Services on Demand
Journal
Article
Indicators
-
Cited by SciELO
-
Access statistics
Related links
-
Cited by Google
-
Similars in SciELO
-
Similars in Google
Share
Ingeniería y Universidad
Print version ISSN 0123-2126
Ing. Univ. vol.19 no.2 Bogotá July/Dec. 2015
https://doi.org/10.1114/javeriana.iyu19-2.kamr
Kinetics of Adsorption in Mercury Removal Using Cassava (Manhiot esculenta) and Lemon (Citrus limonum) Wastes Modified with Citric Acid1
Cinética de adsorción para la remoción de mercurio usando residuos de yuca (Manhiot esculenta) y limón (Citrus limonum) modificados con ácido cítrico2
Candelaria Tejada Tovar3
Ángel Villabona Ortiz4
Luz Ercilia Garcés Jaraba5
1Reception date: June 10,h, 2014. Acceptance date: March 2nd, 2015. This work is the result of a research project sponsored by Colciencias as part of the program of Jóvenes Investigadores.
2Fecha de recepción: 10 de junio de 2014. Fecha de aceptación: 2 de marzo de 2015. Este trabajo es resultado de un proyecto de investigación financiado por Colciencias en el marco del programa Jóvenes Investigadores.
3MSc. en Educación, MSc. en Ingeniería ambiental. Docente de planta de la Facultad de Ingeniería, Universidad de Cartagena, Colombia. E-mail: ctejadat@unicartagena.edu.co
4MSc. en Ingeniería Ambiental. Docente de planta de la Facultad de Ingeniería, Universidad de Cartagena, Colombia. E-mail: avillabonao@unicartagena.edu.co
5Ingeniera química. Investigadora, Universidad de Cartagena, Colombia. E-mail: luz.garces.jaraba@gmail.com
Para citar este artículo / To cite this article
C. Tejada Tovar, Á. Villabona Ortiz, and L. E. Garcés Jaraba, "Kinetics of adsorption in mercury removal using cassava (Manhiot esculenta) and lemon (Citrus limonum) wastes modified with citric acid", Ing. Unv., vol. 19, no. 2, pp. 283-298, 2015. http://dx.doi.org/10.1114/javeriana.iyu19-2.kamr
Abstract
The high toxic concentrations of mercury (Hg) in water bodies and its negative impact on the environment has resulted in the need for research on effective and low-cost methods for the treatment of industrial effluents, such as adsorption. This research compares the feasibility and viability of the Hg (II) adsorption abilities of cassava and lemon citric acid-modified peels. The results showed that the modified peel of cassava has a better adsorption ability compared to that of lemon. The kinetics models that best fit the experimental data were a pseudo-second order model and the Elovich model for both modified biomasses. This means that the mechanism that controls the adsorption is a second order reaction, and also shows that the catalytic area of the adsorbent is heterogeneous; whereas the Freundlich isotherm describes better the adsorption process. In conclusion, the use of waste material is possible for Hg (II) removal. The study of chemical modifications is suggested to improve the adsorption percentages.
Keywords: biomass; mercury; chemical modification
Resumen
Las altas concentraciones tóxicas de mercurio en los cuerpos de agua y su impacto negativo en el medio ambiente han generado la necesidad de estudiar métodos más económicos y eficaces para el tratamiento de los efluentes industriales, como es el caso de la adsorción. Esta investigación realizó un estudio de factibilidad, viabilidad y un comparativo entre las capacidades de adsorción de la cáscara de yuca y la cáscara de limón modificadas con ácido cítrico. Los resultados arrojaron que la cáscara de yuca modificada presenta una capacidad de adsorción mayor, en comparación con la cáscara de limón modificada. Los modelos cinéticos que más se ajustaron a los datos experimentales obtenidos fueron el modelo de seudosegundo orden y el de Elovich para las dos biomasas estudiadas modificadas, lo cual indica que el mecanismo controlante de la adsorción es una reacción de segundo orden y que la superficie catalítica del bioadsorbente es heterogénea; mientras que la isoterma de Freundlich describe mejor el proceso de adsorción. Se concluye que es posible el uso de materiales residuales para la remoción de Hg (II) y se sugiere el estudio de modificaciones químicas que puedan mejorar los porcentajes de adsorción.
Palabras clave: biomasa; mercurio; modificación química
Introduction
The high concentration of heavy metals in water bodies is primarily due to the development of anthropogenic activities. Industries such as mining, cement industry, dyes, tannery, electroplating, steel production, photographic material, corrosive paints, nuclear energy production, manufacturing of textiles, and aluminum anodizing present a great concentration of heavy metals such as chromium, nickel, cadmium, lead, and mercury in their effluents, which are not disposed of properly when they are let out into the environment [1]-[5]. Heavy metals are toxic and harmful, not only to human health but also to most life forms that live in neighboring ecosystems where they are poured. Metallic ions can be detected in the environment in their elemental state, which means they are not subject to biodegradation; this is why they are prone to be concentrated and collected by different species, becoming more dangerous as they rise in the evolutionary chain, until it reaches men [6]-[8].
Mercury is one of the most toxic ions of heavy metals, with a high rate of pollution in water bodies. Once emitted, it remains in the environment for years [9]. This metal can be found in nature in its inorganic forms: elemental [Hg (0)] or ionic [Hg (I) and (II)]; and its organic forms: methyl-mercury (CH3Hg) (MeHg), dimethyl-mercury [(CH3)2Hg], and phenyl-mercury (C6H?Hg) [10]-[12]. Hg (II) is inclined to join tightly to the components of the ground, which reduces its bioavailability. On the other hand, its organic forms, mainly MeHg, are highly toxic due to their high potential to bio-magnification, which means it tends to accumulate in biological membranes. In aquatic environments Hg can be consumed by Protozoa, and in turn these can be consumed by small invertebrates; fish eat invertebrates, and finally fish are eaten by humans. Once Hg has entered to the human body, and according to the ingested quantity, it causes different unfavorable effects in human health such as gastrointestinal diseases (increase in salivation, stomatitis, gingivitis, anorexia, nausea, vomiting, and diarrhea), respiratory diseases (rhinitis, anosmia, coughing, and fever), skin conditions (vesicular lesions and erythematous papular), and eye diseases (mercurialentis and constrictions in visual fields) [12], [13]. Due to the previously mentioned reasons, the necessity to treat effluents polluted by Hg becomes apparent, in order to avoid risks in the environment and for the health of all living creatures.
There are diverse methods in use today for treating this kind of metal-polluted water, among which are: chemical precipitation, membrane filtration, electrolytic reduction, solvent extraction, and ion exchange. However, many of these processes are expensive and inefficient due to their little applicability to different pollutants, generation of sludge that is difficult to eliminate, difficulty to find optimal operating conditions when there are different heavy metals in solutions, and the necessity of previous treatments [14]-[17]. Hence the need to apply environmentally "friendly" and more efficient remediation technologies such as adsorption, which allows big removal percentages of metallic ions; in addition, it uses lignocellulosic material for the adsorption process, taking advantage of waste that was not useful before. Among the most extensively reviewed waste biomass with excellent results, we can find pine sawdust [18], [19], sugarcane bagasse [20], and walnut shell [21].
Also, many authors have studied the mercury adsorption performance in aqueous solutions using residual biomass. Working with arborescent ferns, a maximum adsorption capacity of 26.5 mg/g was reached with a temperature of 25 degrees Celsius [22]; using eucalyptus bark, the maximum adsorption capacity recorded was 33.11 mg/g with 20 degrees Celsius [23]; using peels of guava, it was found that the Hg (II) removal is highly dependent on pH, reaching its maximum adsorption with a pH of 9 which was 3.3 mg/g, obtained after 80 minutes [24]; using wood of papaya the maximum removal was 70.8 mg/g with a pH of 6.5 [25]; using rice ears the maximum adsorption of metallic ions was 0.110 mmol/g. In addition, rice ears showed an excellent result as a bioadsorbent of mercury metal ions in industrial effluents [26]; using sawdust of ceiba sawdust, peel of green bean, and wastes of chickpea harvest, removal capacities of 25.88; 23.66 and 22.88 mg/g were obtained respectively [27]. Likewise, the modification of these materials has been studied, finding high efficient results; for example, pistachio shells and licorice wastes impregnated with zinc chloride have shown a maximum removal of 147.1 mg/g [28].
Within this context, this paper shows the kinetics of Hg (II) adsorption using lemon (Citrus limonum) and cassava (Manhiot esculenta) peel as waste biomasses; both biomaterials are considered good adsorbents according to consulted literature, given their easy treatment and preparation, high availability, easy attainment, and low cost. Adsorption tests were performed in batch system and cassava and lemon peels were chemically modified with citric acid in order to improve their adsorption capacity of Hg (II) ions.
1. Material and Methods
1.1. Preparation of Adsorbents Materials
Cassava and lemon peels were collected in some food companies in Cartagena, trying to pick them in their best possible condition, in order to guarantee the efficiency of the adsorption process. The plant material was washed with plenty of deionized water three times in a row in order to eliminate tannins, sugar gum, and other pollutants, which can alter the adsorption of metal ions [29]. Later, cassava and lemon peels were dried in an oven at 90 degrees Celsius for 24 h. The employed particle size for the cassava and lemon peels adsorption tests were 0.355-0.5 mm and 0.5-1, respectively. These sizes were chosen according to previous results obtained in non-modified biomass adsorptions tests. The waste biomass adaptation and treatment stages are summed up in Figure 1.
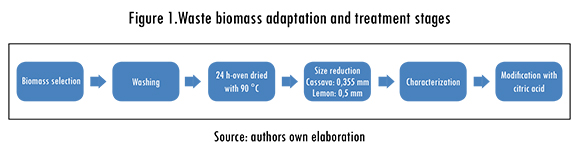
1.2. Characterization of Adsorbent Materials
Once prepared, the adsorbent materials were characterized in order to determine the elements and organic compounds that can be found in cassava and lemon peels, and can have an affinity with mercury ions. Different analytic methods were carried out in order to determine the presence of the distinctive elements of lignocellulosic materials, as indicated in Table 1. An FTIR analysis was also done to each biomass, before and after its chemical modification, and after the metal removal process, to identify functional groups that take part in adsorption. The adsorbent was enclosed in KBr pellets, in order to be analyzed by a spectrophotometer in the range of 4000-400 cm-1.
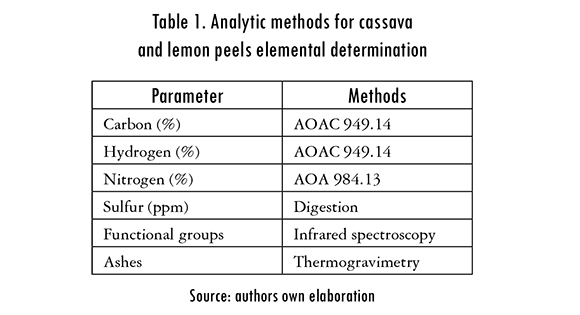
1.3. Modification with Citric Acid
Cassava and lemon peels were chemically modified with 0.6 M citric acid, in a 40g/200 mL biomass-acid relation; this mixture was left in constant stirring for 2 hours at 60 °C and 150 rpm. Afterwards, the biomass was washed with plenty of deionized water in order to remove excess acid; later, it was dried in an oven for 24 h at 55 °C. This modification was based on a carboxylation mechanism, in which the objective is to add carboxyl groups to the treated fiber, making up an anhydrous between two carboxylic groups. Then, the anhydrous reacts with a hydroxyl group of the biomass cellulose to form an ester and, in this way, increase the possibility of linking to the metal ion, as shown in Figure 2.
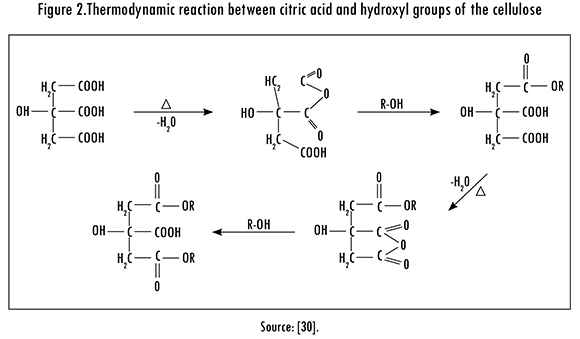
1.4. Adsorption Isotherm and Kinetics
The adsorption kinetics was carried out in order to determine the time in which the biomass reaches its maximum ability to retain mercury ions. The tests were held in a Shaking incubator IN-666 at 25 °C and 150 rpm, putting in contact 0.5 g of each modified biomass with 100 mL of a 100 ppm and pH 6 Hg (II) ions solution. 5 mL aliquots were taken each period between 10 and 330 min. The Hg (II) final concentration was calculated by UV-Vis spectroscopy in the supernatant. The kinetics models that come to terms with obtained experimental data are registered in Table 2.
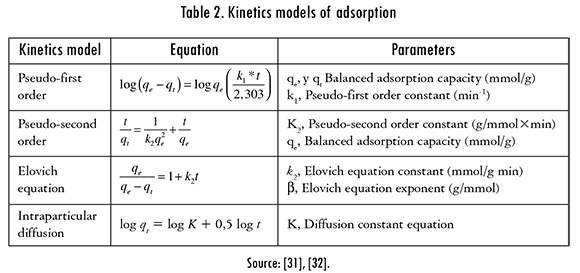
The adsorption isotherms models that were used for describing the separated solute between solid and liquid phases are presented in Table 3. In the carrying out of the tests, different Hg (II) starting concentrations (25; 50.75 and 100 ppm) were used for both modified biomasses.
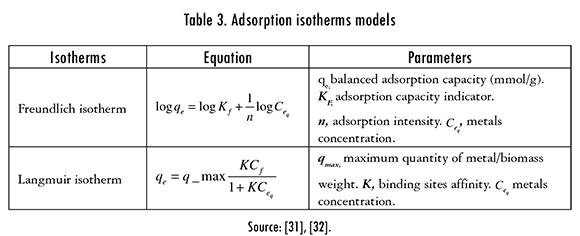
1.5. Hg (II) Determination
For the making of a 100 ppm Hg (II) standard solution, an analytical grade, Merck's brand mercury chloride (II) was used. The determination of the removed Hg (II) ions was carried out by a UV-Vis spectrophotometer, using ammonium thiocyanate as indicator. Additionally, a calibration curve was made, in which the detention limit of the technique for Hg (II) was determined [33].
2. Results and Discussion
For the characterization of adsorbent materials different analytic techniques were required in order to determine the chemical and elemental composition of cassava and lemon peels. The results obtained for each biomass are shown in Table 4. The element that presented the highest percentage in both biomasses was Carbon, 39.96% in cassava peels and 38.48% in lemon peels. Furthermore, it was also found that due to the high lignin, cellulose, and hemicellulose content in both adsorbents, high adsorption efficiency would be obtained because all of these polymers are well-known for its large quantities of hydroxyl, methoxyl, and carbonyl groups, which favor the adsorption of metals.
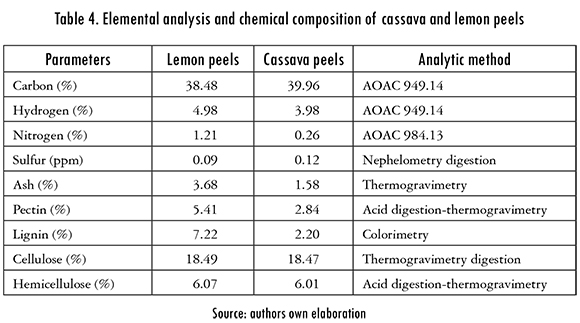
In the same way, the functional groups that are present in both biomasses and played a role in the adsorption of Hg (II) ions were identified by the Fourier transform infrared spectroscopy technique (FTIR). Figure 3 shows the infrared spectrum graphics before the adsorption process of Hg (II) ions for non-modified cassava peels (C. Yuca), cassava peels modified with citric acid (C. Yuca A.C), non-modified lemon peels (C. Limon), and lemon peels modified with citric acid (C. Limon A.C).
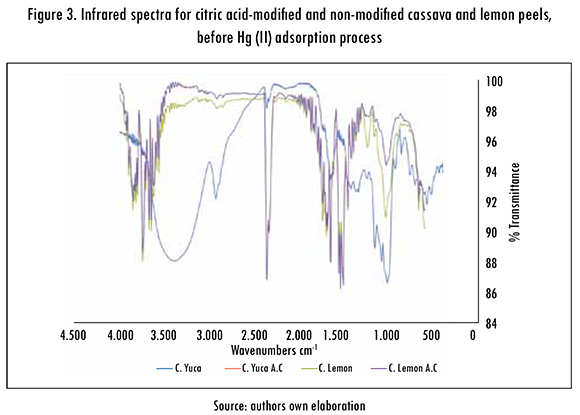
Very wide peaks can be observed in the spectrum, which indicates the complex nature of bioadsorbents. The wide and strong peaks between 33 900.28 and 3750.12 cm-1 indicate the stretching of the hydroxyl groups which, according to the literature, are found in 3300-3800 cm-1 range. The peaks between 1698.34 and 2000.13 cm-1 belong to carbonyl C = O stretching, which indicates the vibration of pectin, hemi-cellulose and lignin carboxyl groups in modified and non-modified cassava and lemon peels. The strong band that includes a range from 1233.54 cm-1 to 526.23 cm-1, belongs to the alcohol and carboxylic acid C-O group.
On the other hand, the infrared spectrum graphics after the adsorption process of Hg (II) ions for cassava peels modified with citric acid (C. Yuca A.C Hg) and lemon peels modified with citric acid (C. Lemon A.C Hg), can be observed in Figure 4. After the adsorption process of Hg (II) ions, the peaks for both modified biomasses decline slightly; this change in frequency can be attributed to the interaction of the molecules of Hg ions with the functional groups present in the surface of both biomasses. Nevertheless, greater intensity can be observed in peaks around 4000 cm-1, which corresponds to hydroxyl groups, and also a 2370 cm-1 peak that corresponds to C-H vibration. Higher intensity peak for carbonyl groups C = O can be observed in 1540 cm-1, and in 1000 cm-1 for the corresponding vibration of C-OH bond from a possible secondary alcohol, in this case. Thereby restating the involvement of hydroxyl group and carboxylic acid in the removal of the metal under investigation.
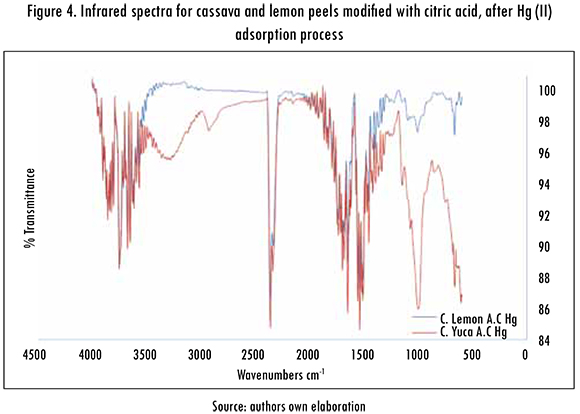
2.1. Adsorption Isotherms and Kinetics
The kinetics models that were reported in literature (Pseudo-first order, pseudo-second order, Elovich, and intraparticle diffusion), were adjusted to experimental data of the Hg (II) adsorption process using the tool named Microsoft Excel Solver. The statistical analysis of the results of the adsorption process of Hg (II) ions revealed that the maximum adsorption capacity of modified cassava peels was 72.98%, whereas lemon peels could remove 49.83%. Nevertheless, both biomasses have a similar adsorption rate of Hg (II) ions because they reach balance within the period between 240 and 330 min after the initiation of the process.
The adjustment of the kinetics models to the experimental data is shown in Figures 5 and 6. The kinetics models that better fitted the Hg (II) adsorption, using cassava and lemon peels modified with citric acid were Pseudo-second order and Elovich models; this means that the metal ion can be adsorbed by two active sites in the biomass. In addition, the mechanism that controls the adsorption is a second order reaction, the catalytic area of the bioadsorbent is heterogeneous, and shows different activation energies. In this model, a is related to chemisorption energy and |3 is related the covered surface. The obtained results of data fitting are shown in Table 5.
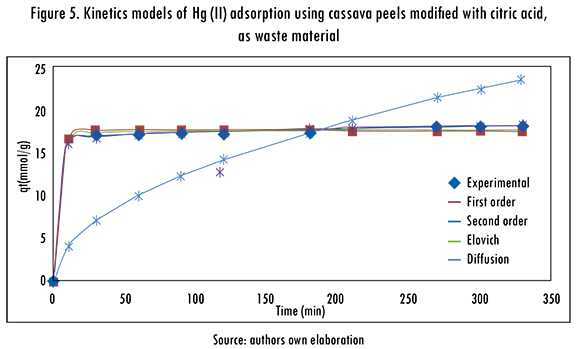
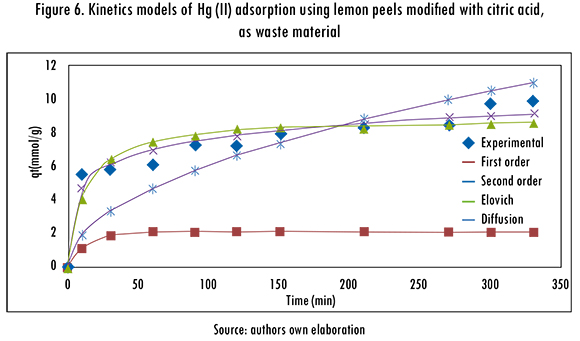
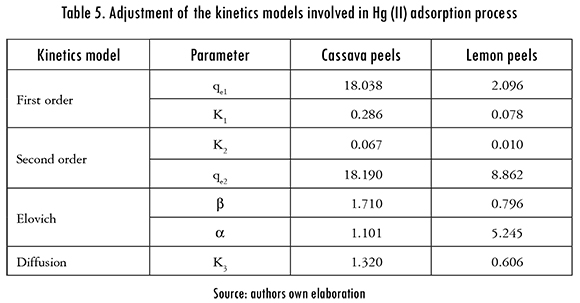
The adsorption isotherms of Langmuir and Freundlich were adjusted to the experimental data to evaluate the balance of the solute that was separated between the liquid and solid phase,. These models describe the energy distribution of the active sites, which can be homogeneous or heterogeneous, and also suggests monolayer or multilayer adsorption, with or without interaction between adsorbed molecules. The adjustment of Langmuir and Freundlinch models is registered in Figures 7 and 8. The calculated parameters are shown in Table 6.
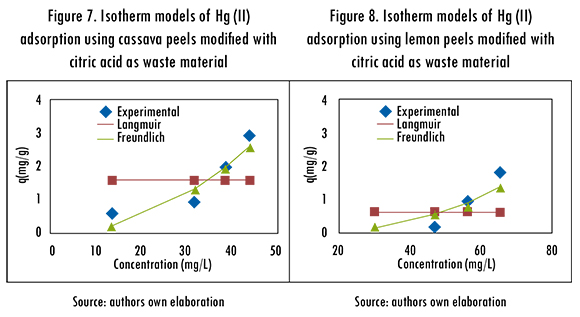
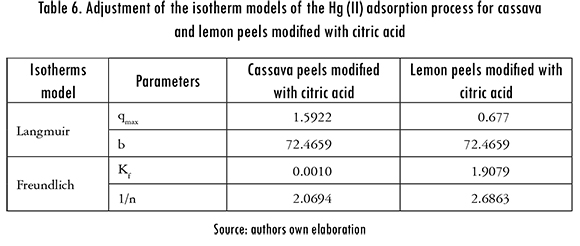
The isotherm models that better fitted the adsorption process of Hg (II) ions was Freundlich's. Consequently, it is established that during the adsorption process, multilayers are formed in the surface of the biomaterial; and the active sites of adsorption are first occupied by strong bonds and this strength decreases as the active sites are taken by Hg (II) ions.
From the results, it is established that cassava peels presents higher adsorption capacity than lemon peels. However, when comparing this to the information of the papers consulted [22-28], it can be found that the researched waste materials have a low adsorption capacity; and this is why studying the chemical modification of these materials is recommended in order to find some that would increase the availability of functional groups that can be capable to remove mercury.
Conclusion
According to the results obtained in this research, it was confirmed that cassava and lemon peels present a high potential for removing Hg (II) from synthetic water due to its chemical structure, which is largely made up of biopolymers as lignin, cellulose and hemi-cellulose. Likewise, the presence of hydroxyl and carbonyl groups in the surface of biomasses, which has great affinity to metal ions, was verified. However, it is recommended to study other chemical modifications that could increase the metal sorption, because the citric acid one does not show good results.
The adjustment of the obtained experimental data to the different chosen model indicates that Pseudo-second order and Elovich's are the ones that better fit the experimental data, describing the Hg (II) adsorption kinetics according to cassava and lemon peels modified with citric acid. On the other hand, the isotherm model that better fitted the obtained results was Freundlich's, according to which was restated that the adsorption process is controlled by a chemical reaction, as referenced in the consulted bibliography.
References
[1] O. Higuera, L. C. Flórez and J. V Arroyave, "Diseño de un biofiltro para reducir el índice de contaminación por cromo generado en las industrias del curtido de cueros", Dyna, vol. 76, no. 160, pp. 107-119, 2009. [ Links ]
[2] A. G. González and O. S. Pokrovsky, "Metal adsorption on mosses. Toward a universal adsorption model", Journal of Colloid and Interface Science, vol.415, pp. 169-178, 2014. [ Links ]
[3] A. Pérez, V Meseguer, O. Sáenz and M. Llorens, "Biosorption of chromium (III) by orange (Citrus cinensis) waste. Batch and continuous studies". Chemical Engineering Journal, vol. 155, pp. 199-206, 2009. [ Links ]
[4] L. Wang and C. Lin, "Adsorption of chromium (III) ion from aqueous solution using rice hull ash". Revista del Instituto Chino de Ingenieros Químicos, vol. 39, no. 4, pp. 367-373, 2008. [ Links ]
[5] J. Yang, C. Ling, L. Z. Liu, W L. Shi and X. Z. Meng, "Comprehensive risk assessment of heavy metals in lake sediment from public parks in Shanghai", Ecotoxicology and Environmental Safety, vol. 102, pp. 129-135, 2014. [ Links ]
[6] S. Luo, X. Li, L. Chen, J. Chen, Y. Wan and C. Liu, "Layer-by-layer strategy for adsorption capacity fattening of endophytic bacterial biomass for highly effective removal of heavy metals", Chemical Engineering Journal, vol. 239, pp. 312-321, 2014. [ Links ]
[7] M. A. Capó, Principios de ecotoxicología. Madrid: McGraw Hill, 2002. [ Links ]
[8] M. Santarder y P. Tapia, "Biosorción de iones cobre con biomasa de algas y orujos deshidratados", Rev. Metal. Madrid, vol. 45, pp. 365-374, 2011. [ Links ]
[9] M. Pinzón-Bedoya y A. Cardona, "Influencia del pH en la bioadsorción de Cr (III) sobre cáscara de naranja. Determinación de las condiciones de operación en proceso discontinuo", Revista de la Facultad de Ciencias Básicas, vol. 8, no. 1, pp. 45-53, 2010. [ Links ]
[10] F. Baldi, M. Gallo and D. Marchetto, "Seasonal mercury transformation and surficial sediment detoxification by bacteria of Marano and Grado lagoons", Estuarine Coastal Shelf Sci, 2012 [online]. Availabre: doi.org/10.1016/j.ecss.2012.02.008 [ Links ]
[11] A. Hutchison and D. Atwood, "Mercury pollution and remediation. The chemist's response to a global crisis",J. Chem. Crystal, vol. 33, pp. 631-645, 2003. [ Links ]
[12] O. Parkash, S. Lafferty and R. Meagher, "Biotechnological approaches for phytoremediation", in Plant Biotechnology and Agriculture, A. Altman and M. IP Hasegawa, Eds. Oxford: Academic Press, 2012, pp. 309-328. [ Links ]
[13] A. Le Jeune, F. Bourdiol and L Aldamman, "Factors affecting methyl mercury biomagnification by a widespread aquatic invertebrate predator, the phantom midge larvae Chaoborus", Environ Pollut, vol. 165, pp. 100-108, 2012. [ Links ]
[14] S. Pandey and S. B. Mishra, "Organic-inorganic hybrid of chitosan/organoclay bionano-composites for hexavalent chromium uptake", Journal Colloid Interface Science, vol. 361, pp. 509-520, 2011. [ Links ]
[15] L. Khezami and R. Capart, "Removal of chromium (VI) from aqueous solution by activated carbons. Kinetic and equilibrium studies", Journal of Hazard Material, vol. 123, pp. 223-231, 2005. [ Links ]
[16] R. M. Shrestha, I. Varga, J. Bajtai and M. Varga, "Design of surface functionalization of waste material originated charcoals by an optimized chemical carbonization for the purpose of heavy metal removal from industrial waste waters", Microchemical Journal, vol. 108, pp. 224-232, 2013. [ Links ]
[17] M. Torab-Mostaedi, M. Asadollahzadeh, A. Hemmati and A. Khosravi, "Equilibrium, kinetic, and thermodynamic studies for biosorption of cadmium and nickel on grapefruit peel", Journal of the Taiwan Institute of Chemical Engineers, vol. 44, pp. 295-302, 2013. [ Links ]
[18] M. Ajmal, R. Khan and J. Ahmad, "Adsorption studies on Citrus reticulate (fruit peel of orange). Removal and recovery of Ni (II) from electroplating wastewater", Journal of Hazardous Materials, vol. 79, pp. 117-131, 2000. [ Links ]
[19] C. Villanueva y N. Tapia, "Bioadsorción de Cu (II) por biomasas que contienen pectina", Revista Peruana de Química e Ingeniería Química, vol. 8, no. 1, 2005. [ Links ]
[20] F. J. Barragán, "Elaboración de un adsorbente de plomo y cadmio del agua a partir de los residuos de la industrialización del limón (Citrus aurantifoliaswingle)" [Tesis doctorado en ciencias de la F.C.B.A], Colima, México: Universidad de Colima, 2005. [ Links ]
[21] F. Colak, N. Atarb, D. Yazicioglu and A. Olgun, "Biosorption of lead from aqueous solutions by Bacillus strains possessing heavy-metal resistance", Chemical Engineering Journal, vol. 173, no. 2, pp. 422-428, 2011. [ Links ]
[22] Y Ho and C. Wang, "Sorption equilibrium of mercury onto ground-up tree fern", Journal of Hazardous Materials, vol. 156, pp. 398-404, 2008. [ Links ]
[23] I. Ghodbane and O. Hamdaoui, "Removal of mercury (II) from aqueous media using eucalyptus bark. Kinetic and equilibrium studies", Journal of Hazardous Materials, vol. 160, pp. 301-309, 2008. [ Links ]
[24] M. Lohani, A. Singh, D. Rupainwar and D. Dharc, "Studies on efficiency of guava (Psidiumguajava) bark as bioadsorbent for removal of Hg (II) from aqueous solutions", Journal of Hazardous Materials, vol. 159, pp. 626-629, 2008. [ Links ]
[25] S. Basha, Z. PV Murthy and B. Jha, "Sorption of Hg (II) onto Carica papaya. Experimental studies and design of batch sorber", Chemical Engineering Journal, vol. 147, pp. 226-234, 2009. [ Links ]
[26] C. Rocha, D. Morozin, R. da Silva, and A. da Silva, "Use of rice straw as biosorbent for removal of Cu(II), Zn(II), Cd(II) and Hg(II) ions in industrial effluents", Journal of Hazardous Materials, vol. 166, pp. 383-388, 2009. [ Links ]
[27] M. Madhava, D. Kumar, P. Venkateswarlu and K. Seshaiah, "Removal of mercury from aqueous solutions using activated carbon prepared from agricultural by-product/waste", Journal of Environmental Management, pp. 634-643, 2009. [ Links ]
[28] A. Neda, K. Tahereh and S. Mansooreh, "Elimination of mercury by adsorption onto activated carbon prepared from the biomass material", Journal of Industrial and Engineering Chemistry, vol. 18, pp. 283-289, 2012. [ Links ]
[29] H. B. Asberry, C.-Y. Kuo, C.-H. Gung, E. D. Conte and S. Y. Suen, "Characterization of water bamboo husk biosorbents and their application in heavy metal ion trapping", Microchemical Journal, vol. 113, pp. 59-63, 2014. [ Links ]
[30] S. Pitsari, E. Tsoufakis and M. Loizidou, "Enhanced lead adsorption by unbleached newspaper pulp modified", Chemical Engineering Journal, vol. 223, pp. 18-30, 2013. [ Links ]
[31] K. Y. Foo and B. H. Hameed, "Preparation, characterization and evaluation of adsorptive properties of orange peel based activated carbon via microwave induced K2CO3 activation", Bioresource Technology, vol. 104, pp. 679-686, 2011. [ Links ]
[32] A. Pérez, V Meseguer, O. Sáenz and M. Llorens, "Biosorption of chromium (III) by orange (Citrus cinensis) waste: Batch and continuous studies", Chemical Engineering Journal, vol. 155, pp. 199-206, 2009. [ Links ]
[33] R. S. Vieira and M. Marisa, "Dynamic and static adsorption and desorption of Hg (II) ions on chitosan membranes and spheres", Water Research, vol. 40, pp. 1726-1734, 2006. [ Links ]