Serviços Personalizados
Journal
Artigo
Indicadores
-
Citado por SciELO
-
Acessos
Links relacionados
-
Citado por Google
-
Similares em SciELO
-
Similares em Google
Compartilhar
Ingeniería y competitividad
versão impressa ISSN 0123-3033
Ing. compet. vol.15 no.2 Cali jul./dez. 2013
Treatment of vinasse by using electro-dissolution and chemical flocculation
Tratamiento de vinaza empleando electrodisolución y floculación química
Charles Cardona
Chemical Engineering School. Universidad del Valle. Cali, Colombia
E-mail: charles-24@hotmail.com
Fiderman Machuca-Martínez
Chemical Engineering School. Universidad del Valle. Cali, Colombia
E-mail: fiderman.machuca@correounivalle.edu.co
Nilson Marriaga-Cabrales
Chemical Engineering School. Universidad del Valle. Cali, Colombia
E-mail: nilson.marriaga@correounivalle.edu.co
Eje temático: Chemical engineering / Ingeniería química
Recibido: 19 de Abril de 2013
Aceptado: 21 de Junio de 2013
Abstract
Vinasse is the most important waste from the bioethanol plants due to its high polluting potential, for this it has received special attention because of interest to develop new treatment alternatives. It was evaluated the removal capacity of solids content and other parameters of vinasse by using electro-dissolution of iron and chemical flocculation through separate stages. The appropriate operating conditions for electro-dissolution were established by means of linear polarization sweeps and electrochemical impedance spectroscopy analyses. During the flocculation were conducted jar tests by using Ca(OH)2 as coadjuvant agent and as pH modifier. In this way, was possible to diminish 84% total solids, 54% chemical oxygen demand, 88% dissolved organic carbon and 76% color. The electrical consumption during electro-dissolution was 0.25 kWh/m3.
Keywords: Electrocoagulation, electrodissolution, flocculation, vinasse.
Resumen
La vinaza es el residuo más importante de las plantas de bioetanol debido a su alto potencial contaminante, por esto ha recibido gran atención debido al interés de desarrollar nuevas alternativas de tratamiento. Se evaluó la capacidad de reducción del contenido de sólidos y otros parámetros de la vinaza empleando electrodisolución de hierro y floculación química a través de etapas separadas. Las condiciones de operación adecuadas para llevar a cabo la electrodisolución de hierro se establecieron a través de barridos de polarización lineal y análisis de espectroscopia de impedancia electroquímica. Durante la floculación se llevaron a cabo pruebas de jarras empleando Ca(OH)2 como modificador de pH y como agente coadyuvante. De esta manera fue posible disminuir 84% los sólidos totales, 54% la demanda química de oxígeno, 88% el carbono orgánico disuelto, y 76% el color. El consumo eléctrico durante la electrodisolución fue 0.25 kWh/m3.
Palabras claves: Electrocoagulación, electrodisolución, floculación, vinaza.
1. Introduction
During years it has been studied numerous ways to treat vinasse from ethanol distillery. Previous studies (Pant & Adholeya, 2007; Satyawali & Balakrishnan, 2008) have considered that anaerobic treatments are most attractive for pollutants removal of vinasse because energy can be recovered from biogas. However, anaerobic treatments do not degrade melanoidins which are responsible for brown color of the vinasse and also they are persistent compounds (Chandra, et al. 2008; Liang, et al. 2009). This has led to study the combination of anaerobic treatments with advanced oxidation processes (Martín, et al. 2002) or by using bio-concentration processes (Navarro, et al. 2000)
In addition, It have been studied other physicochemical treatments as adsorption, coagulation, electrochemical oxidation and membranes technologies; in spite of this, an efficient technology that can be used for treatment of vinasse has not been found yet (Mohana, et al. 2009). Chemical coagulation by using inorganic salts, such as FeCl3∙6H2O or Al2(SO4)3∙16H2O, have proven to be effective for color removal of vinasse biologically pretreated (VBP) but the coagulant consumption is actually high (Chaudhari, et al. 2007). Diluted vinasse with 6,000 ppm of chemical oxygen demand (COD) has also been treated by electro-oxidation in presence of sodium chloride (15,000 ppm), achieving diminish 99% of COD (Vijayaraghavan, et al. 1999) but with a high electricity consumption, around of 30 kWh/m3. Yavuz (2007) assessed the electro-fenton technique applied to VBP (4,985 ppm of COD) and achieved 93% of COD removal; likewise, Dávila, et al. (2011) also studied the electrocoagulation-electroflotation of raw vinasse without dilution (160,000 ppm of COD) and they achieved 61% of COD removal by using up to 60,000 ppm of H2O2.
On the other hand, the electrocoagulation has shown some advantages over conventional wastewater treatments such as its low cost and its simple operation, for these reasons, applications have increased in recent years. Effluents containing heavy metals, food waste, waste oil, organic matter from landfill leachates, phenols, nitrates, sulfates, fluoride, arsenic, chromium and organic compounds non-biodegradable, have been treated with this technology (Emamjomeh & Sivakumar , 2009).
Therefore, the electrocoagulation has become interesting alternative for VBP treatment; Kumar et al. (2009) achieved 50% of COD removal in VBP from an initial content of 15,600 ppm and also 92% of color removal by using iron anodes during electrocoagulation. Thakur, et al. (2009) achieved 61% of COD removal from an initial content of 9,300 ppm and also 98% of color removal by using stainless steel anodes. In another study, VBT with 4,500 ppm of COD was subjected to chemical flocculation and it was achieved 80% of COD removal (Ryan, et al. 2008). Here was reported that treatment of 1,000 m3/day of VBP would require 6 ton/day of conventional coagulant, whereas through the electrocoagulation it would take only 300 kg/ day of iron. Likewise, VBP (3,950 ppm COD) was treated by electrocoagulation using iron and aluminum electrodes and were achieved reductions of 99% color and 80% COD (Gadd, et al. 2010).
In spite of this, even exists few studies of electrocoagulation applied to untreated vinasse (raw vinasse); nevertheless, the electrocoagulation has been employed for decolorizing raw vinasse (diluted up to 17.5%) using iron anodes (Prasad, et al. 2008). They were found out that at current density of 31 mA cm-2and 31,500 coulombs/liter could be reduced 93.5% the color; unfortunately they did not shown the characteristics of vinasse studied. Also, this technique was applied to diluted vinasse (14,500 ppm of COD) by using aluminum electrodes and additionally it was supplied activated carbon (40,000 ppm of COD); thus, it was achieved to diminish 80% COD and 99% turbidity (Kannan, et al. 2006). Recently, electrocoagulation was used simultaneously with ozone in diluted raw vinasse (2,500 ppm COD) and was achieved complete discoloration and 83% reduction in COD (Asaithambi, et al. 2012). The ozone dosage was 15 L min-1 for two hours of treatment and required power consumption in the electrocoagulation was 5.1 kWh m-3 Electrocoagulation is a process based on the electrolytic dissolution of a metal as iron or aluminum and in situ production of insoluble amorphous species. However, this method has severe limitations when the suspension to be treated has high buffer-capacity, like the raw vinasse, due to it is increased the electrical energy consumption. The purpose of this study was to evaluate the removal of total solids content in untreated vinasse by combining iron electrodissolution and chemical-flocculation.
2. Matherials and methods
2.1 Iron-electrodissolution
The lab-scale cell consisted of a plexiglas recipient with volume of 1,000 mL and flat electrodes (monopolar configuration) with six 316 stainless steel cathodes and five anodes of carbon-steel (ASTM-A-36). The electrodes had dimensions of 9×6 cm with 3 mm gap among them and total anodic area was 900 cm2. The raw vinasse was recycled through a centrifugal pump which supplied an upward flow of 1.9 L min-1. Direct current was supplied by an Extech® source of 5A, which was used in galvanostatic mode. Before testing the electrodes were subjected to dry abrasion with abrasive paper No. 500 and then with abrasive paper No. 1,200 to get a shiny appearance. Afterward, the electrodes were rinsed with distilled water and immersed in acetone for about ten minutes to remove any traces of oil or grease. Finally they were rinsed with distilled water before use them in electrodissolution.
Preliminary tests. The current density magnitude in the lab-scale cell was established by recording the linear polarization resistance in a three electrodes cell assembly by using a Gamry potentiostat PC4-750. The effects of pH and the current density during the electrodissolution were evaluated by supplying the same specific electrical charges (15,000 C L-1). The solution resistance behavior, the charge transfer resistance and double-layer capacitance during the iron electrodissolution process were established by using Electrochemical Impedance Spectroscopy (EIS). Three EIS spectra were taken at 30 minutes intervals, keeping the current density (J) at 0.3 mA cm-2
Final tests. The experiments were conducted by varying the theoretical dosage of iron (through of the specific electric charge magnitude supplied) and by setting the initial pH at 4.5 and current density at 0.3 mA/cm2. The parameter Rc was defined to relate the theoretical amount of iron supplied during the electrodissolution and the solids content of the vinasse, then was calculated by Eq. (1).

where i represents the current supplied in amperes, t is time in seconds, and St represents the total solids concentration (%) in the treated vinasse. Also, it was studied the influence of three Rcvalues: 1,000; 1,500 and 2,000 during the electrodissolution stage and its effect on the flocculation stage. Under these considerations and for comparison effects, the iron content in the solution was measured by atomic absorption and the physiochemical characteristics of samples were determined according the standard methods (AHPA, 1998). For all tests were established the power consumption, W in kWh m-3, through the Eq. 2.

where v represents the voltage supplied in volts and vol is the volume of sample in liters.
2.2 Chemical flocculation
The vinasse samples were taken after going through the electrodissolution stage (Rc1,500) and then diluted with different ratios by using distilled water. The jar tests were carried out with rapid stirring (500 rpm) during 10 seconds and then slow agitation (70 rpm) for 10 minutes, using a slurry of Ca(OH)2 (7% w/w) as pH modifier and coagulation adjuvant agent. For all tests, the residual turbidity in the supernatant was recorded with a Hach-2000 turbidity meter and removal of solids was registered by evaporation of the samples at 105 °C by using a Radwag thermoanalytical balance.
For conditions which highest turbidity removal was obtained (Rc 1,500 and St 10%), subsequent flocculations were performed (pH=11.5) using supernatant instead of distilled water for dilution. For this reason, were assessed all parameters of interest carrying out three continuous recirculation of supernatant. The obtained sludge was lyophilized (-80 °C and 3 Pa) with a FDU2100 Eyela lyophilizer, and scanning electron micrographs were recorded with a JEOL (JSM6490LV) equipment.
3. Results and discussion
The characteristic parameters of the raw vinasse were: 40,000 ppm of dissolved organic carbon (DOC); 125,000 ppm of COD; 10.4% w/w of total solids (TS); pH of 4.6; turbidity of 2,140 NTU and electrical conductivity of 20.1 mS/cm.
3.1 Electrochemical analysis
Important information was obtained from the study of the iron-electrodissolution process and the electrode passivation in the electrolytic reactor through of techniques as linear anodic polarization and electrochemical impedance spectroscopy.
Linear anodic polarization. The electrodissolution of Fe2+ showed faradic behavior (Figure 1) up to 13.5 mA cm-2(540 mV vs Ag/AgCl) where was observed onset a passivation phenomenon. Gadd, et al. (2010) studied electrocoagulation of biologically treated vinasse and they found out the formation of localized pits on the anode surface due to presence of chloride ions that would lead to an aggressive corrosion environment but there was no evidence of anodic protection at current densities below 5 mA cm-2. Possibly the Fe2+ formed insoluble species on the anode surface causing a sudden increase in the electrode voltage; for that reason, the system should be operated at lower current densities. Moreover, Mansouri, et al. (2011) found out that the presence of phosphate ions inhibits the corrosion of iron by formation of a passive layer of iron hydroxide/phosphate on anode surface. It is known that the vinasse has high content of chloride ions and phosphate ions; therefore, this could be related with the behavior found here.
Electrochemical impedance spectroscopy (EIS). The linear polarization does not demonstrate the electrode behavior with time whilst EIS provides information related with reaction mechanism. Nyquist diagrams revealed three arcs with increasing diameters when iron-electrodissolution took place (Figure 2); therefore, the transient behavior of the anode can be described by a Randles circuit. This would indicate that only appeared an active-adsorbed specie on anode surface during the electrodissolution (Maddala, et al. 2010). In acidic solutions the reaction mechanism of the iron dissolution considered a non-catalytic path (Vukovic, 1996):

Determining step involves the dissolution of an active complex adsorbed on the metal surface:

The complex ions FeOH+ are produced easily and are converted in Fe2+ ions:

The values obtained fitting the parameters of the Randles circuit with the Nyquist diagrams are shown in Table 1. This results indicate that the charge transfer resistance (Rct) of the anodevinasse interface increased by 65% during the first 30 minutes and then 21% additional during the remaining 30 minutes. This would suggest the formation of insoluble species on the electrode surface during the first hour of operation. The Fe2+ reacted with OH¯ ions generated at the cathode to form insoluble hydroxides [Fe(H2O)5(OH)2], this layer diminished the fraction of area occupied for active complex adsorbed species that contribute with the electrodissolution (eq. 3); therefore was increased Rct (Ma, et al. 1998).
3.2 Iron-electrodissolution
Effect of pH and current density (J). During the electrodissolution the pH of the vinasse was increased but this effect was less noticeable when J decreased (Figure 3). The pH did not show significant changes when was used low current density (0.3 mA cm-2), although for initial pH greater to 4.5 was observed an increase to 35% at the final pH when J = 13 mA cm-2.
The H2 production rate at the cathode reaction diminish when J decreases, this is equivalent to a reduction of the OH¯ production rate. In addition, the formation of complex-iron ions due to hydrolysis consumes OH¯ ions; hence, the interaction of these phenomena would explain why the pH at low current densities was not increased. Sasson, et al. (2009) showed that at low current densities and low pH, the iron-electrodissolution was favored due to contribution simultaneous of the chemical corrosion.
Figure 4 shows the behavior of the lab-cell voltage and the pH after 4 hours of operation for two values of J. When it was applied the lower J value, the cell voltage and the pH were kept constant; conversely, these two variables were increased when it was operated at 0.8 mA cm-2. The increase in the voltage was particularly high (80 mV to 1,200 mV) in the last two hours of operation. This can be attributed to the presence of insoluble iron oxy-hydroxides attached to the electrode surface causing its partial passivation. The presence of these insoluble species at a pH above 5.5 can cause inefficient metal electrodissolution (Linares-Hernández, et al. 2009; Cañizares, et al. 2009).
Effect of Rc. Figure 5 shows the behavior of the reducing turbidity (% RT) and reducing total solids (% RS) when was varied Rc during subsequent flocculation tests. The lowest turbidity removal was achieved when Rc=1,000 which would indicate that the applied electric charge was not sufficient to obtain the necessary concentration of iron cations.
For Rc greater or equal to 1,500 and to the extent that the content of solids in the vinasse increased, the turbidity removal and solids removal were improved. The possible cause is related to the destabilization of colloids by incorporating a chemical bridge due to the increased concentration of ions as HPO4-2, Ca2+, Fe2+ y Mg2+ contents in the vinasse (Voegelin, et al. 2010).
C urrent efficiency. By comparing the theoretical amount of Fe2+ (4,000 ppm calculated by using Faraday's law) to the registered total iron by atomic absorption (1,400 ppm) only was possible achieve an efficiency of 35%. This low efficiency was attributed to the formation of insoluble species adsorbed on anodes; despite this, the power consumption was low just 0.25 kWh m-3.
3.3 Chemical flocculation
Figure 6 shows the behavior of the residual turbidity and lime consumption in the range of pH tested for flocculation. As the pH increased in flocculation, the residual turbidity in the supernatant was reduced achieving a maximum reduction at pH of 11.5.
It has been shown (Semerjian & Ayoub, 2003) that the process of clarification of wastewaters with high content of Mg2+, Ca2+, CO32- , and HPO43, like vinasse, is strongly favored at pH greater to 11 due to precipitation of insoluble salts. The following equations show the possible reactions of different ions in the solution during the flocculation process.
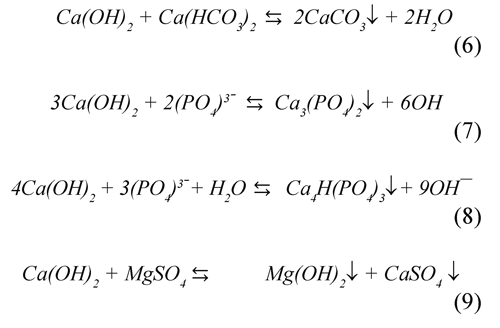
Also, the chemical equilibrium of ferrous species in aqueous solutions (Figure 7) reveals that at pH greater to 11 the Fe(OH)2achieves the saturation as a precipitated amorphous solid (Morgan & Lahav, 2007). Figure 8 shows the characteristic porosity of the settled solids obtained during flocculation, this would have relation with phenomena of aggregation of amorphous species of ferrous-hydroxide and of slaked lime colloidal. Conversely, the lime consumption was significantly increased at a pH greater than 10.5, due to possibly to the loss of solubility of calcium hydroxide.
3.4 Supernatant recirculation
The lime consumption was reduced by decreasing from 0.51 to 0.28 g lime/g solids, representing a decrease of 45% compared to the test without recirculation. The total solids removal was from 63% up to 84%. In addition, reductions of 88% TOC, 76% of color (Pt/Co scale) and 54% of COD were achieved. Finally, it was established that the total iron content in the supernatant was 28 ppm, meaning that about 98% of the iron was precipitated in the sludge.
4. Conclusions
By coupling iron electrodissolution and chemical flocculation processes, a reduction of 84% of total solids content, 54% of chemical oxygen demand, 88% of dissolved carbon content and 76% of color were achieved in raw vinasse with low power consumption (0.25 kWh/m3). The best results were achieved when the process of electrodissolution was performed with 0.3 mA/cm2 and an Rc of 1,500. This current density is within the working range found by linear polarization tests and EIS. Flocculation tests showed that the most appropriate condition was obtained at pH = 11.5 using Ca(OH)2 as a coagulation adjuvant and as a pH modifier. It was verified that it was favorable to use the supernatant as dilution water and thus the total solid, TOC and COD removal was increased.
5. Acknowledgments
The authors acknowledgments to the Universidad del Valle and COLCIENCIAS for the grant No. 110652128599. TRATAMIENTO COMBINADO DE VINAZAS DE DESTILERIA: ELECTRODISOLUCION, FLOCULACION Y OXIDACION (ELECTROFLOX-UV)
6. References
American Public Health Association (AHPA). (1998). Standard Methods for the Examination of Water and Wastewater (20 ed). Washington, DC. [ Links ]
Asaithambi, P., Susree, M. & Saravanathamiz, R. (2012). Ozone assisted electrocoagulation for the treatment of distillery effluent. Desalination , 297, 1-7. [ Links ]
Cañizares, P., Jiménez, C., Martínez, F., Rodrigo, M.A., & Sáez, C. (2009). The pH as a key parameter in the choice between coagulation and electrocoagulation for the treatment of wastewaters. Journal of Hazardous Materials , 163 (1), 158-164. [ Links ]
Chandra, R., Bharagava, R., & Rai, V. (2008). Melanoidins as major colourant in sugarcane molasses based distillery effluent and its degradation. Bioresource Technology , 99, 4648-4660. [ Links ]
Chaudhari, P., Mishra, I., & Chand, S. (2007). Decolourization and removal of chemical oxygen demand (COD) with energy recovery: Treatment of biodigester effluent of a molasses-based alcohol distillery using inorganic coagulants. Colloids and Surfaces A: Physicochem. Eng. Aspects. , 296, 238-247. [ Links ]
Dávila, J., Machuca, F., & Marriaga, N. (2011). Treatment of vinasses by electrocoagulation- electroflotation using the Taguchi method. Electrochimica Acta , 56, 7433-7436. [ Links ]
Emamjomeh, M. & Sivakumar, M. (2009). Review of pollutants removed by electrocoagulation and electrocoagulation/flotation processes. Journal of Environmental Management , 90, 1663-1679. [ Links ]
Gadd, A., Ryan, D., Kavanagh, J., Beaurain, A., Luxem, S., & Barton, G. (2010). Electrocoagulation of fermentation wastewater by low carbon steel (Fe) and 5005 aluminium (Al) electrodes. Journal of Applied Electrochemistry , 40 (8), 1511-1517. [ Links ]
Kannan, K., Karthikeyan, G., & Tamilselvan, N. (2006). Comparison of treatment potential of electrocoagulation of distillery effluent with and without activated Areca catechu nut carbon. Journal of Hazardous Materials , 137 (3), 18031809. [ Links ]
Kumar, M., Ponselvan, F., Malviya, J., Mall, I.D. & Srivastava, V. (2009). Treatment of biodigester effluent by electrocoagulation using iron electrodes. Journal of Hazardous Materials , 165 (1-3), 345-352. [ Links ]
Liang, Z., Wang, Y., Zhou, Y. & Liu, H. (2009). Coagulation removal of melanoidins from biologically treated molasses wastewater using ferric chloride. Chemical Engineering Journal , 152, 88-94. [ Links ]
Linares-Hernández, I., Barrera-Díaz, C., Roa-Morales, G., Bilyeu, B., & Ureña-Núñez, F. (2009). Influence of the anodic material on electrocoagulation performance. Chemical Engineering Journal , 148 (1), 97-105. [ Links ]
Ma, H., Cheng, X., Chen, S., Wang, C., Zhang, J., & Yang, H. (1998). An ac impedance study of the anodic dissolution of iron in sulfuric acid solutions containing hydrogen sulfide. Journal of Electroanalytical Chemistry, 451 (1-2), 11-17. [ Links ]
Maddala, J., Sambath, K., Kumar, V. & Ramanathan., S. (2010). Identification of reaction mechanism for anodic dissolution of metals using. Electrochemical impedance spectroscopy. Journal of electroanalytical chemistry , 638 (2), 183-188. [ Links ]
Mansouri, K., Elsaid, K., Bedoui, A., Bensalah, N., & Abdel-Wahab, A. (2011). Application of electrochemically dissolved iron in the removal of tannic acid from water. Chemical Engineering Journal , 172 (2-3), 970-976. [ Links ]
Martín, M., Raposo, F., Borja, R. & Martín, A. (2002). Kinetic study of the anaerobic digestion of vinasse pretreated with ozone, ozone plus ultraviolet light, and ozone plus ultraviolet light in the presence of titanium dioxide. Process Biochemistry , 37, 699-706. [ Links ]
Martínez-Navarro, F. (2007). Tratamiento de aguas residuales industriales mediante electrocoagulación y coagulación convencional . Tesis (Ph.D) Ingeniería Química. Universidad de Castilla La Mancha. Ciudad Real, España. [ Links ]
Mohana, S., Bhavik, K., Acharya, B. & Madamwar, D. (2009). Distillery spent wash: Treatment technologies and potential applications. Journal of Hazardous Materials , 163 (1), 12-25. [ Links ]
Morales, A. (2000). Extracción e identificación de compuestos orgánicos en vinazas . Tesis de Maestría en Ciencias-Química, Escuela de Química, Universidad del Valle, Cali, Colombia. [ Links ]
Morgan, B. & Lahav, O. (2007). The effect of pH on the kinetics of spontaneous Fe(II) oxidation by O2 in aqueous solution - basic principles and a simple heuristic description. Chemosphere , 68 (11), 2080-2084. [ Links ]
Navarro, A., Sepúlveda, M. d. C. & Rubio, M. (2000). Bio-concentration of vinasse from the alcoholic fermentation of sugar cane molasses. Waste Management , 20 (7), 581-585. [ Links ]
Pant, D. & Adholeya, A. (2007). Biological approaches for treatment of distillery wastewater: A review. Bioresource Technology , 98 (12), 2321-2334. [ Links ]
Prasad, R. & Srivastava, S. (2009). Sorption of distillery spent wash onto fly ash: Kinetics, mechanism, process design and factorial design. Journal of Hazardous Materials , 161 (2-3), 1313-1322. [ Links ]
Prasad, R., Kumar, R. & Srivastav, S. N. (2008). Design of Optimum Response Surface Experiments for Electro-Coagulation of Distillery Spent Wash. Water Air Soil Pollut , 191, 5-13. [ Links ]
Ryan, D., Gadd, A., Kavanagh, J., Zhou, M., & Barton, G. (2008). A comparison of coagulant dosing options for the remediation of molasses process water. Separation and Purification Technology , 58 (3), 347-352. [ Links ]
Sasson, M., Calmano, W. & Adin, A. (2009). Iron-oxidation processes in an electroflocculation (electrocoagulation) cell. Journal of Hazardous Material , 171 (1-3), 704-709. [ Links ]
Satyawali, Y. & Balakrishnan, M. (2008). Wastewater treatment in molasses-based alcohol distilleries for COD and color removal: A review. Journal of Environmental Management , 86 (3), 481-497. [ Links ]
Semerjian, L. & Ayoub, G. (2003). HighpH-magnesium coagulation-flocculation in wastewater Treatment. Advances in Environmental Research , 7 (2), 389-403. [ Links ]
Stratmman, M. & Franket, G. (2003). Corrosion and Oxide Films. In, A. Bard, M. Stratmann & G. Frankel (Eds.) Encyclopedia of Electrochemistry (vol. 4) (pp. 78-81). Indianapolis (IN): Wyley-VCH. [ Links ]
Thakur, C., Srivastava, V. & Mall, I. (2009). Electrochemical treatment of a distillery wastewater: Parametric and residue disposal study. Chemical Engineering Journal , 148 (2-3), 496-505. [ Links ]
Vijayaraghavan, K., Ramanujam, T. K. & Balasubramanian, N. (1999). In Situ Hypochlorous Acid Generation for the Treatment of Distillery Spentwash. Ind. Eng. Chem. Res. , 38, 2264-2267. [ Links ]
Voegelin, A., Kaegi, R., Frommer, J., Vantelon, D., & Hug, S.J. (2010). Effect of phosphate, silicate, and Ca on Fe(III)-precipitates formed in aerated Fe(II)- and As(III)-containing water studied by X-ray absorption spectroscopy. Geochimica et Cosmochimica Acta. , 74 (1), 164-186. [ Links ]
Vukovic, M. (1996). Anodic dissolution of Armco iron in 0.5 H2SO4 in the presence of adsorbed chloride ions. Hydrometallurgy , 42, 387-398. [ Links ]
Yavuz, Y., (2007). EC and EF processes for the treatment of alcohol distillery wastewater. Separation and Purification Technology , 53, 135140. [ Links ]
Revista Ingeniería y Competitividad por Universidad del Valle se encuentra bajo una licencia Creative Commons Reconocimiento - Debe reconocer adecuadamente la autoría, proporcionar un enlace a la licencia e indicar si se han realizado cambios. Puede hacerlo de cualquier manera razonable, pero no de una manera que sugiera que tiene el apoyo del licenciador o lo recibe por el uso que hace.