Serviços Personalizados
Journal
Artigo
Indicadores
-
Citado por SciELO
-
Acessos
Links relacionados
-
Citado por Google
-
Similares em SciELO
-
Similares em Google
Compartilhar
Ingeniería y Universidad
versão impressa ISSN 0123-2126
Ing. Univ. vol.19 no.1 Bogotá jan./jun. 2015
https://doi.org/10.11144/Javeriana.iyu19-1.tdkp
Thermal Degradation Kinetic of Polylactic Acid in Multiple Extrusions1
Cinética de degradación térmica de poliácido láctico en múltiples extrusiones2
Andrés Felipe Rojas-González3
Javier Ignacio Carrero-Mantilla4
1Submitted on: March 14th, 2014. Accepted on: November 24th, 2014. The article "Thermal degradation kinetic of polylactic acid in multiple extrusions" is the product of the investigation Project named: Implementación de modelos matemáticos en el estudio de la degradación térmica del poliácido láctico, PLA.
2Fecha de recepción: 14 de marzo de 2014. Fecha de aceptación: 24 de noviembre de 2014. El artículo "Cinética de degradación térmica de poliácido láctico en múltiples extrusiones" es el resultado del proyecto de investigación titulado: Implementación de modelos matemáticos en el estudio de la degradación térmica del poliácido láctico, PLA. con código 15704 , financiado por la Universidad Nacional de Colombia sede Manizales bajo la Convocatoria: Programa para financiación de semilleros de investigación en pregrado en la Universidad Nacional de Colombia sede Manizales - 2012.
3Ingeniero químico, Universidad Nacional. Magíster en ingeniería, Universidad del Valle. Doctor en ingeniería, Universidad del Valle. Profesor, departamento de ingeniería química, Universidad Nacional de Colombia sede Manizales. Bloque L, campus La Nubia, km. 5 vía al aeropuerto, Manizales, Colombia. Correo electrónico: anfrojasgo@unal.edu.co.
4Ingeniero químico, Universidad del Valle. Magíster en ingeniería, Universidad del Valle. Doctor en ingeniería, Universidad del Valle. Profesor, departamento de ingeniería química, Universidad Nacional de Colombia sede Manizales. Manizales, Colombia. Correo electrónico: jicarrerom@unal.edu.co.
Para citar este artículo / To cite this article
A. F. Rojas-Gonzáles and J. I. Carrero-Mantilla, "Thermal degradation kinetic of polylactic acid in multiple extrusions", Ing. Univ., vol. 19, no. 1, pp. 189-206, Ene.-Jun., 2015. http://dx.doi.org/10.11144/Javeriana.iyu19-1.tdkp
Abstract
Results of a thermal degradation kinetic study of polylactic acid (PLA), raw and reprocessed by extrusion are shown. The reprocessed samples were obtained after each one of five successive extrusions. The samples were tested for glass transition temperature, melt flow index, higher heating value, and dynamic thermogravimetry. Kinetic triplet was determined from the dynamic thermogravimetry data by Flynn-Wall-Ozawa (FWO), Friedman, Gyulai, Kissinger-Akahira-Sunose (KAS), Kissinger, Differential and Integral Arrhenius, Briodo, Chang, Coats-Redfern, Flynn Wall, Horowitz-Metzger, MacCallum-Tanner, Madhusudanan, and Dynamic methods. It was found that activation energy (E) increases with the reprocessing, and that frequency factor (A) increases with the heating rate. It indicates that PLA experiences thermal degradation when is processed by continuous extrusion. It was also found that the average kinetic triplet obtained by isoconversional methods is E=147.14 kJ/mol, ln(A)= 26.94 (with A in min-1), and reaction order n = 0.86, and the triplet averaged from non-isoconversional methods is E = 225.30 kJ/mol, ln(A)= 45.07, and n = 0.91.
Keywords: thermal degradation; kinetic triplet; multiple extrusions; PLA
Resumen
Este artículo presenta los resultados del estudio cinético de la degradación térmica del poliácido láctico (PLA, por sus iniciales en inglés), sin procesar y reprocesado por extrusión. Las muestras reprocesadas se obtuvieron después de cada uno de los cinco ciclos de extrusión y se analizaron para obtener la temperatura de transición vítrea, el índice de fluidez, el poder calorífico superior y la termogravime-tría dinámica. Se determinó el triplete cinético usando los datos de termogravimetría dinámica por medio de los métodos Flynn-Wall-Ozawa (FWO), Friedman, Gyulai, Kissinger-Akahira-Sunose (KAS), Kissinger, Arrhenius Differential e Integral, Briodo, Chang, Coats-Redfern, Flynn-Wall, Horowitz-Metzger, MacCallum-Tanner, Madhusudanan y dinámico. Se encontró que la energía de activación (E) disminuye con el reprocesamiento y que el factor de frecuencia (A) aumenta con la velocidad de calentamiento. Esto indica que el PLA experimenta degradación térmica al ser sometido a procesos continuos de extrusión. También con métodos isoconversionales se obtuvo un triple-te cinético promedio de E = 147,14 kJ/mol, ln(A) = 26,94 (con A en min-1 ), y orden de reacción n = 0,86; el triplete promedio obtenido con métodos no isoconversionales es E=225,30 kJ/mol, ln(A)= 45,07, y n = 0,91.
Palabras clave: degradación térmica; triplete cinético; múltiples extrusiones; PLA
1. Introduction
Due to the need for recycling during the life cycle of materials, reprocessing is appealing in the production of plastic, because it minimizes waste and allows reaping economic benefits by reducing the consumption of raw materials [1]. Reprocessing is defined as the operation that returns material already processed to the production line substituting part of the raw material [2]. During each reprocessing the polymer experiences i) thermal and mechanical degradation, ii) physical chemical changes, and iii) a loss of mechanical properties that make the polymer brittle and reduces its life span [3].
The degradation processes of polymers occur not only during their production, processing, and reprocessing; but also during their final life stage: the waste disposal [4]. Reprocess-induced degradation is mechanical and thermal; it breaks the polymer's mean chain, which reduces the molecular weight and the viscosity, and increases the melt flow index [5]. Reprocessing also changes other properties such as glass transition temperature (Tg), crystallinity percentage, and flow index (FI). However, the nature of the change is completely variable for different types of polymer and process [2], [6].
Besides Tg and FI measurements the effect of reprocessing can be quantified with the higher heating value (Q) and thermogravimetric analysis (TGA). The latter is one of the most applied thermal analysis techniques in the study of solid materials' thermal degradation [7]. In this analysis the weight loss is measured as a function of temperature (TG curve or gravimetric thermogram). The derivative of the TG curve is named differential thermogravimetric thermogram, or DTG curve [8]. The DTG curve is used to obtain the temperature at the maximum weight loss rate (peak temperature, Tm) [9], and the TG/DTG data are employed to establish the degradation kinetics by means of isoconversional and non-isoconversional methods. [10], [11].
The purpose of this work is to study the effect of five cycles of extrusion processing on the properties and thermal degradation kinetics of polylactic acid (PLA). To this end Tg, FI, and Q were measured and TG/DTG curves were obtained at four heating rates (B). The reprocessing-induced degradation was quantified, and the kinetic triplet was determined from the TG/DTG data using 10 non-isoconversional and 5 isoconversional methods.
2. Materials and methods
2.1. Raw polymer properties and extrusion
Extrusion-grade polylactic acid (PLA) was supplied by Nature Works — USA. This polymer had a flow index of 14.2 g/10min (210°C/2.16kg), with glass transition, fusion, and crystallization temperatures of 63°C, 165°C, and 120°C respectively. The PLA was subject to five reprocessing cycles. Each one included the same operations: an initial drying, extrusion, cooling, size reduction, and a second drying. The size obtained was 2mm (diameter) by 3mm (height), approximately. The drying was held in an oven at 90°C for one hour. The extruder was a single-screw EXTRUDEX ED-N 45-30D, with a 45mm screw diameter, and a length/diameter ratio L/D=30. It was operated with a polymer exit dosi-fier restriction of 10 (minimum is 0, and maximum is 56), a 30 rpm rotating speed, and a 180/190/200/205/210/210/210/210 temperature profile for the extruder's eight heating zones.
2.2. Extruded polymer characterization
Samples of raw and extruded polymer were characterized with Tg, FI, Q, and thermogravimetric curves. The glass transition temperature was measured following the ASTM D3418-08 standard in a Netzsch DSC 200-PC differential scanning calorimeter. The samples, with a 10,0 ± 1,0 mg mass were subject to this controlled heating / cooling program: heating from 20°C to 160°C, constant (160°C) temperature for 5 minutes, followed by cooling from 160°C to 20°C. Flow index was measured in a Dynisco D4003 melt flow indexer. For PLA the FI is measured with the mass that flows through a capillary tube in a 10 min lapse, at 210°C, and an equipment mass of 2,16kg (ASTM D1238 standard). The calorific value was measured in a LECO AC 350 calorimeter following the standard ASTM D5865 using 1g samples. Themogravimetric (TGA) analysis was carried out in a TA Instruments TGA SDT Q600 analyzer. Samples with mass between 10mg and 15mg were heated from room temperature to 600°C at four heating rates, B = 5, 10, 20, and 40°C/min in inert atmosphere with a nitrogen flow of 50mL/min.
2.3. Quantification of reprocessing degradation
The extent of degradation induced by the polymer processing was quantified using the Processing Degradation Index, PDI, [12]:

where abs means absolute value, n is the number of reprocessing cycles, and FI. is the flow index in the reprocessing i (for raw polymer i=0). Degradation is proportional to PDI; an ideal, non-degraded polymer has PDI = 0, while PDI » 1 indicates a very high degradation.
2.4. Kinetic triplet determination
Activation energy (E), frequency factor (A), and reaction order (n) values were calculated with the isoconversional and non-isoconversional methods described in Table 1. The non-isoconversional methods are differential (with an assumed n = 1), Arrhenius integral (n = 1), Briodo (n = 1), Chang, Coats-Redfern, Flynn-Wall, Horowitz-Metzger (n = 1), MacCallum-Tanner, Madhusudanan, and dynamic method. The isoconversional methods are Flynn-Wall-Ozawa (n = 1), Friedman, Gyulai- Greenhow (n = 1), Kissinger-Akahira-Sunose (n = 1) and Kissinger (n = 1). Temperature and conversión (a) data were fitted to the isoconversional and non-isoconversional models using functions written for the software Scilab-5.4.0.
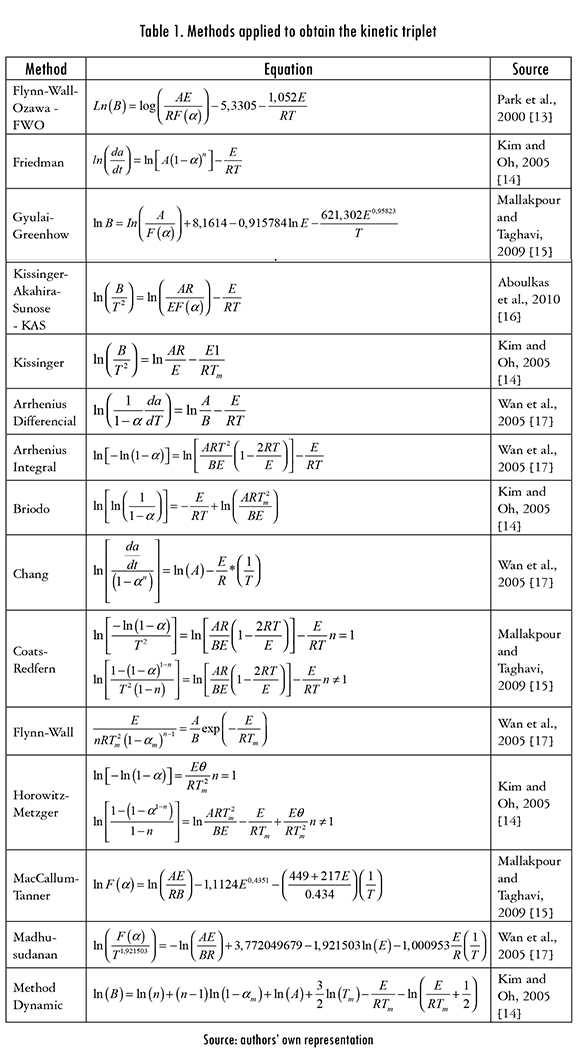
3.1. Extrudedpolymer characterization
Raw and processed polymer Tg, FI, and Q values are shown in Table 2. It can be noticed that Tg varies only slightly with the number of extrusions (Ext. 1 — Ext. 5), and hence it can be assumed that reprocessing does not alter such parameter. Although Q reduced with each extrusion this effect is small and the reduction for all reprocessed samples is below 0.5%. On the contrary, FI increases with each reprocessing, which suggests that polymer's molecular structure was degraded. This result also explains the reduction of Q with reprocessing.
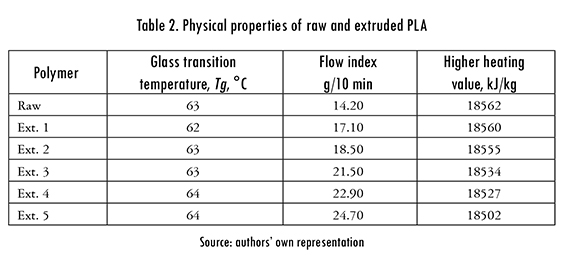
3.2. Processing degradation index
In Figure 1 each gray tone represents the degradation experienced by PLA in each reprocess in terms of the PDI. The first reprocessing produces most degradation, and as expected the effect is accumulative with the number of reprocesses. There is a linear relationship between the number of reprocess (P) and PDI: PDI=2.636 + 7.446P, with determination coefficient R2 = 0.998. This is in agreement with thermal degradation results obtained from TG curves, see Figure 3.
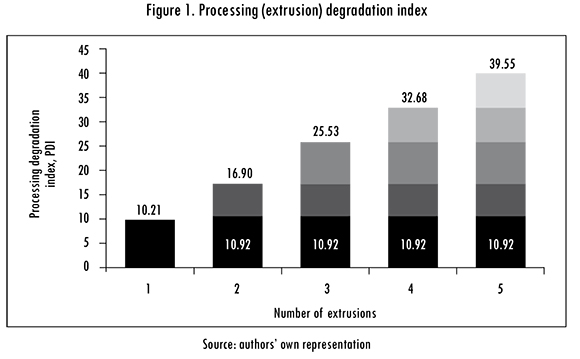
3.3. Thermogravimetric analysis
The TG and DTG analyses were done at four heating rates (B) for each extrusion. TG and DTG curves for extrusion 5 with all Bs are shown in Figure 2. The curves for B=10°C/min for all five extrusions are in Figure 3. These results are representative of the other extrusions and heating rates.
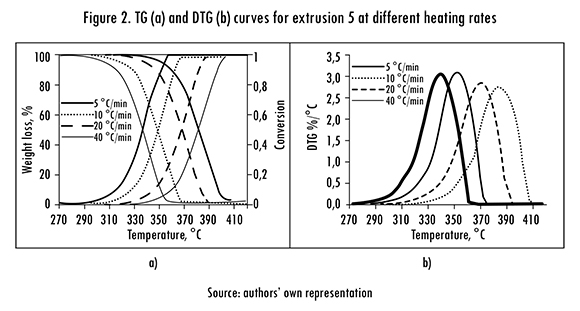
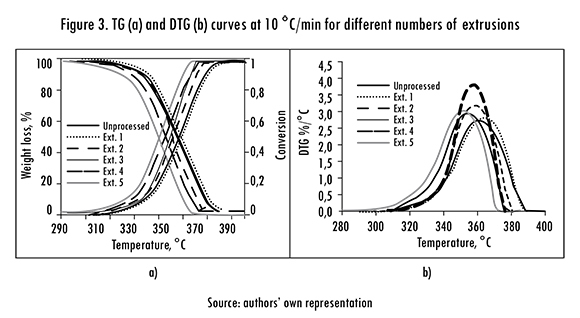
The TG a curves displace to the right when B increases (Figure 2a), it implies that the same a will be reached at a higher temperature. Therefore the increase in B causes a delay in material's degradation, which reflects in the shift to the right in the a curves. The same behavior appeared with all samples, in agreement with results reported for commercial rubber [18]. This effect has been attributed to the change in the mechanism of reaction when B is increased [13]. Similar results were obtained for DTG, when B increases the curves shift to the right and the peaks appear at higher temperature (Figure 2b). This can also be attributed to the reduction of reaction time, the final temperature of the thermogravimetric analysis is reached in a shorter time [18].
Figure 3 shows TG and DTG curves obtained with a 10°C/min heating rate for the unprocessed sample and the five reprocesses. The thick black solid line represents the polymer unprocessed data, the first reprocessing is the dotted line, the second is the thin dashed line, the third continuous thin line, the thick fourth and fifth dashed line is the gray line reprocessing. It is observed that the thermal degradation profile and the a curve exhibit a subtle shift to the left, it suggests that: i) polymer degradation affects very little the PLA thermal properties, ii) there is a small variation in the degradation mechanisms. It can be also noticed that reprocessed samples reach the same a value with a lower temperature, which confirms that the polymer degrades with reprocessing. Figure 3b shows that DTG curves have a single peak, indicating a single stage thermal degradation process in the reprocessed samples [16]. A similar behavior appears at different Bs (Figure 2b).
The variation of Tm with the number of reprocesses, for each B, is shown in Figure 4. T increases with B at each extrusion, but it decreases with the number of extrusions for a given B. Therefore, considering Tm as an indicator, it is concluded that PLA suffers a small degree of thermal degradation with reprocessing.
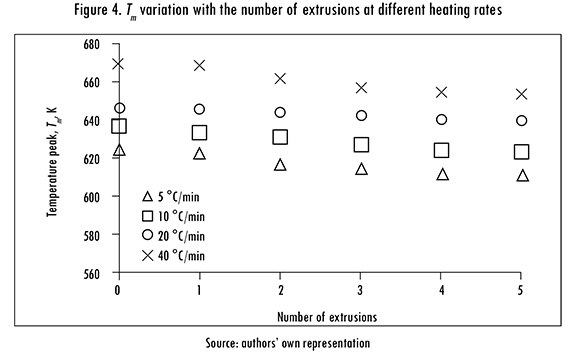
3.4. Thermal degradation kinetics — kinetic triplet
Results from non-isocoversional methods assuming first order reactions are shown in Table 3 for the four heating rates (B). It can be observed in the kinetic triplet values that both A and E increase with B. The parameter A is a probability measure for polymer molecules collisions that lead to a degradation reaction [19]. According to the Table in most samples such probability, i.e. A, increases with B, an expected result because the kinetic energy of the molecules increases in a shorter time and therefore a higher number of them will have enough energy to reach the transition state and react. It is worth to mention that the Horowitz-Metzger method gives the lowest E values for any B and number of reprocesses, while Briodo and Arrehenius integral methods give the same E values, alongside with very close A values.
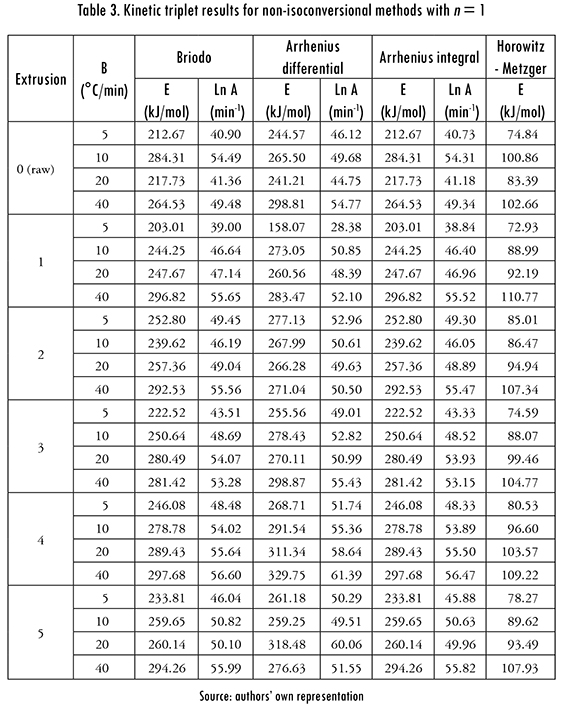
For the other non-isoconversional methods (MacCallum-Tanner, Madhusu-danan, Coats-Redfern, Flynn-Wall, Chang, and dynamic), which are not shown in this document, n ^ 1 reaction orders were found, with values between 0,56 and 1,10, and a 0,86 average (it is calculated with results from all five extrusions and four heating rates). It was also found that n is approximately the same for MacCallum-Tanner, Madhusudanan, Coats-Redfern, and dynamic methods. Average n for Flynn-Wall method was 0,69, and 1,07 for Chang method, which gives the highest n values, close to 1. The average values are calculated as the arithmetic average of all the data obtained in 5 extrusions and 4 Bs. It was also found that, with a fixed heating rate there is not a definite relationship between n and the number of reprocesses, and that n tends to increase with B [13].
The averaged ln A obtained with dynamical method was 37.91 (with A in min-1), the Chang method gave the highest values (55.57), Flynn-Wall gave the lowest (29.43), while MacCallum-Tanner (44.92), Madhusudanan (43.82), and Coats-Redfern, (43.75) gave similar values. The highest E values were obtained with the Chang method, a 294.72 kJ/mol average, followed by MacCallum-Tan-ner (240.13 kJ/mol), Madhusudanan (229.43 kJ/mol), Coast-Redfern (228.74 kJ/mol), dynamic (219.74 kJ/mol), and Flynn-Wall methods (158.13 kJ/mol).
Kinetic triplet results for isoconversional Kissinger, KAS, FWO, Gyulai, and Friedman methods are included in Table 4. The values are averages from the four Bs and conversions 0.05, 0.1, 0.2, 0.3, 0.4, 0.5, 0.6, 0.7, 0.8, 0.9 and 0.95 in each reprocess. Parameter n was 1, except for Friedman method, which gave ns that vary slightly with reprocess, between 0,23 and 0.39. It is also observed in this Table that E and the frequency factor decrease with the number of reprocesses.
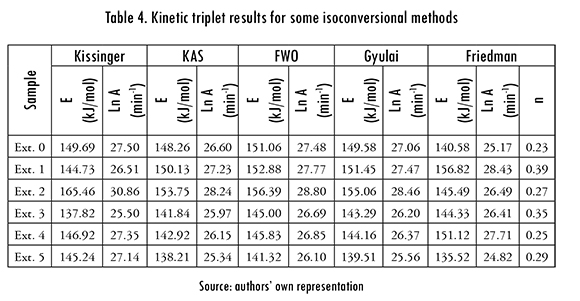
The analysis of A, E, n results is challenging because all methods give different values, as can be observed in Tables 3 and 4. But all these methods are data fit models and their goodness of fit was measured defining a square error with the differences between left-hand and right-hand sides (y'., yr. ) of the equations in Table 1, divided by the smallest value. The residuals average was calculated as

where N is the number of experimental points, and higher lSr values indicate a better fit. Results for isoconversional methods are presented in Table 5, the Kissinger method produced the best fit.
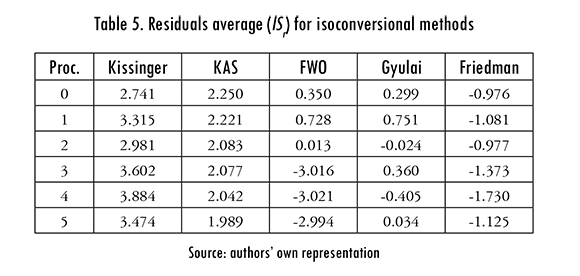
The variation of E, A (calculated with KAS method) with a is shown in Figure 5. The activation energy E increases with a, except for the second reprocess, in which E remained almost constant. This behavior indicates that degradation is delayed because the material becomes more refractory, and more stable, when it decomposes due to the temperature increase [19], [20].
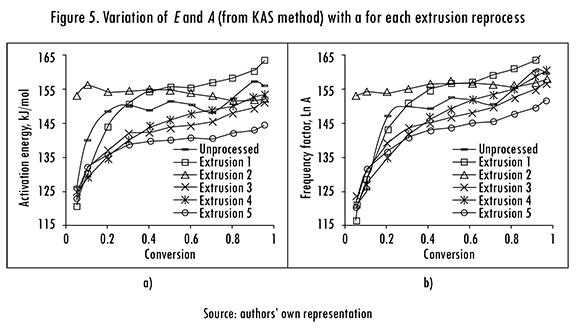
It is also observed that E increases in the 20-150 kJ/mol range for 0.05 < a < 0.30, and remains almost constant between a =0.35 and a =0.95. These values are in agreement with previously reported PLA thermal degradation results obtained by different methods [21]. Despite the initial increase the change of E was not significant, which suggest that polymer's decomposition occurs in a single stage [16]. In Figure 5b it is shown that in most extrusion reprocesses ln A increases from a =0.05 to a =0.2, and then it remains almost constant with values between 25.5 and 29.0. These behaviors of E and ln A were also observed in the results from FWO and Gyulai methods (Table 4).
The variation of E (obtained from isoconversional and non-isoconversional methods) with the number of reprocesses is shown in Figure 6 for a heating rate of 10°C/min. The tendencies observed in this Figure are the same for the other Bs. Regarding the isoconversional methods (Figure 6a) KAS, FWO, and Gyu-lai results have the same tendency, while results from Kissinger method show two maxima in reprocesses 2 and 4. The variation of E calculated with KAS, FWO, and Gyulai methods is similar to reprocessing results reported for other polymers [6]. Briodo, Arrhenius integral, Madhusudanan, and Coats-Redfern methods show the same results in Figure 6b. It can also be seen that Chang method gives the highest E values and Horowitz-Metzger the lowest, and that the method with the highest E variation is Flynn-Wall.
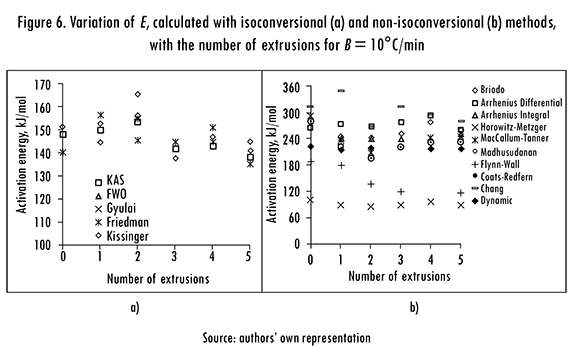
The variation of n with the number of reprocesses, calculated with n ≠ 1 non-isoconversional methods is shown in Figure 7 for B = 10°C/min. In the results from MacCallum-Tanner, Madhusudanan, and Coats-Redfern methods n varies in the same way, giving similar values for each extrusion. With Flynn-Wall method n is approximately 1 up to the third reprocess, after that it falls to a value close to 0.6. The Chang method gives the highest n values, while Coats-Redfern gives the lowest ones.
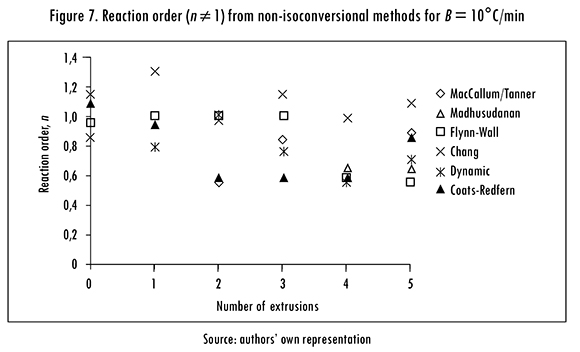
Averaged values of E, A, n are shown for each extrusion in Figure 8. Iso-conversional results were obtained in the same way described for Table 4; but non-isoconversional results from all Bs were averaged first for each extrusion and the resulting values were then averaged for each method. In this Figure it can be seen that E, A, n values from isoconversional methods are lower than the respective values from non-isoconversional methods. This behavior indicates that kinetic triplet, mainly E, is influenced by a; in fact, when the effect of a on is taken in account E reduces 25%.
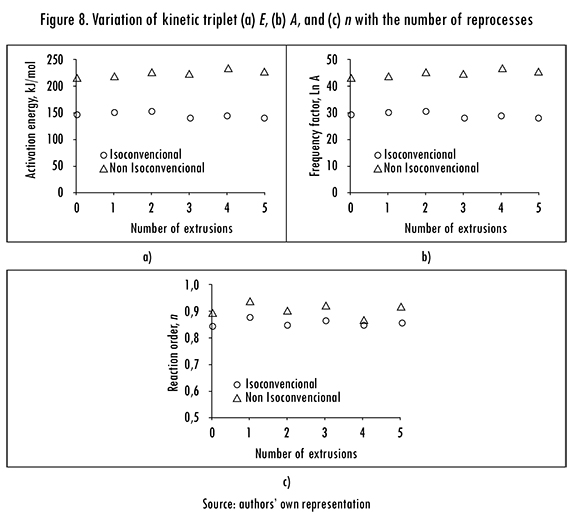
Figure 8a shows that E increases with the number of reprocesses when it is calculated with isoconversional methods, but it decreases when calculated from non-isoconversional methods. It indicates that isoconversional methods adequately represent the thermal degradation behavior of PLA, in successive thermal treatments it suffers bond rupture and its degradation requires less energy [22]. The parameter A increases with the number of extrusions (Figure 8b), and it is more evident for results from non-isoconversional methods. On the other hand n decreases slightly with the number of reprocesses (Figure 8c), which indicates that degradation produces smaller molecules, reducing the number of bonds to be broken.
The kinetic triplet averages from raw and extruded polymer samples are E = 147.14 kJ/mol and 225.30 kJ/mol, A = 26.94 and 45.07 min1, and, n = 0.86 and 0.91 from isoconversional and non-isoconversional methods, respectively.
4. Conclusions
Extrusion reprocessing of polylactic acid barely alters its glass transition temperature and heating value. But its melt flow index changes noticeably, and processing degradation index results indicate that most of the effect comes from the first reprocess.
Thermogravimetry results suggest that PLA decomposition occurs in a single stage, with a reaction order 1. The Kissinger method gave the most appropriate description of the thermal decomposition kinetics, with activation energies between 137.82 and 165.46 kJ/mol, and preexponential factor (Ln A) values between 25.50 and 30.86, with A in min1.
It was found that the averaged kinetic triplet of PLA extruded in five reprocesses, from isoconversional and non-isoconversional methods has E values between 147.14 and 225.30 kJ/mol, A values between 26.94 and 45.07 min1, and n values between 0.86 and 0.91, respectively.
References
[1] T. Osswald, E. Baur, K. Oberbach, and E. Schmachtenberg, International Plastic Handbook: The Resource for Plastics Engineers. Alemania: Hanser Publishers, 2006. [ Links ]
[2] A. A. Mendes, A. Cunha, and C. Bernardo, "Study of the degradation mechanisms of polyethylene during reprocessing", Polym Degrad Stab., vol. 96, pp. 1125-1133, 2011. [ Links ]
[3] K. Jitendra, K. Pondey, A. Ragunata, K. Prep, and R. Sing, "An overview on the degradability of polymer nanocomposites", Polym Degrad Stabil., vol. 88, pp. 234-250, 2005. [ Links ]
[4] M. Jakic, N. Stipanelov, and I. Klari, "Thermal degradation of poly(vinyl chloride)/poly(ethylene oxide) blends: Thermogravimetric analysis", Polym Degrad Stab., vol. 98, pp. 1738-1743, 2013. [ Links ]
[5] P. Wilichowski, "Comparison of effect of smooth and grooved feed extrusion on HDPE recyclability", M. Sc. Thesis, Univ. Winsconsin, Madison, USA, 2004. [ Links ]
[6] J. Pérez, J. Vilas, J. Laza, S. Arnáiz, F. Milangos, E. Bilbao et al., "Effect of reprocessing and accelerated ageing on thermal and mechanical polycarbonate properties", J Mater Proces Technol., vol. 210, pp. 727-733, 2010. [ Links ]
[7] R. Valapa, G. Pugazhenthi, and V. Katiyar, "Thermal degradation kinetics of sucrose palmitate reinforced poly(lactic acid) biocomposites", Int J Biolog Macromol., vol. 65, pp. 275-283, 2014. [ Links ]
[8] W. Grellmann and S. Seidler, Polymer Testing. Munich: Editorial Hanser, 2007. [ Links ]
[9] F. Carrasco, P. Pages, J. Gámez-Pérez, O. O. Santana, and M. Maspoch, "Processing of poly(lactic acid): characterization of chemical structure, thermal stability and mechanical properties", Polym Degrad Stab., vol. 95, pp. 116-125, 2010. [ Links ]
[10] D. S. Achilias, E. Panayotidou, and I. Zuburtikudis, "Thermal degradation kinetics and isoconversional analysis of biodegradable poly(3-hydroxybutyrate)/organomodified montmorillonite nanocomposites", Thermochim Acta, vol. 514, pp. 58-66, 2011. [ Links ]
[11] Z. Ma, D. Chen, J. Gu, B. Bao, and Q. Zhang, "Determination of pyrolysis characteristics and kinetics of palm kernel shell using TGA-FTIR and model-free integral methods", Energ Convers Manag., vol. 89, pp. 251-259, 2015. [ Links ]
[12] J. Tochacek and J. Jancar, "Processing degradation index (PDI). A quantitative measure of processing stability of polypropylene", Polym Test., vol. 31, pp. 1115-1120, 2012. [ Links ]
[13] J. W Park, S. C. Oh, H. P Lee, H. T. Kim, and K. O. Yoo, "Kinetic analysis of thermal decomposition of polymer using a dynamic model", Korean J Chem. Eng., vol. 17, pp. 489-496, 2000. [ Links ]
[14] H. Kim and S. Oh, "Kinetics of thermal degradation of waste polypropylene and high-density polyethylene", J Indust Engin Chem., vol. 11, num. 5, pp. 648-656, 2005. [ Links ]
[15] S. Mallakpour and M. Taghavi, "The accuracy of approximation equations in the study of thermal decomposition behavior of some systhesized optically active polyamides", Iranian Polym J., vol. 18, num. 11, pp. 857-872, 2009. [ Links ]
[16] A. Aboulkas, K. El Harfi, and A. El Bouadili, "Thermal degradation behaviors of polyethylene and polupropylene. Part I: Pyrolysis", Energy Conv. Manag., vol. 51, pp. 1363-1369, 2010. [ Links ]
[17] W B. Wan, F. N. Ani, and H. H. Masjuki, "Thermal stability evaluation of palm oil as energy transport media", Energy Conv Manag., vol. 46, pp. 2198-2215, 2005. [ Links ]
[18] W Kim, S. Kim, S. Lee, and I. Hong, "Kinetic characterization of thermal degradation process for commercial rubbers", J Ind Engin Chem., vol. 6, pp. 348-355, 2000. [ Links ]
[19] J. C. Santos, I. M. Dos Santos, A. G. Desousa, S. Prasad, and A. V Dos Santos, "Thermal stability and kinetic study on thermal decomposition of commercial edible oils by ther-mogravimetry", J Food Sci., vol. 67, num. 4, pp. 1393-1398, 2006. [ Links ]
[20] J. Badia, L. Santonja, A. Martínez-Felipe, and A. Ribes-Greus, "Reprocessed polyactide: studies of thermo-oxidative decomposition", Biores Technol., vol. 114, pp. 622-628, 2012. [ Links ]
[21] F. Carrasco, L. Pérez-Maqueda, IP Sánchez-Jiménez, A. Perejón, O. Santana, and M. Maspoch, "Enhanced general analytical equation for the kinetics of the thermal degradation of poly(lactic acid) driven by random scission", Polym Test., vol. 32, pp. 937-945, 2013. [ Links ]
[22] B. M. Roduit, "Computational aspects of kinetic analysis: Part E: The ICTAC Kinetics Project-numerical techniques and kinetics of solid state processes", Thermoch Acta, vol. 355, pp. 171-180, 2000. [ Links ]