biomass on energy basis, this amount changes to 1,103 kg CO2 per MWh.
Keywords: biomass; power plants; exergy; co-firing process; Life Cycle Assessment (LCA); Greenhouse Gases (GHG)
Resumen
Este artículo presenta los resultados del análisis técnico (basado en la cantidad de exergía) y ambiental de una planta termoeléctrica de 50 MWe nominales, localizada al sur del estado de Santa Catarina (Brasil), diseñada para operar con carbón pulverizado y adaptada para operar en proceso co-firing carbón-biomasa residual. Además de la planta termoeléctrica, el estudio consideró una frontera extendida que involucró los procesos relacionados con la obtención, transporte y manipulación tanto del carbón como de la biomasa. El análisis exergético siguió lineamentos de la segunda ley de la termodinámica, mientras que el análisis ambiental se realizó siguiendo la metodología de análisis de ciclo de vida (ACV), considerando 1 MWh como unidad funcional y la categoría de impacto en el calentamiento global, mediante el método IPCC 2007 GWP 100 años. Para ambos casos (operación solo con carbón y en co-firing), el análisis exergético indicó que la planta termoeléctrica responde por más del 95 % del total de la exergía consumida. Para el análisis ambiental, los resultados indicaron que para la operación solo con carbón se emiten 1230 kg de CO2-eq por MWh, mientras que para la operación en co-firing, con una participación de hasta 10 % de biomasa en base energética, el valor efectivo de la emisión fue de 1103 kg de CO2-eq por MWh.
Palabras clave: biomasa; planta termoeléctrica; exergía; Proceso co-firing; Análisis Ciclo de Vida (ACV); Gases Efecto Invernadero (GEI)
1. Introduction
A widespread discussion about climate change is taking place nowadays. One of its most representative factors might be the inevitable use of fuels derived from oil, necessary for many human activities. According to information from the International Energy Agency (IEA), in the last 40 years, the total contribution of the main energy carriers (coal, oil, and natural gas) has more than doubled, from approximately 6.000 Mtoe to 13.000 Mtoe [1]. Despite the high ratio of CO2 emissions reported during coal combustion, the worldwide electric power generation based on this combustible amounts for nearly 40% of the total amount generated. The explanation for this high share is in its low costs and high availability in the international market. Co-firing of coal with residual biomass from traditional crops has been studied during more than a decade as a technological, energetic, and environmental alternative to direct combustion. Its purpose is to mitigate greenhouse gases (GHG) released from the direct combustion of coal, mainly CO2, released during coal combustion, and CH4, since the biomass is not decomposed in the farming fields. It is important to remark that according to the Intergovernmental Panel on Climate Change (IPCC), within a 100 years horizon, CH4 is regarded as 25 times more aggressive than CO2 regarding its Global Warming category.
Within the field of thermoelectric generation from co-firing, it is important to highlight the work by the Electric Power Research Institute (EPRI), where steam generators are studied after modifications for co-firing operations with more than 50 different sources of residual biomass [2]. Similar studies were carried out in the European Union to assess the potential of residual biomass in co-firing process, aiming to replace up to 15% of the coal energetic base in electricity power generation [3]. Other studies analyzed the co-firing process, focusing on the formation of short-lived gases such as NOX and SOX [4]. Meanwhile, a Japan-based case study [5] presents the results of the environmental evaluation of nine thermoelectric generation systems by means of Life Cycle Assessment (LCA), using a GHG factor as index to evaluate the characteristics of the GHG actually released by the different generation systems. Likewise, several researchers studied the GHG emissions during the Life Cycle of a pulverized coal thermoelectric plant in the United Kingdom, including the construction and dismantling stages [6]. In addition to these studies, there are detailed report results from LCA-based evaluation of several thermoelectric plants in the U.S.A [7]. One of the presentations in the 20th International Congress of Mechanical Engineering [8] shared the studies on the potential of residual biomass from traditional cultures and its use in co-firing operations in a thermoelectric complex in Brazil. In a similar manner, a presentation held during the 13th Brazilian Congress Thermal Sciences Engineering [9] showed the thermodynamic analysis of a steam generator modified to co-firing operation of pulverized coal and residual biomass. To complement the previous information, another study presents the results obtained after measuring the performance of biomass combustion (coffee husks and rice husks) in a bubbling fluidized bed reactor [10]. By analyzing the amounts of CO2, O2, and CO obtained from its emissions, the variables that affect the biomass combustion process are defined. Recently, studies involving biomass as an energy source have been reported. An example of this would be the work of researchers on a study that shows the results of co-firing versus biomass-fired in a power plant, considering the GHG emissions savings comparison by means of the LCA (Life Cycle Assessment) methodology [11]. Another example is the analysis of the penetration of rice straw co-firing systems in the Taiwanese power market [12]. A similar study investigates the economic feasibility of rice straw co-firing at coal power plants in Malaysia considering capital, and logistic costs [13]. It also includes the implication of rice straw use under different co-fired ratios, transportation systems, and CO2 emission prices. Finally, another study in the same area delves on the impact of co-firing biomass (wood pellets and straw pellets) on the emission profile of power plants with carbon capture and storage. Two types of coal-fired power plants were assessed: a supercritical pulverized coal power plant (SCPC), and an integrated gasification combined cycle plant (IGCC).
This paper shows the potential of residual biomass as fuel in the thermoelectric generation process, based on a technical (exergy-basis) and environmental evaluation of the first experience in Brazil. Both the exergetic and the environmental analysis considered an extended boundary; also both analysis were subject to a systematic methodology. The exergetic analysis followed the guidelines of the second law of thermodynamics, whereas the environmental analysis was based on LCA, which is internationally accepted for processes analysis and supported by the European Standards ISO 14040 and 14044 [15], [16].
2. Boundary
Our analysis considered an extended boundary of four stages; the first three corresponding to the obtaining, transportation, and previous processing of the combustibles (coal and biomass), and a fourth stage corresponding to the process in the thermoelectric plant (TEPP). These stages can be seen in Figure 1.
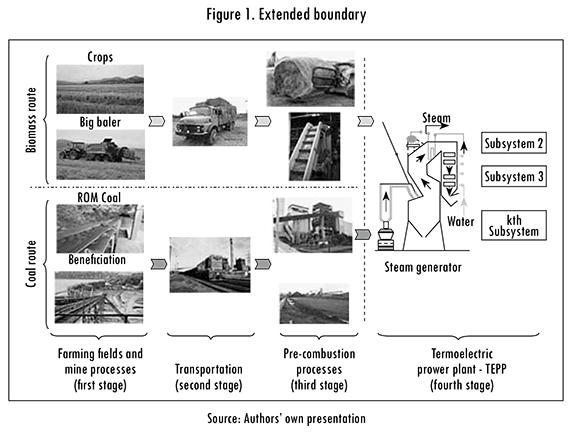
2.1. Description of the thermoelectric power plant (TEPP)
The TEPP of the study is located in the southern region of the Santa Catarina State in Brazil. Its thermodynamic cycle includes a steam generator, a turbine of high and low pressure without intermediate heating, feed-water heaters, and a deaerator. The condenser uses river water for the cooling process. Under normal operation conditions, 185 t/h of steam are generated at 89 bar and 510 °C. Table 1 shows the nominal and operational characteristics of the TEPP.
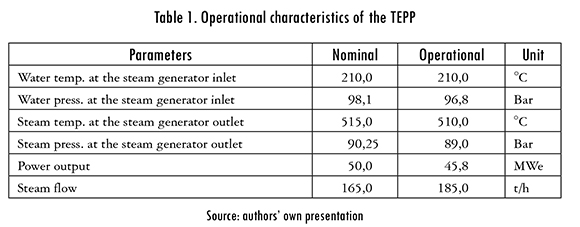
2.2. Coal route description
Coal is extracted from mines located in the southern region of Santa Catarina. According to the ASMT classification, it is considered highly volatile, bituminous, with high content of ashes and volatile matter [17]. Its extraction requires underground mining using the room and pillar technique. Run of mine (ROM) coal is taken to the surface where the beneficiation process starts in order to obtain the required granulometry. Beneficiation consists of two operations: (i) Crushing, to homogenize the size of coal in a range between 25 and 32 mm; and (ii) cleaning, to remove part of inert material and sulfur through jigging washing. The coal transportation to the TEPP is done with freight trains; there it is homogenized and transported to the storage silos, which in turn supply the coal mills.
2.3. Biomass route description
The biomass considered in this work is rice straw obtained from large crops in the neighboring area of the TEPP. Santa Catarina's Company of Farming Research and Rural Extension Service (EPAGRI by its abbreviation in Portuguese) has reported that the southern region of Santa Catarina has yearly rice crops of approximately 650,000 tons. According to the ASAE Paper by Yore, Summers, and Jenkins [18], the agricultural output of this kind of biomass depends on variables such as weather, soil quality, and the individual features of the crop, making hard to estimate the potential. However, in the literature a factor close to 0.5 has been reported, leading to a reasonable expectation of 325,000 tons of straw per year.
Due to the average distance between the rice growing fields and the TEPP, the straw was compressed in bales. The definition of technological route of the bio-mass took into account the whole process, from the growing fields to the TEPP. The transportation was performed by diesel trucks in an average distance of 60 km.
The straw rice processing system previous to combustion consists of conveyors, vibrating grills, and a vibrating sieve to remove foreign bodies added while compressing in bales in the growing fields. The pulverizing process needs to lower the moisture of the biomass, so the straw is transported through a pneumatic system with a hammer mill; the pulverized biomass is then stored in a silo, where it is subsequently transported through a pneumatic system of pipelines that intersect the pulverized coal, guaranteeing a uniform mix of the two combustibles for the co-firing operation before entering the burner.
Table 2 shows the proximate and ultimate analysis of both coal and rice straw. The properties were determined from samples evaluated in the Institute of Combustion and Power Plant Technology (IFK) Stuttgart, Germany.
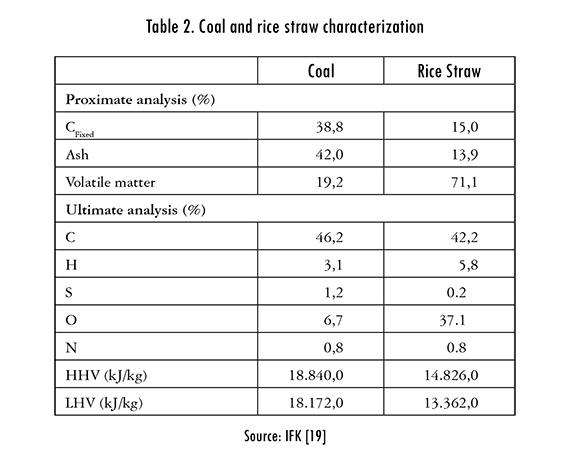
3. Methodological proposal
Figure 2 gives a systematic presentation of the methodological proposal for both the exergetic and environmental analysis of the thermoelectric generation. It comprises three steps: the first is related to the definition of the problem; the second includes the exergetic and environmental analysis; and finally the third corresponding to the results and discussion. The following are descriptions for each step of the methodological proposal for the case studied herein.
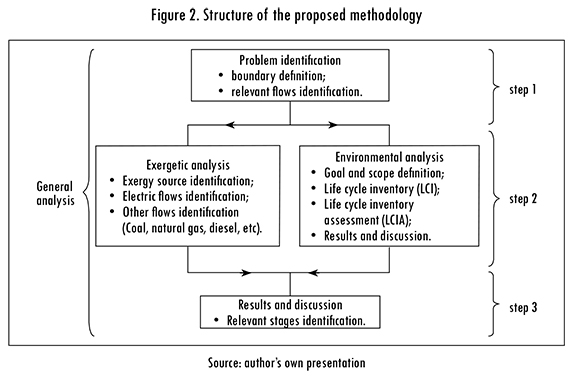
3.1 Problem identification
According to Figure 2, the first step of the proposal consists of a visual tour of the processes studied herein in order to properly identify the boundary of the problem. The boundary should include all the relevant elements to the exergetic and environmental analysis, i.e: processes, sub-processes, mass, and energy flows. Once the boundary is defined, the problem is represented in a flowchart that helps to visualize the relationship of the input flows (entering through the boundary), the identified processes, and the output flows (emissions to the air or water exiting through the boundary).
3.2 Exergetic and environmental analysis
From the thermodynamics point of view, the objective of the exergetic analysis is to identify in the stages defined by the problem boundary (step 1) processes with potential for improvement; whereas the environmental analysis has the objective of estimating the impact associated to the process under review. Both the exergetic and environmental analysis depend on the appropriate identification of mass and energy flows associated to the process, and this emphasizes the importance of the first step of this methodological proposal.
3.2.1 Exergetic analysis
For the case of the thermoelectric generation studied here, the combustible at the boiler inlet is proposed as the exergy "source" (see Figure 3). Combustible exergy is estimated as follows:
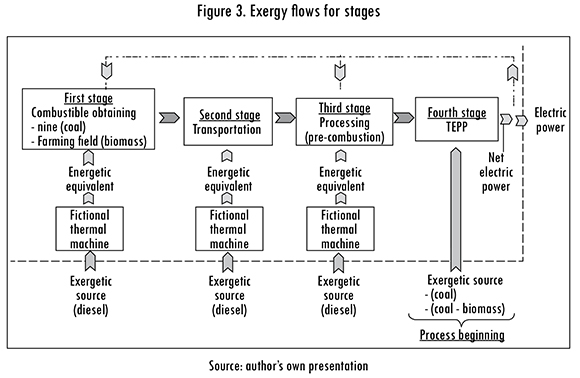

where m is the mass flow of the combustible and e is the specific chemical exergy of the combustible [20], thus:

Here, and echs and echH20 are the chemical exergy of sulfur and water, andand mf;C; mf;H; mf;O; and mf;N are the mass fraction of carbon, hydrogen, oxygen, and nitrogen respectively.
LHV is the inferior heat value and the β factor corresponds to the chemical exergy of the ratio of chemical exergy of dried organic substances. This factor is calculated for coal and rice straw as follows:
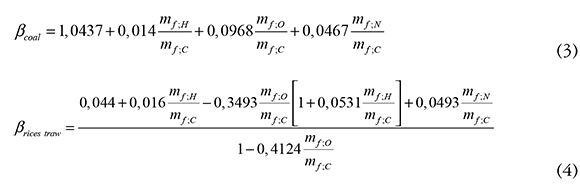
The thermodynamics cycle performance parameters considered, as expressed by Bejan, Tsatsaronis, and Moran [21] are: the efficiency of the second laws of thermodynamics ηII; the total destroyed exergy ED;total and the destruction ratio of energy γdest,k.
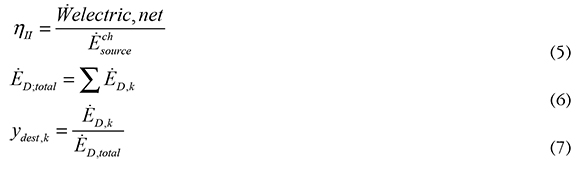
For the extended boundary, the next hypotheses were considered:
- As indicated in Figure 3, the model assumes that the electric energy consumed in the first and third stages (for both routes) is generated by the TEPP, and therefore it can be regarded as destroyed exergy.
- For operations involving diesel consumption, it is advisable to include the energy of diesel as an equivalent value to the electric energy (exergy) generated by the TEPP With that purpose, we propose a "fictional" thermal machine with efficiency equivalent to that of the TEPP. Thereby, the destroyed exergy from the diesel use is equivalent to that exergy estimated by Equation (8):

where mj is the mass flow of diesel and ηsq is the equivalent efficiency.
The assesment parameters are proposed according to the approach described in a Brazilian study on exergy-environmental analysis of thermoelectric plants [22]:
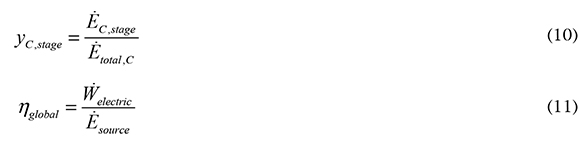
where Ètotal,c is the total exergy consumed by the system; γC,stage corresponds to the ratio of consumed exergy during the different stages; and ηglobal' is the global efficiency of the thermoelectric power generation, taking into account the total exergy consumed by the extended boundary.
3.2.2 Environmental analysis
The environmental analysis was performed using the Life Cycle Assessment (LCA). The principal challenge for the LCA is the construction of a reliable model of the characteristics of the process under review beyond simplifications and hypothesis. LCA has four steps: (i) definition of the goal and scope of the study; (ii) Life Cycle Inventory (LCI); (iii) Life Cycle Impact Assessment (LCIA), and (iv) interpretation of results.
The goal of the analysis should consider both the characteristics and the reasons behind the study, while the scope should clearly define the system. Also, the functional unit is defined and the impact categories to be evaluated will be determined in this first part. In the second part, the LCI is carried out based on the flows of mass and energy relevant to the process and they are subsequently related to the functional unit. The first step in the methodological structure should be helpful to the LCI (see Figure 2). The third part (LCIA) needs to define the most appropriate method to analyze the impact categories. The fourth part corresponds to the ACV results and discussion.
3.3 Results analysis
The purpose of the third step is to evaluate the information obtained during the previous steps of the methodological structure. From the exergetic point of view, the results should identify stages and processes relevant due to their high exergy consumption. From the environmental point of view, stages and processes with the greatest impact generation should be identified in agreement with the method and the impact category considered. In this study, this step is presented together with the results of step 2 (see item 4.2).
4. Results and discussion
The following is a step-by-step presentation of the results from the methodological proposal presented herein for the specific case of a TEPP based on pulverized coal combustion. The TEPP was modified for a co-firing operation where rice straw has a share of up to 10% of the energetic base.
4.1 Problem identification
Figure 4 shows the representative flowchart for the previously defined boundary. The flowchart also shows the input and output flows of each of the four stages, showing the mass and energy balances for every stage. Note that for the problem addressed, the main flows are the electricity consumed by mining operations and during the processing of the combustibles prior to combustion; and the diesel used in the transportation of coal and in the trucks, ploughing, bale compressing, and finally, in hauling trucks that remove the bales from the fields.
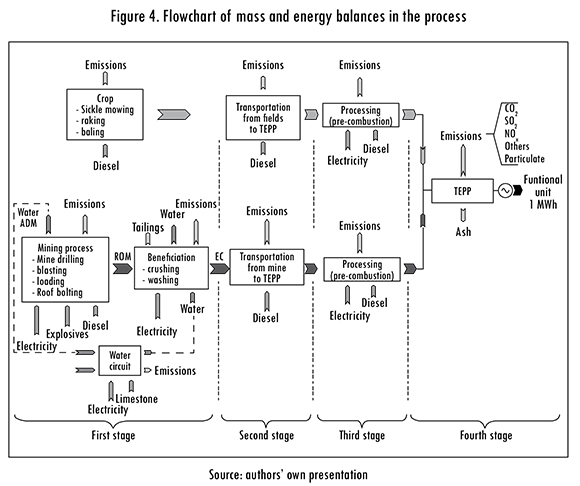
4.2 Exergetic and environmental analysis results
4.2.1 Exergetic analysis.
Table 3 reports the assessment of performance parameters for the 100% coal operation and for the co-firing operation (with a share of up to 10% of rice straw in the energetic bases). The parameters are: mass flow, exergy of the combustibles, the second law of thermodynamics -efficiency-, exergy destroyed in the TEPP, and global efficiency, considering the extended boundary.
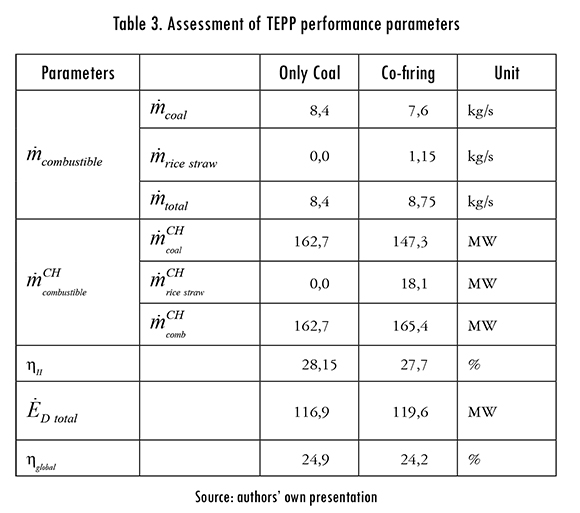
From a thermodynamic point of view, the results exhibited in Table 3 are unfavorable for the co-firing operation, since the performance parameters related to efficiency decrease and destroyed exergy increases. The decrease of the efficiency of the second law of thermodynamics is due to the efficiency decrease in the steam generator considered by the thermodynamic model during the co-firing operation. According to the co-firing test results of Hughes and EPRI [23], it is estimated at 0.5% for every 10% of biomass share in the process of combustion in energetic base.
Figure 5 shows the consumed exergy ratio considering the extended boundary. Note that the TEPP is responsible for a 95,6% of the total in the case of the coal-only operation. No significant changes take place for the co-firing operation, as expected, due to the high irreversibility associated to the combustion process occurring in the TEPP and also due to the low share of rice straw (10% of energetic base).
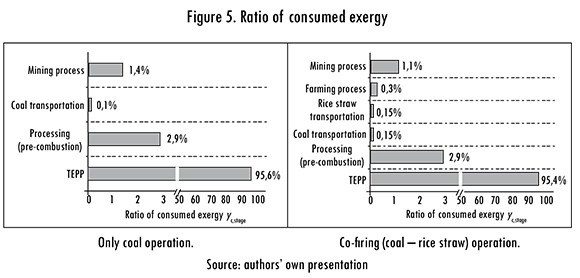
4.2.2 Environmental analysis
For the first part of LCA, 1 MWh generated by the TEPP was proposed as the functional unit. The impact category considered was global warning following IPCC 2007 100-year GWP and focusing on the total kg CO2 released as a parameter to identify the harmfulness of the process. As addressed by Heidi, Leif, and Anders [24], the possible effects of this category include temperature increase, causing polar ice-caps meltdown and thus an increase of the sea levels. The temperature increase can also give rise to climate changes at the regional level. Wherever GHG are released, they will contribute to the aforementioned effects and that is the reason why this impact category should be considered to have a global character.
After defining the boundary, the LCI described in Table 4 was performed. LCI takes into account two operational conditions of the TEPP: only-coal and co-firing with a 10% share of rice straw in the energetic base.
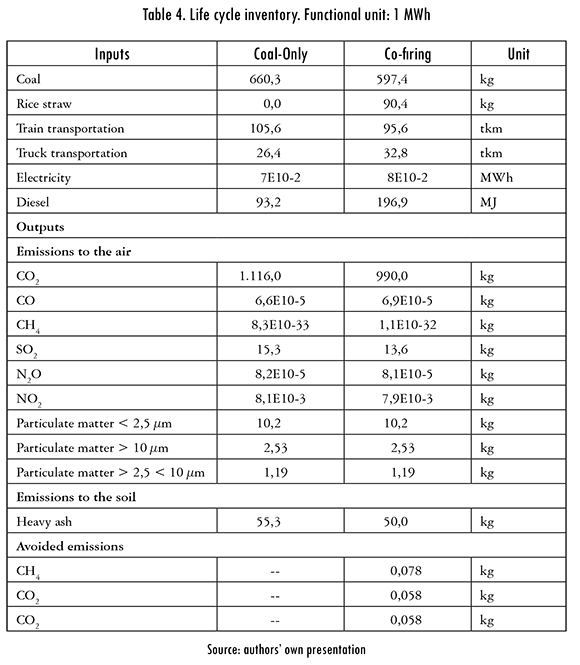
SimaPro 7.2 was used for the purposes of the LCIA. SimaPro is a recognized software for the environmental assessment of products or processes. The environmental model enabled us to know the impact distribution during the different stages within the boundary of the system (Figure 1). For the case of the only-coal operation, the identified value was 1,230 kg CO2-eq per MWh. Figure 6 a shows the percentage contribution to the total of kg de CO2 released; 91% is related to the TEPP and 9% is the result of the operations during extraction, beneficiation, transportation, and pre-combustion processing of the coal before entering the boilers of the steam generator. This result has the same order of magnitude as the results presented by Hondo, Odeah et al. and Spath et al. [5]-[7], when analyzing similar processes.
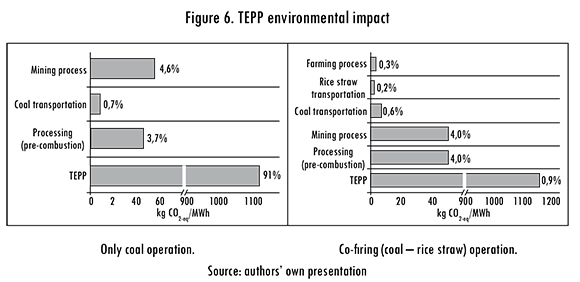
For the case of the co-firing operation, a total emission of 1,253 kg CO2 per MWh was reported, exceeding the amount of coal-only combustion. As previously explained, the cause of this increase is due to the fact that the model for the exergetic analysis considered a 0.5% efficiency loss of the steam generator for each 10% of rice straw contribution, thus forcing a larger participation of the combustible en masse. It is important to consider that 150 kg of CO2-eq of the total are released during rice straw combustion, but those 150 kg de CO2-eq can be regarded as neutral if we take into account the CO2 uptake during photosynthesis. Thus the net emission of CO2-eq is 1,103 kg de CO2-eq per MWh, giving an approximate reduction of 12%. Figure 6b shows that the TEPP is responsible for 90,9% of emissions in the co-firing operation while activities related to the obtaining, transportation, and pre-combustion processes of both coal and rice straw amount for 9,1% of the impact.
Table 4 reports the averted emissions in kg of CH4, CO2, and CO related to the avoided straw rice decomposition in the farming fields. Those values were estimated by considering that 50% of carbon present in the rice straw is incorporated again to the soil after the harvest and the other 50% is volatilized in these compounds, with proportions of 40% of CH4, 30% of CO2, and 30% of CO. Under the previous assumption, the total value decreases even more reaching 921 kg of CO2-eq per MWh, making the co-firing proposal very sensible for purposes beyond thermoelectric generation.
Finally it is important to state that the impact related to the burning of the combustibles was estimated using a combustion model in chemical equilibrium. Due to a lack of information, the impact related to the use of diesel, limestone, and explosives was taken from the Ecoinvent database for similar processes. It is also necessary to clarify that the environmental model did not take into account the construction and dismantling periods of the TEPP. According to the literature review, the CO2-eq contribution of those periods is less than 1% of the total estimated during the life cycle.
5. Conclusions
This paper shows the potential of the residual biomass as fuel in the TEPP, based on a technical (based on exergy) and environmental evaluation of the first experience in Brazil. Beyond the TEPP the boundary of the problem was expanded to include processes related to the extraction, obtaining, transportation, and handling of both the coal and the biomass. Two operational conditions were considered: only-coal and co-firing (coal and rice straw). Results revealed that the TEPP is responsible for more than 95% of the total consumed exergy in both operational conditions studied herein, as a consequence of the high irreversibility during the combustion process. For the environmental analysis a LCA was performed by means of the IPCC 2007 100-year GWP The impact category considered herein was global warming, focusing on the total kg CO2-eq per MWh released as a parameter to identify the harmfulness of the power generation. Results reported emissions of 1,230 kg of CO2-eq per MWh under the only-coal operation, whereas emissions of 1,103 kg de CO2-eq per MWh took place under the co-firing operation, which has a 10% share of biomass in the energetic base. When considering the averted emissions due to the avoidance of biomass decomposition in the farming fields the total value decreased further to 921 kg of CO2-eq per MWh, which makes the use of biomass a very sensible alternative for purposes beyond thermoelectric generation.
Acknowledgements
Authors would like to thank: Tractebel energia; Coordenação de Aperfeiçoamento de Pessoal de Nível Superior — CAPES; ACV-Brasil and SimaPro software.
Nomenclature
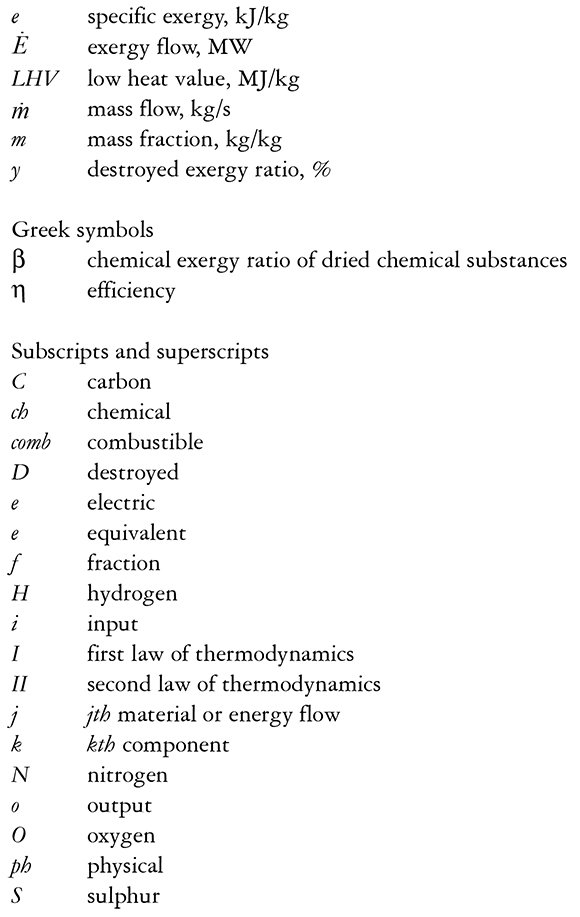
References
International Energy Agency, Key world energy statistics. Paris: IEA, 2013. [ Links ]
Electric Power Research Institute, Biomass Co-firing guidelines. Palo Alto California: EPRI, 1997. [ Links ]
J. Hansson et al., "Co-firing biomass with coal for electricity generation. An assessment of the potential in EU27", Energy Policy, vol. 37, pp. 1444-1455, 2009. [ Links ]
D. A. Tillman, "Biomass co-firing: The technology, the experience, the combustion consequences", Biomass Bioenergy, vol. 19, pp. 365-384, 2000. [ Links ]
M. Hondo, "Life cycle GHG emission analysis of power generation systems: Japanese case", Energy, vol. 30, pp. 2042-2056, 2005. [ Links ]
A. Odeah, T. Naser, and T. Cockerill, "Life cycle analysis of UK coal fired power plants", Energy Conversion Management, vol. 49, pp. 212-220, 2008. [ Links ]
P. Spath, M. Mann, and D. Kerr, Life cycle assessment of coal-fired power production. Golden, Colorado: National Renewable Energy Laboratory, 1999. [ Links ]
F. Pereira, E. Bazzo, and A. Martins de Oliveira, "Biomass co-firing as an alternative technology for a clean coal electric generation in Brazil", en 20th International Congress of Mechanical Engineering, Gramados, RS, Brazil, 2009. [ Links ]
R. Miyake, E. Bazzo, and M. Bzuneck, "Cofiring of rice straw and coal in a coal-fired utility boiler: thermodynamic analysis", en 13th Brazilian Congress Thermal Sciences Engineering, Uberlandia, MG, Brazil, 2010. [ Links ]
G. Moreno-Contreras, J. Serrano-Rico y J. Palacios-Restrepo, "Desempeño de la combustión de desechos industriales en un reactor de lecho fluidizado burbujeante", Ingeniería Universidad, vol. 13, no. 2, pp. 251-266, 2009. [ Links ]
F. Sebastián, J. Royo, and M. Gómez, "Cofiring versus biomass-fired power plants: GHG (greenhouse gases) emissions savings comparison by means of LCA (life cycle assessment) methodology". Energy, vol. 36, pp. 2029-2037, 2011. [ Links ]
H. Ming-Che, H. An-Lei, and W. Tzai-Hung, "GIS-based biomass resource utilization for rice straw cofiring in the Taiwanese power market", Energy, vol. 55, pp. 354-360, 2013. [ Links ]
S. Shafie, T. Mahlia, and H. Masjuki, "Life cycle assessment of rice straw co-firing with coal power generation in Malaysia", Energy, vol. 57, pp. 284-294, 2013. [ Links ]
W. Schakel et al., "Comparative life cycle assessment of biomass co-firing plants with carbon capture and storage", Applied Energy, vol. 13, pp. 441-467, 2014. [ Links ]
ISO, FDIS 14040, Environmental management. Life cycle assessment. Principles and framework. Ginebra: ISO, 2006. [ Links ]
ISO, FDIS 14044, Environmental management. Life cycle assessment. Requirements and guidelines Managements environmental. Analyse du cycle de vie. Ginebra: ISO, 2006. [ Links ]
T. Süffert, Carvão nos Estados do Rio Grande do Sul e Santa Catarina, Porto Alegre. Informe de Recursos Minerais. Série Oportunidades Minerais CPRM, 1997. [ Links ]
M. Yore, M. Summers, and B. Jenkins, "Development of a stubble cutting system for a combine harvester", ASAE Paper Number: 01-1087. ASAE Annual International Meeting. Sacramento, CA, 2001. [ Links ]
IFK, "Report of rice straw and Brazilian coal analyses", Institutfür Feuerungs -und Kraftwerkste-chnik - Universitãt Stuttgart. Stuttgart, 2009. [en alemán] [ Links ].
J. Szargut, D. Morris, and F. Steward, Exergy analysis of thermal, chemical and metallurgical processes. New York: Hemisphere, 1988. [ Links ]
A. Bejan, G. Tsatsaronis, and M. Moran, Thermal design and optimization. Hoboken, NJ: John Wiley & Sons, 1996. [ Links ]
A. Restrepo, "Metodologia de análise e avaliação exergoambiental de plantas termoelétricas operando em combustão combinada carvão", Tesis, Universidade Federal de Santa Catarina, Florianópolis, Brasil, 2012. [ Links ]
E. Hughes and EPRI, Biomass Cofiring: field test results. Palo Alto, CA: Electric Power Research Institute, 1999. [ Links ]
K. Heidi, H. Leif, and S. Anders, Update on impact categories, normalisation and weighting in LCA. Danish: Ministry of the Environment, Environmental Project Nr. 995, 2005. [ Links ]