1. Introduction
To reduce the effect of corrosion and wear on equipment exposed to chemically aggressive environments and subject to wear, the industry applies protective coatings on these elements. These coatings are hard layers of carbides, nitrides, borides and oxides. These coatings are deposited using techniques such as physical vapor deposition (PVD) and chemical vapor deposition (CVD). In PVD, the sputtering technique is used, and in the CVD, spray pyrolysis and thermo-reactive deposition/diffusion (TRD) techniques are often applied [1].
The advantage of TRD is that the deposited layers of dense and continuous carbide can be applied to steels containing carbon percentages higher than 0.3% [1]. TRD is also used for coating surfaces with hard materials in fluidized beds, molten salt baths, or powder. In addition, TRD emerges as a simple and economical alternative for the production of layers of binary carbides such as VC, NbC, TiC or Cr7C3, which are formed by the reaction between the carbon atoms of the substrate and the atoms of the carbide-forming elements (CFE). These elements are dissolved in molten borax and are added in powder form, such as ferro-niobium, ferro-vanadium, ferro-tungsten, ferro-titanium, ferro-chrome and chrome [1], [2]. The use of carbide coatings on steels is due to their physical and chemical properties such as high surface hardness, excellent adhesion, and resistance to wear and corrosion [1]. Because the layer growth depends on the diffusion of carbon, the process requires relatively high temperatures ranging from 800 to 1250 °C to maintain an adequate deposition rate. As shown in [3] and [4], the carbide coating thicknesses ranging from 4 to 15 microns are obtained in time periods between 10 minutes and 8 hours, depending on the bath temperature and the type of steel. Nb is an excellent CFE because it has a lower free energy of carbide formation and its free energy of formation of oxides is higher than that of B2O3 [5], [6].
Niobium carbide (NbC) is a chemically stable compound with a cubic crystalline structure; it melts at a high temperature and has a high hardness. These properties make NbC potentially useful as a protective coating against corrosion. However, the characterization of the corrosion resistance of NbC has not been extensively explored. In particular, there are very few studies related to the corrosion resistance of the carbides obtained by TRD [7], [8]. The corrosion resistances of carbides, nitrides and borides produced using the PVD technique mainly have been studied. The wear resistance and hardness of layers containing carbides obtained by TRD have been analyzed [9]-[12], and those layers have been reported to have good tribological properties. X. S. Fan et al. [13], [14] recently studied the growth mechanism and microstructure of carbides prepared using TRD, analyzed the effect of the carbon activity in the growth of these coatings on AISI H13 and AISI 9Cr18 steel, and developed a mathematical model to explain this effect. The aim of the present work is to analyze the corrosion resistance of niobium carbide coatings grown on AISI D2 and AISI H13 steels using a TRD system with electrochemical techniques
2. Experimental techniques
2.1. Preparation of the substrates
NbC coatings were deposited onto substrates of AISI H13 and AISI D2 tool steel. These substrates were prepared with dimensions of 16 mm in diameter and 3 mm in thickness and were subsequently polished with 600 grit sandpaper and washed in an acetone solution for five minutes in an ultrasound device. The hardness values of the annealed AISI D2 and the annealed AISI H13 were 223 HB and 200 HB, respectively. Table 1 lists the chemical composition of the substrates.
2.2. Growth of the coatings
The temperature conditions and TRD treatment time were 1050 °C and 4 hours, respectively. The reaction medium in the process is formed by 4 wt% aluminum and 20 wt% ferroniobium concentrations combined with a percentage of borax pentahydrate (Na2B4O7 • 5H2O) to complete the mixture. The samples were quenched in water without agitation. The time and temperature conditions ensured the complete austenitization of the steel used in this study without excessive distortion or abnormal grain growth. Samples of niobium carbide obtained on AISI D2 and AISI H13 steels will be called D2-NbC and H13-NbC, respectively.
2.3. Chemical and microstructural characterization
The chemical composition of the layers was evaluated using a MagixPro Philips PW-2440 XRF spectrometer, equipped with a rhodium tube of 4 kW maximum power. This device has a sensitivity of 200 ppm (0.02%) for heavy metals. Semi-quantitative analysis was performed using the IQ software. The specimens were ultrasonically washed in an acetone solution for 5 minutes before each measurement.
The microstructure of the coatings was determined with a PANalytical X’Pert PRO diffractometer using Bragg Brentano geometry with Cu Ka radiation (k=1.5405 A) between 10° and 60° with steps of 0.02°, a current of 40 mA and a potential difference of 45 kV. The sweep range was 20° to 90°. Analysis of the morphology and thickness of the coatings was performed using an FEI Quanta 200 electron microscope. The images were obtained using secondary and backscattered electrons accelerated at an energy of 20 kV. In the analysis of the cross section, transverse samples were cut. The samples were polished to a mirror finish and were attacked with Villela’s solution to reveal their surface microstructure.
2.4. Corrosion resistance analysis
To analyze the behavior of the coated and uncoated substrates in the corrosion process, polarization Tafel tests and electrochemical impedance spectroscopy (EIS) were performed. The Tafel tests were performed in an AC Gill potentiostat manufactured by ACM Instruments, and the EIS tests were performed on a Gamry Instruments potentiostat-galvanostat reference mark 600. The two tests followed the procedures described in ASTM G5 and ASTM G52, respectively [12]. The experimental conditions for the development of the polarization Tafel analysis were as follows: a solution of deionized water to 3% NaCl at room temperature; initial potential (Ei): -0.3 V; final potential (Ef): 0.5 V; scan speed: 20 (mV/min); and 0.196 cm2 of exposed area of the sample in the solution. The EIS tests were performed in the same solution with the following conditions: initial frequency of 100 kHz; final frequency of 0.01 Hz; and AC voltage of 10 mV.
The EIS measurements were conducted for 1, 24, 48, 72 and 168 hours. In all of the tests, the solution was stabilized for 45 minutes after the immersion of the sample in the electrochemical cell, which comprised a graphite counter electrode, a reference saturated calomel electrode (SCE) and a working electrode formed by the sample under test, all immersed in 250 ml of solution. The Tafel polarization curves that were obtained were analyzed using Sequencer software version 5, and the graphs obtained by EIS were analyzed using the Gamry Echem Analyst software, modeled on an equivalent electrical circuit for a porous coating.
3. Results and discussion
3.1. Chemical composition
Table 2 presents the XRF results, which reveal the niobium percentages in the coatings. In the chemical analysis, trace amounts of sodium and aluminum were also observed and appear to be a residue from the borax, which is used as the reaction medium.
3.2. X-ray diffraction
Figure 1 presents the X-ray diffraction (XRD) results of the niobium carbide coatings grown on AISI H13 and AISI D2 steels. The XRD analysis reveals that the niobium carbide was polycrystalline with a face-centered cubic (FCC) structure, of type NaCl, in which the niobium atoms occupy the FCC phase lattice and the carbon atoms occupy the interstitial positions between the niobium atoms [15] (PDF card 00-010-0181) on both substrates. These results agree with those obtained by S. Sen and U. Sen (2008) [11]; Oliveira etal. (2006) [12]; Castillejo et al. (2013, 2011) [7], [16]; and Orjuela et al. (2014) [8]. Figure 1 also shows the planes belonging to the substrates.
3.3. Morphology and thickness
Figure 2 presents cross-sectional SEM images of the niobium carbide layers obtained on AISI D2 and AISI H13. In general, these coatings have a compact and homogeneous morphology and they show a high adherence to the substrate surface [7].
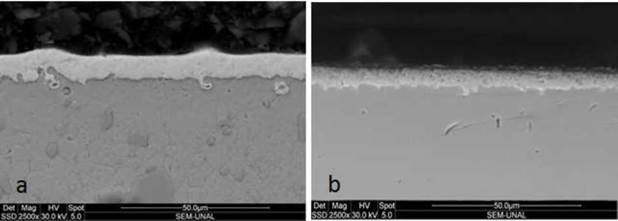
Source: Authors’ own creation
Figure 2 Transverse section SEM images of niobium carbide coatings deposited using TRD on a) AISI D2 and b) AISI H13.
The thicknesses obtained from the SEM micrographs were 13.0 ± 0.6 pm on AISI D2 and 9.5 ± 0.5 pm on AISI H13. The greater thickness of the layers obtained on AISI D2 is due to the growth coefficient Ko of steel: 82 for D2 and 0.0345 for H13, according to the Arrhenius equation (see equation 1).
where d is the layer thickness (cm), t is time (s), Q is the activation energy, T is the absolute temperature (K), R is the gas constant (8.314 J/mol K), K is the growth rate (cm2/s), and Ko is a growth coefficient. The growth coefficient increases with the carbon content of the substrate [17], that is, with increasing carbon activity (Ac) in the substrate and the coating. The carbon activities in the D2 and H13 steels are approximately 0.33 and 0.11, respectively, at 1000 °C [11], [12].
3.4. Corrosion resistance
Table 3 presents the average results of the corrosion potential and corrosion current density for the layers obtained for each of the steels with the corresponding standard deviation. Although the corrosion current density in the coated D2 steel substrate was similar to that obtained in the uncoated steel, the corrosion voltage was higher in the coated steel, thus showing an improvement in the electrochemical protection of the system. On the other hand, the coating of niobium carbide on H13 steel showed greater resistance to corrosion compared to uncoated steel. When comparing the coatings on the two steels, a greater corrosion resistance was observed in the coated D2 steel, since it presents a more positive value of the corrosion voltage compared to the coating on steel H13, which is most likely due to the percentage of porosity in the coatings [16]. In general, the niobium carbide coatings tend to improve the corrosion resistance of the substrate-coating system because the corrosion potential has a higher value compared with the uncoated steel; this effect is likely due to the formation of niobium oxide passivation layers on the coating surface [7], [8].
Table 3 Tafel polarization results of NbC coatings deposits on AISI D2 and AISI HI3 steel
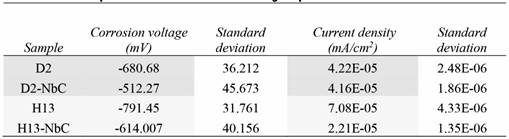
Source: Authors’ own creation
3.4.1. Electrochemical impedance spectroscopy
Figures 3 and 4, which were obtained using EIS, reveal that the niobium carbide coatings on both steels tend to increase the phase angle at low frequencies when the time of immersion in the electrolyte increases. In addition, these figures also reveal a shift of the peaks of the phase angle toward a lower frequency. This demonstrates that the coating enhances the corrosion resistance of the system due to the increased capacitive characteristics of the coating caused by the passivation process at low frequencies [18]-[20]. Therefore, the niobium carbide layer increased the ability to create a passivation layer, in this case, niobium oxide. These results differ from those obtained on uncoated steels at low frequencies because the phase angle tends to be 15° on both substrates, and the impedance decreases to 10,000 Q (D2 steel) and 20,000 Q (H13 steel) for an immersion time of 168 h. These results suggest that the steels are attacked by the electrolyte, and therefore, the dissolution of corrosion products in the electrolyte increased with the time of exposure.
To model the electrochemical behavior of the coatings, we used an equivalent circuit (EC), which accounts for corrosion processes both in the coating-electrolyte system and the coating-substrate system and therefore uses constant phase elements (CPE) to simulate the phenomenon of corrosion in the two systems.
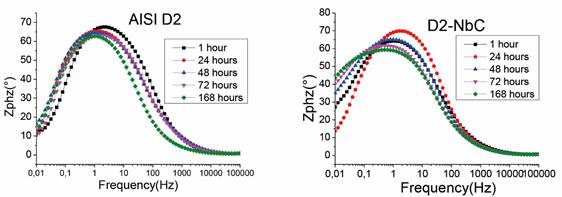
Source: Authors’ own creation
Figure 3 Bode diagrams of (a) uncoated AISI D2 and (b) AISI D2 coated with NbC
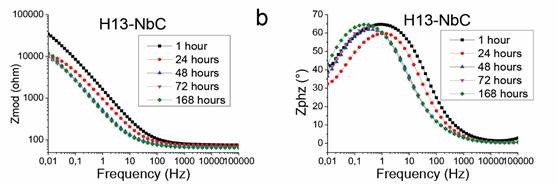
Source: Authors’ own creation
Figure 4 Bode diagrams of (a) uncoated AISI H13 and (b) AISI H13 coated with NbC
In the coating-substrate system, the corrosion processes are galvanic and are produced due to the presence of defects such as pores and porous vacancies in the coating. Therefore, corrosion processes occur in the coating and at the coating-substrate interface through these defects. These results agree with those obtained by the authors in previous studies [8]. The electrochemical process that occurs in the interface can thus be simulated by the equivalent circuit illustrated in Figure 5[8]. The circuit is formed by RC elements connected in series and in parallel; elements Rpore and CPE1 are equivalent to the coating that protects the metal [8], [21]. The resistor Rct and the capacitor CPE2 are equivalent to the dielectric double layer formed at the electrolyte-substrate interphase [22], [23]. The circuit is also formed by the resistor Rs, which is the resistance of the solution and reference electrode RE and the working electrode WE. The values obtained for each of these elements during the simulation are presented in Table 4.
The results indicate that the solution resistance (Rs) of the niobium carbide coatings deposited on H13 steel tends to decrease as a function of immersion time, which is due to an increase in corrosion products. This increase means that there is a chemical reaction between the coating and the electrolyte, i.e., the coating loses its corrosion protective characteristic. In D2 steel, the Rs value is higher because the surface of the coating is less porous [7], [23], as evidenced by the Rpore values for each coating. The charge transfer resistance (RTC) between the electrolyte and the substrate has the highest value and increases with immersion time (in D2 steel), which implies that the double dielectric layer formed between the electrolyte and substrate appears to be the principal cause of the increased corrosion resistance of the coatings deposited on the substrates. The RTC values of the coating deposited on H13 steel are smaller than those deposited on D5 steel and do not change with time during the EIS test. These charge transfer resistance values are explained due to the properties of the ceramic coating and the large amount of chromium in D2 and H13 steels. Due to a diffusion process that is produced in treating TRD, chromium can be precipitated onto the surface and form a passive oxide layer.
The adjustment parameters m and n correspond to the constant phase elements CPE1 and CPE2, respectively. An ideal capacitor has m and n values of 1, and a leaky capacitor, pores or defects when its value ranges are represented by values between 0.5 and 1 [24]. The values of m and n obtained can determine whether the coatings tend to behave like a leaky capacitor, pores or defects because its value varies between 0.59 and 0.84, as shown in [21], [23] and [25], denoting a high roughness of both the interface between the coating and the substrate [25], [26].
Figure 6 presents the polarization resistance results (Rp = Rs + Rpore + Rtc) for the coatings deposited on the two steels studied; the Rp of the uncoated substrates is shown for reference. These results establish that the niobium carbide coatings deposited on AISI D2 steel improved the polarization resistance by a factor of four after 168 hours of immersion in the electrolyte.
4. Conclusions
In this work, niobium carbide coatings were prepared on AISI D2 and AISI H13 substrates using a deposition-diffusion thermoreactive technique. A greater thickness in the coatings deposited on the D2 steel was observed due to this substrate having higher carbon activity and growth coefficient values compared with those of the H13 steel.
The analysis of corrosion resistance using the EIS technique revealed that the coatings prepared on the D2 steel have a higher corrosion resistance because they most likely have fewer pores and imperfections on the surface, which causes the coating to exhibit dielectric behavior. Furthermore, the carbon activity and the amount of chromium present on the substrate appear to produce chromium oxides, which act as passivation films at the substrate-coating interface.
Finally, it was established that the niobium carbide coatings deposited on the AISI D2 steel have a polarization resistance that is four times larger than that of uncoated steel, thus establishing the anticorrosive effect exhibited by the coating.