1. Introduction
During oil wells productive life it is normal to find water which is called as production water; in its composition suspended particles, dissolved hydrocarbons and inorganic salts such as calcium carbonate, calcium sulfate, strontium sulfate and barium can be found 1. Most of the oil fields in the world are characterized by producing large volumes of water with oil, as mentioned by Bailey et al. 2 who express that global water production is approximately 210 million barrels per day (33.4 million m3/d) produced with 75 million barrels per day (11.9 million m3/d) of oil, being perceived as a common situation in mature fields, establishing water excess represents a problem and a significant cost for oil producing companies.
This water generates scale which is formed either when the inorganic salts are solidified at certain pressure and temperature conditions, 3-6 or when water injection is applied as a recovery method, generating scale due to incompatibility between injected water and groundwater. Scaling phenomenon is one of the most common problems in oil industry because it generates damages to distribution systems, shortening their useful life and obstructions in the pipelines, causing pressure drops and decreasing hydrocarbon production./
These accumulations in greater proportion are formed in different stages such as formation pores, in well vicinity or in the production pipes, valves and pumps, gunship holes, well completion equipment and surface facilities 4,7,8, causing a reduction in porosity and permeability and an early hole obstruction, thus making difficult the natural displacement fluids and causing great economic losses 3,9,10. For this reason, in cases where scale formation has occurred, the problem is solved applying chemical or physical methods, using acidic solutions or mechanical equipment, respectively 11-13.
There are mechanisms that help preventing scale formation and growth such as inhibitors 14, which applied in small quantities in production waters have successfully inhibited the formation of these precipitates 15 through mechanisms of threshold effect, crystal distortion, dispersion and chelation 16,17, these agents are in general synthetic formulations of specific chemical compounds that produce high environmental impact 18 because they are not biodegradable. These inhibitors are generally composed of phosphates: inorganic polyphosphates, organic phosphate esters, organic phosphonates, organic aminophosphates, and organic polymers. Nowadays, motivated by the growing global concern about the use of environmentally compatible and safe products, in addition to rigorous environmental laws, efforts have been made to minimize damage caused by to these inhibitors 6,19,20. This represents some of the reasons to develop scale inhibitors based on eco-friendly compounds and natural substances.
Relevant studies have been made about scale bioinhibitors which have provided important information for this research, such as Suharso et al. 21, researchers of gambier extract effect as a calcium carbonate precipitation inhibitor; Guo et al.22, who used a modified chitosan copolymer to prevent calcium carbonate scale deposition; use of Okra extract (Abelmoschus esculentus) as a calcium carbonate inhibitor additive by Malavé et al. 23; Bendaoud-Boulahlib and Ghizellaoui 24 measured orange peel inhibitory effect on scale-generating waters; in addition to the study by Rendón et al. 25 who evaluated dehydrated Passiflora edulis pectin effect as a mineral scale inhibitor.
Accordingly, the purpose of this research was to evaluate an inhibitor with organic properties based on linseed (Linum usitatissimum), in order to generate a proposal that would suggest the feasibility of its use in order to minimize problems caused by scales in oil industry and having as a hypothetical experimental premise the calcium carbonate scale inhibition in samples of synthetic and production water from Oritupano oilfield, in Venezuela. Based on this, water samples were characterized, as well as the mucilage obtained from linseed and the anti-scaling agents. Experimental products (at different concentrations) were then evaluated, using NACE TM0374-2007 26; and finally making a statistical comparison between the efficiencies of experimental additives and a commercial product, through an analysis of variance (ANOVA).
2. Methodology
The study was conducted at Hydrocarbon Processing, Drilling and Reservoir Laboratories of Petroleum Engineering Department, Universidad de Oriente, Núcleo de Monagas, Campus Los Guaritos; and Molecular Sieves Laboratory, Universidad de Oriente, Núcleo de Monagas, Campus Juanico, located in Monagas state, Venezuela.
2.1 Synthetic and production water samples characterization
Before characterizing, synthetic water was formulated by mixing a solution of sodium chloride (35 g/L) and calcium carbonate (10 g/L). Once synthetic water was formulated, synthetic brine and a production water sample from Oritupano field, located in Anzoátegui state, Venezuela, were characterized physicochemically, using the different tests and standards indicated below: density (ASTM D369 ) 27, dissolved solids content (COVENIN 2342: 86) 28, total and suspended solids content (COVENIN 2461: 87) 29, conductivity (COVENIN 3050-93) 30, pH (COVENIN 2462 : 87) 31, alkalinity (API RP 13B-1) 32, in addition to chloride content (COVENIN 3141: 1995) 33 and calcium hardness (COVENIN 2771: 91) 34.
2.2 Preparation and characterization of Linum usitatissimum mucilage
First of all raw material was required for being used in scale inhibitor formulation, this is linseed mucilaginous gel which is obtained by hydrolysis process, where heat was applied to both seeds and distilled water (in 100 g of linseed per 1000 mL of water proportion) for about 15 minutes. Produced gel was filtered to separate it from seeds. Once the resulting gel was at ambient temperature, it was centrifuged to separate aqueous phase from the oils, and then it was placed in a decanting funnel for a period of 24 hours for obtaining a more efficient separation of free water contained. Gel was preserved at a temperature of 20.5 °C to prevent its degradation.
Mucilage characterization was made through the determination of viscosity, using Brookfield viscometer (ASTM D2983-03) 35; functional groups identification through analysis by infrared spectroscopy on FT-IR spectrometer equipment (Nicolet is10 ) (ASTM E168-06 36 and ASTM E1252-98 37); microscopic appearance (ASTM E1951-98) 38 by direct observation in a DIGISYSTEM (XSZ-207) biological microscope, and thermal stability (Method developed by Malavé et al.) 23 setting three values of temperature (50, 70 and 90 °C). Density, total, dissolved and suspended solids, pH and conductivity were determined through the standards procedures considered for synthetic and formation water characterization.
2.3 Linseed mucilage inhibitor development
It was started with formulation of the different scale inhibitor samples, based on three concentrations (30, 40 and 50% m / V), mixing linseed gel obtained with distilled water; diluting 30, 40 and 50 grams of linseed gel for every 100 mL of distilled water, and 0.02 g/L of citric acid was added for each one as a preservative. Inhibitors were applied in two doses specified by NACE TM 0374-2007 26 (10 and 100 ppm), with 55 ppm added as a third dosage to establish comparative statistical analysis.
Once different antiscaling formulations were made, they were characterized from their physicochemical properties. The tests made were: viscosity, density; content of total, dissolved and suspended solids, pH, conductivity and thermal stability; following standard procedures already indicated. Additionally, it was required a commercial inhibitor as a reference for statistical analysis. This came from an unknown manufacturer but commonly used by Petróleos de Venezuela, S.A. (PDVSA). Commercial inhibitor was also characterized through: viscosity, density, total solids content and pH.
Linseed scale inhibitor evaluation according to NACE TM 0374-2007 26 was based on determining the antiscaling chemicals ability to keep calcium carbonate (CaCO3) soluble, preventing their precipitation during the tests. Different linseed scale inhibitors and commercial product were used in these tests. In order to calculate efficiency, it was necessary to determine concentration corresponding to water sample calcium hardness before and after the test without inhibitor dosage (cold and hot sample, respectively), using the Eq. 1:
Where:
Ca: calcium hardness in ppm of treated sample after precipitation.
Cb: calcium hardness in ppm of blank after precipitation (hot sample).
Cc: calcium hardness in ppm of blank before precipitation (cold sample).
Additionally, a polynomial regression analysis was performed with results obtained from inhibitor performance test in synthetic water sample and in production water sample, thus for verifying products behavior experimentally at concentrations above 50 %m/V, at the best performing dose. In order to complement linseed scale inhibitor evaluation, a statistical study was conducted using the Statgraphics Centurion XVI computational program, considering the results obtained in the previous tests. The analysis consisted of comparing dosed samples efficiencies with the developed product, and dosed samples efficiencies with the commercial product, considering ANOVA methodology for this analysis, based on comparing multiple samples means and determining which of them were different.
3. Results and discussion
3.1 Physicochemical properties of water samples and linseed mucilage obtained
Table 1 shows that the two water samples evaluated were classified according to their hardness as "very hard", presenting values greater than 300 ppm, following what is stated in COVENIN 2771: 91 standard 34, which gives the tendency to have a high scaling power. Therefore, it will increase when the calcium hardness increases because the carbonate compounds will transform into crystals of the corresponding element when they experience an increase in temperature 39. This scaling tendency is ratified with the results obtained from dissolved solids, generally associated with salts with a solubility higher degree and that according to Becker 40 the mineral crystals precipitation depends mainly on dissolved salts and even more so if they are lower size; in addition, a high total solids content increases the waters scaling tendency 41.
Other properties that confirm the scaling nature are pH and alkalinity. Production water from Oritupano oilfield obtained a value of 7.45, while for synthetic water was 9.45; indicating for the last case a basic nature and, although the production water pH value is relatively neutral, this is not an obstacle to minerals precipitation in case of any change in pressure or temperature experienced by this fluid. Furthermore, in most oilfields the groundwater has pH values in a range from 6 to 8.5 42.
Table 1 Water samples physicochemical properties
Property | Measurement unit | Production water | Synthetic water |
---|---|---|---|
Density | g/mL | 1.0252 | 1.0567 |
Total solids | ppm | 31,130 | 43,880 |
Dissolved solids | ppm | 30,040 | 35,600 |
Suspended solids | ppm | 1,090 | 8,280 |
pH | - | 7.45 | 9.54 |
Conductivity (25 oC) | mS/cm | 43.50 | 52.60 |
Calcium hardness | ppm | 9,200 | 760 |
Chlorides | ppm | 1,900 | 2,300 |
Partial alkalinity | ppm | 0 | 40 |
Total alkalinity | ppm | 700 | 1020 |
The two samples studied were also characterized by their high chlorides content giving it a corrosive tendency, considering what is established by the World Health Organization, which states that a chlorides value greater than 250 ppm influences the water corrosivity 43).
Table 2 shows the results obtained after the linseed mucilage characterization. Gel color was light yellow with a gelatinous appearance and an acidic pH (5.59), due to the high lignans content and polyunsaturated fatty acids (more than 70%), mostly α-linolenic acid (ALA) 44. Linseed gel has good foaming capacity, stability, stable viscosity and it is resistant to the presence of salts in a wide pH range 45. This gives the mucilage the inhibitory capacity in water samples with salts its composition and its viscosity can be considered as the main characteristic on which its applicability inhibitor is projected because it is stable enough, appreciating that the gel had a low viscosity at the beginning of its preparation (at a temperature of approximately 80 °C), but on cooling viscosity increased considerably which confirms that this property is strongly dependent on temperature.
Table 2 Linum usitatissimum gel properties
Property | Measurement unit | Result |
---|---|---|
Density | g/mL | 1.006 |
Viscosity | cP | 91.5 |
pH | - | 5.59 |
Conductivity (25°C) | mS/cm | 1,114 |
Total solids | ppm | 9,260 |
Dissolved solids | ppm | 8,820 |
Suspended solids | ppm | 440 |
Temperature action on the gel is verified when the thermal stability test is performed, being the way to ensure that mucilage does not lose its properties or inhibition mechanism when it is subjected to temperature variations. Figure 1 shows the curves that describe linseed gel behavior when it was subjected to different temperature conditions (50 ºC, 70 ºC and 90 ºC) during a determined time period (8 hours). The gel showed a slight mass decrease at temperatures of 50 ºC and 70 ºC respect to the initial value, but after two hours of temperature exposure, the mass stabilized until its decrease was not appreciable. This allows to deduce that gel will tend to maintain stability respect to the 50 and 70 ºC temperatures, at times above 8 hours.
On the other hand, at temperature of 90 ºC it is observed that gel mass variation was more significant and did not stabilize, because mass decreased while the exposure time increased at that temperature. Based on this, it is inferred that when linseed gel was subjected to temperatures below 70 °C, it did not thermally degrade, but above this temperature the gel became more unstable, presenting greater mass variations, because the bonds that stabilized it were broken and gel was thermally degraded. From the study by Rendón et al.25 where they used dehydrated Passiflora edulis pectin as a scale inhibitor, the behaviors similarity of both natural polymers at the first two temperatures (50 °C and 70 °C) is shown, while for pectin referential a high percentage decline (54.73%) at the temperature of 90 °C (from the beginning to the end of the test) was observed, with respect to the new proposal based on linseed (0.33%); what confers to linseed mucilage better stability to high temperatures.
In relation with total solids in linseed gel they result to be 9860 ppm, because linseed composition that shows a wide range of proteins, carbohydrates, lipids, fibers and presence of minerals such as potassium, phosphorus, iron, zinc and manganese 46; in addition to impurities (seed remains) which appeared during gel extraction.
Figure 2 shows a linseed gel sample optical micrograph, appreciating that this sample is mostly occupied by a uniform and practically homogeneous liquid environment, characteristic of mucilage gelatinous body and the aqueous medium through which it was obtained. Also, formation of small bubbles inside by the air encapsulation is shown. It was not possible to appreciate in more detail other characteristics present in linseed gel, because the microscope resolution was lower than needed.
Figure 3 shows signals obtained in linseed gel infrared spectrum. Infrared spectroscopy is a qualitative method where each bond type absorbs infrared radiation at a different frequency when it is transmitted through a thin layer of substance, allowing the functional groups that the sample studied has to be identified, but it does not allow determining the exact proportions that they have 47. Generally, it scans from 4000 cm-1 to 400 cm-1, allowing a transmittance or absorbance spectrum generation, indicating which wavelengths the infrared is absorbed by the sample
Infrared (IR) spectrum is unique for any chemical compound; therefore for linseed gel composition there is a spectrum that characterizes it. Figure 3 visualizes presence of hydroxyl groups (OH-) and water, appearing in a range between 3600 and 3100 cm-1, this corresponds to phenols in gel composition; also, between 2950 and 2870 cm-1, aliphatic carbon-hydrogen elongation (C-H) is evident.
In the interval between 2870 and 1800 cm-1, presence of a carboxylic group possibly associated with lipids found in linseed gel is visualized. Subsequently, an H-O-H flex appears due to water presence in steam form at 1650 cm-1, as well as the possible monosubstituted aromatics presence belonging to a carbonyl group (C = O). Aliphatic carbon-hydrogen (C-H) flexion is also observed at 1390 cm-1, due to fatty acids presence, abundant in gel composition; between 1190 cm-1 and 1000 cm-1, a C-O stretch is evident, caused by carboxylic acid group presence, probably associated with polysaccharides in gel content; and finally, oscillations between 900 cm-1 and 400 cm-1 are visualized due to distortions and impurities contained in sample.
3.2 Proposed inhibitor development
3.2.1 Formulated inhibitors characterization
Table 3 summarizes results obtained, showing inhibitors density (at different concentrations) with an upward tendency and directly related to mucilage concentration, also affecting the solubility of each formulated product when used as antiscaling agents. Those density values are directly associated with solids amount shown in Table 3. In relation with scale inhibitor properties, total solids content is expected to be as low as possible, in order not to introduce more solids into the system; this parameter does not determinate directly inhibitor efficiency, but its consideration is important. Similarly, high suspended solids concentrations are undesirable in scale inhibitor, because scale inhibitor must be able to fully dissolve in water sample to effectively perform its function of preventing crystal formation. Related to this, Mata 48 suggests when applying inhibitors, suspended solids presence should be taken into account, because these affect maximum efficiency of the additive.
Table 3 Formulated inhibitors characterization at different Linum usitatissimum gel concentrations
Property | Measurement unit | 30 %m/V | 40 %m/V | 50 %m/V |
---|---|---|---|---|
Density | g/mL | 1.00575 | 1.00702 | 1.0072 |
Viscosity | cP | 17.7 | 29.5 | 35.4 |
pH | - | 3.43 | 3.28 | 3.21 |
Total solids | ppm | 3,240 | 3,920 | 4,180 |
Dissolved solids | ppm | 3,020 | 3,600 | 3,800 |
Suspended solids | ppm | 220 | 320 | 380 |
Associated with solids content in each formulation, viscosity increases are shown while solid material proportion is increased, which are associated with fibers, carbohydrates and minerals 46, provided by mucilage. Considering viscosity is not the only property that directly influences the inhibitor efficiency, because it will also depend on certain conditions, such as pressure and temperature, salts content, total solids present in water and solids present in formulated inhibitor 48.
Obtained pH values were 3.43; 3.28 and 3.21 for inhibitors with concentrations of 30, 40 and 50 % m/V, respectively; showing that pH tends to decrease at a higher concentration, because a greater linseed gel quantity is added when formulated, giving a greater acidity to inhibitor as mucilaginous material proportion used is increased and additionally, citric acid ratio used as a preservative contributed to decrease pH, in a range between 38.60% and 42.60%. In addition, most commercial scale inhibitors are acidic and based on this, it is known that at lower pH inhibitors will be more efficient, preventing scale formation.
Formulated product inhibition mechanism was determined through the infrared spectrum made to linseed gel, obtaining polysaccharides in its composition, associated with a carbonyl group located at 1650 cm-1 and a carboxylic group between 1190 cm-1 and 1000 cm-1. According to Daun et al.44 these polysaccharides are mainly composed of arabino-rhamnose, galactose, xylose and galacturonic and manuromic acids, with xylose (62.8%) being the main component of neutral (electrically) polysaccharide, while rhamnose (54.5% ) represents the main component of acidic polysaccharide. Inhibitors antiscaling capacity may have come from xylose and ramnose, creating a protective film that generates nucleation changes of calcium carbonate crystals 49.
In addition, Hall, et al45 express that linseed gel is a polydisperse complex hydrocolloid and different rheological behaviors are caused by differences in ratio between neutral and acidic polymers and by molecular weight and polysaccharides structural conformation. Linseed gel also has a powerful cation chelating agent and complexer of proteins and starch, such as phytic acid, which could possibly have prevented compounds precipitation such as calcium carbonate. It also represents between 60 and 90% of phosphorus present in linseeds 44.
3.2.2 Commercial product characterization
A commercial inhibitor commonly applied in oil industry was used as a comparative standard which was provided by the state oil company Petróleos de Venezuela, S.A (PDVSA). This inhibitor was characterized, obtaining following values shown in Table 4.
Table 4 Commercial scale inhibitor properties
Property | Measurement unit | Result |
---|---|---|
Density | g/mL | 1.06445 |
Viscosity | cP | 12.9 |
pH | - | 4.37 |
Total solids | ppm | 0 |
According to tabulated results, a commercial product with favorable characteristics of a scale inhibitor is exhibited, such as relatively low density and viscosity which give it the miscibility characteristics in medium where they were tested (water), in addition to an acid pH and a non-existent solids content, which generate inhibition characteristics of mineral particles accumulations formation present in water samples and no solids contribution to evaluation systems, respectively.
3.2.3 Linseed mucilage experimental inhibitors efficiency
After fluid characterizations, formulated bioinhibitors were evaluated (at three concentrations) in samples of synthetic brine and Oritupano oilfield production water, using three previously specified dosages (10, 55 and 100 ppm), as shown in Table 5. Efficiency percentages were obtained between 18.89% (30 %m/V inhibitor at a 10 ppm dose, evaluated in synthetic water), up to a maximum efficiency of 95.67% (50 %m/V inhibitor at a 100 ppm dose, evaluated in production water); it should be noted that the best results were obtained at 100 ppm dose (77.67; 90.00 and 95.67% for 30, 40 and 50 %m/V concentrations, respectively), even exceeding efficiencies obtained by commercial inhibitor. Showing that greater or lesser inhibitors efficiency is attributed to the fact that at higher antiscaling product concentration calcium in solution retention during tests was higher, preventing salts precipitation 25.
Table 5 Linum usitatissimum scale inhibitor efficiency results
Concentration (%m/V) | Dose (ppm) | Efficiency (synthetic brine) (%) | Efficiency (production water) (%) |
---|---|---|---|
30 | 10 | 18.89 | 39.00 |
55 | 44.44 | 56.67 | |
100 | 63.33 | 77.67 | |
40 | 10 | 30.00 | 53.33 |
55 | 55.56 | 65.67 | |
100 | 88.89 | 90.00 | |
50 | 10 | 36.67 | 59.00 |
55 | 63.33 | 71.00 | |
100 | 92.22 | 95.67 | |
Commercial inhibitor | 10 | 25.56 | 55.67 |
55 | 47.78 | 62.33 | |
100 | 77.78 | 82.33 |
It also shows a better performance of experimental product in its different concentrations when it is evaluated in production water sample, which in a cold condition obtained a calcium hardness of 9200 ppm, greater than synthetic water (760 ppm), which allows us to infer that its performance was more satisfactory for a water sample with a higher scaling degree.
Comparing with results of Malavé et al. 23 notable difference is verified, because when using Okra pod a maximum efficiency of 80% was achieved, for a 600 ppm dosage of a product with 5 %m/V concentration, in a production water sample with a 50 ppm calcium hardness, which is obviously lower than hardness of water used in this study (9200 ppm -production water- and 760 ppm -synthetic water-), where maximum efficiencies, as already indicated, resulted above 90%. The opposite case occurs with proposal made by Suharso et al. 21, who through use of gambier extract obtained efficiencies between 60 and 100%, using product doses between 50 and 250 ppm, respectively; positioning the studied agent (Linum usitatissimum) as a good option to use in operational conditions, for calcium carbonate scale precipitation control.
Figures 4 and 5 show behaviors resulting from polynomial regression performed assuming concentrations greater than 50 %m/V, noting that as inhibitor concentration increases above the mentioned value, the efficiencies in both water samples are decreased. The maximum efficiencies were 92.22% (for synthetic brine), at a 50 %m/V concentration, and 96.07% for production water (at a 54 %m/V concentration linseed gel), these being the ideal for 100 ppm dose and with which it could have a greater effect in mineral scale inhibition. In addition, according to the hypothetical behaviors shown in respective graphs, a better product performance is revealed (at maximum dose of 100 ppm) in production water, showing a less pronounced decrease above the highest concentration used experimentally.
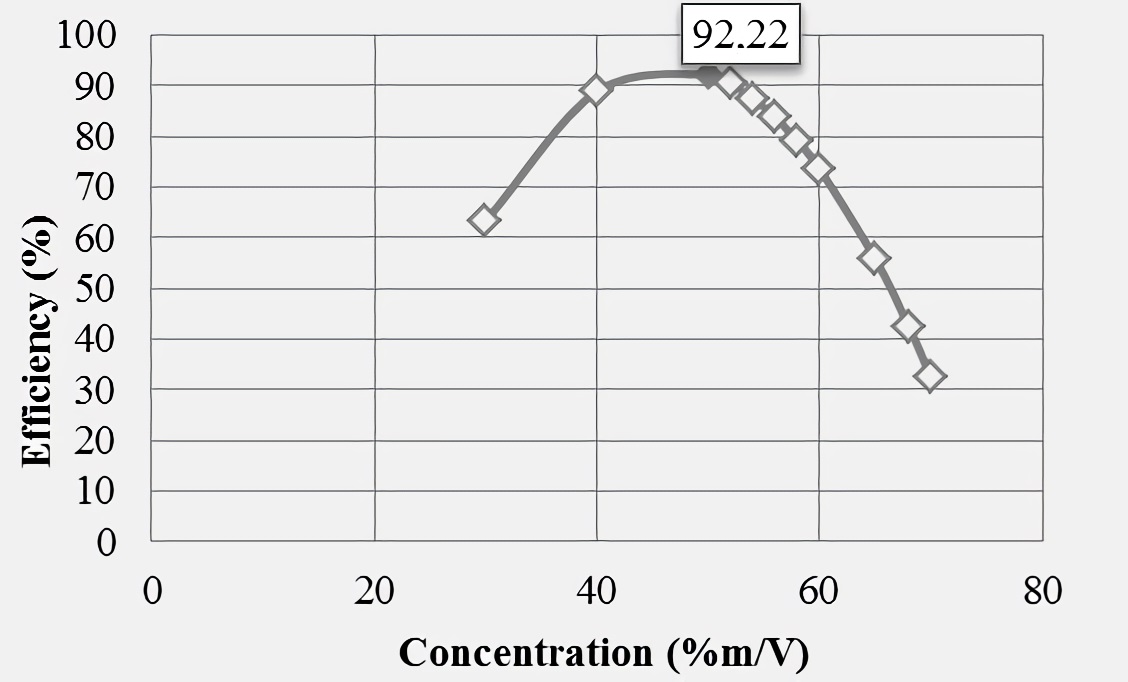
Figure 4 Graphical representation of polynomial regression for concentrations greater than 50% m/V at 100 ppm dose in synthetic brine
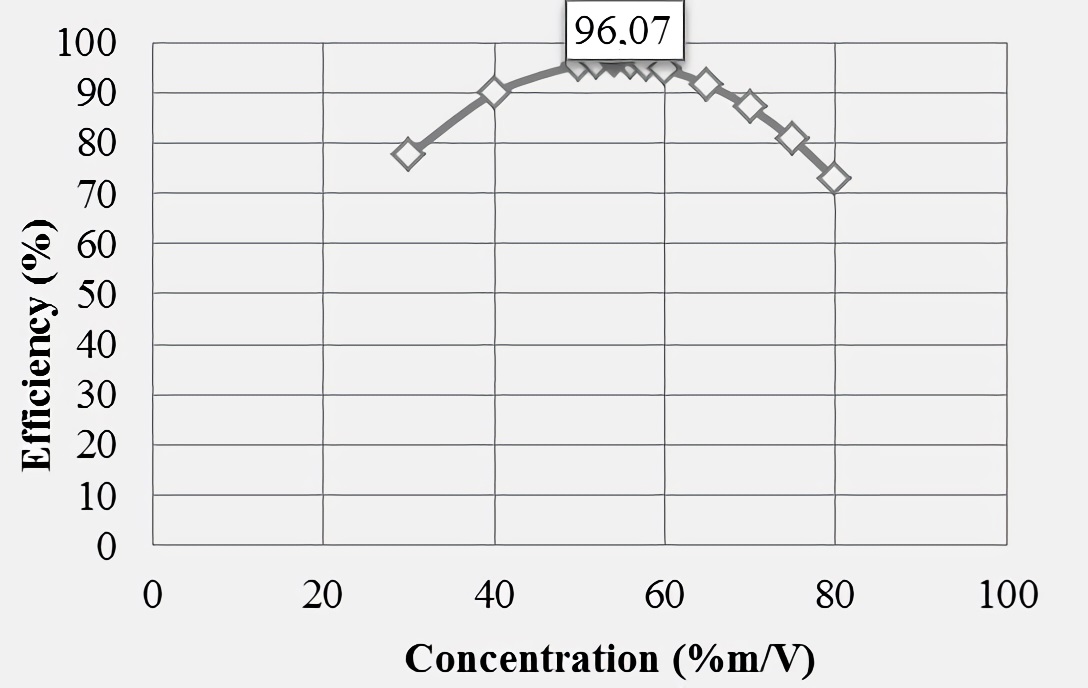
Figure 5 Graphical representation of polynomial regression for concentrations greater than 50% m/V at 100 ppm dose in production water
Figure 6 graphically specifies the behaviors found according to the efficiencies of experimental inhibitors and commercial product, in advance of statistical analysis performed. This graph shows that Linum usitatissimum mucilage scale inhibitors in their respective concentrations (30, 40 and 50 %m/V), showed similar upward behaviors with each other, also showing that in two waters considered for the study, the commercial antiscaling agent, only showed better performance than the organic product with the linseed mucilage lowest concentration (30 %m/V), evidencing superior behaviors of the other concentrations compared to the commercial reference product. This is corroborated in following statistical table.
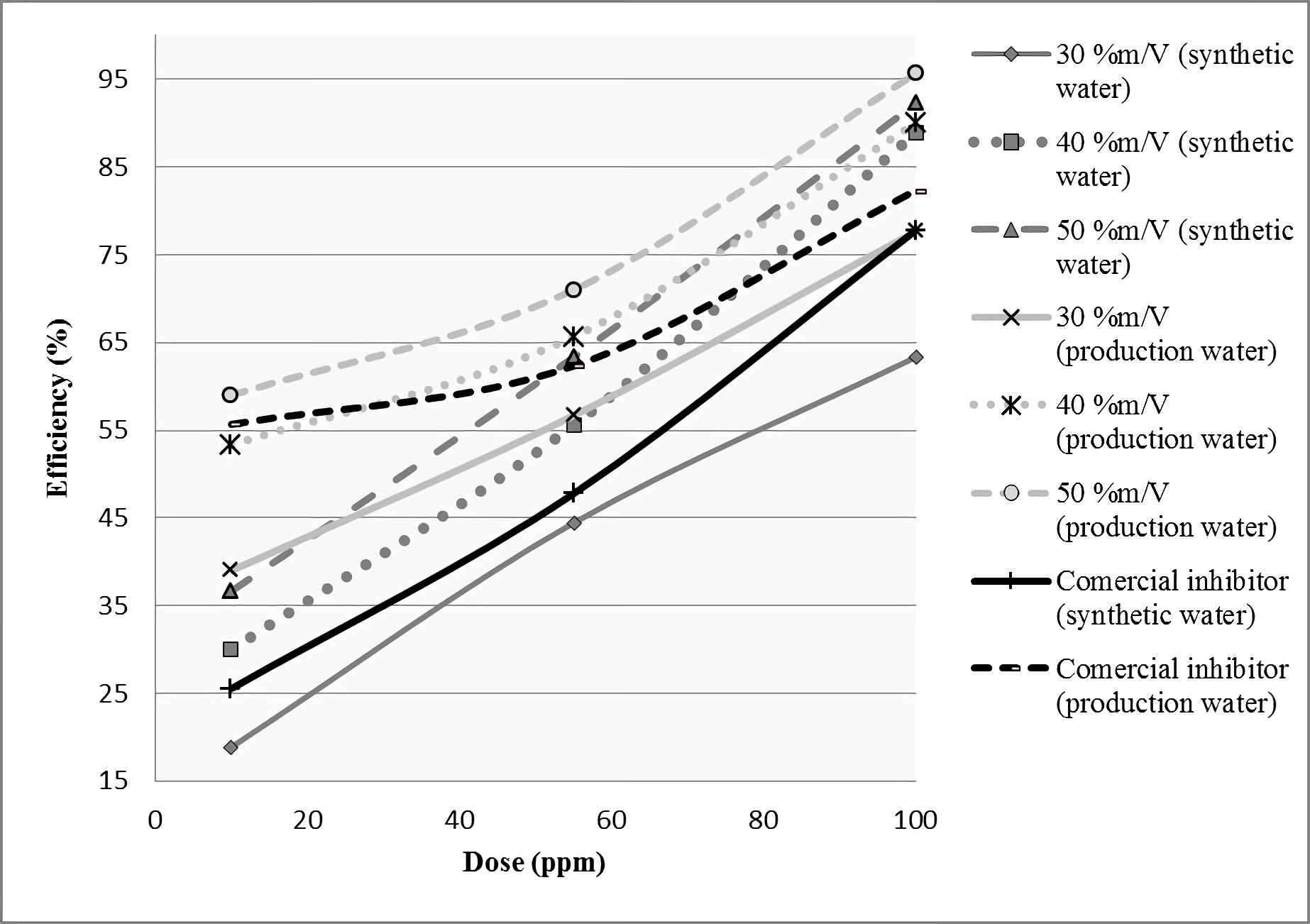
Figure 6 Experimental linseed-based inhibitors efficiency behaviors, respect to reference commercial product
Table 6 shows statistical results of the analysis made to linseed gel inhibitors of 30, 40 and 50 %m/V concentration compared with the reference product; there is not statistically significant difference between efficiencies means shown by formulated additives and commercial inhibitor, because the P value was greater than 0.05; this indicates that antiscaling inhibitors used showed similar effects in water samples studied.
Table 6 Simple analysis of variance for efficiencies of Linum usitatissimum-based inhibitor and commercial product
Source | Sum of squares | Fd | Middle square | F-ratio | P-value |
---|---|---|---|---|---|
Between groups | 2,407.77 | 7 | 343.967 | 0.67 | 0.6929 |
Intra groups | 8,186.18 | 16 | 511.636 | ||
Total (Corr.) | 10,594.0 | 23 |
Comparing the studied proposal, where a natural polymer from linseed was used, with product developed by Guo et al. 22 based on a modified chitosan copolymer, a similarity is verified in maximum efficiencies results obtained (95.67% versus 95.62%); considering the composition of two primary sources are different, it is shown that it was not necessary to chemically modify the linseed mucilage, so that it exhibited the same efficiencies as those provided by a modified polymer, whose transformation is developed essentially to improve its efficiencies. It is valid to indicate then, that a linseed gel modification could improve the efficiencies and a high preservation capacity.
4. Conclusions
The inhibitory capacity antiscaling agent based on Linum usitatissimum mucilage was more favorable in production water sample than in synthetic brine with a tendency to early CaCO3 precipitation, obtaining better results (95.67%) at higher concentrations and doses (50% m/V and 100 ppm, respectively) and showing a directly proportional concentration-efficiency behavior, resulting more satisfactory for linseed bioinhibitors of 40 and 50% m/V concentration than that provided by commercial product used for comparative purposes. Furthermore, they did not show significant differences in their behaviors, among themselves and with the reference agent when the analysis of variance was elaborated. It is convenient to evaluate the developed product behavior at concentrations above 50% m/V and at dosages above 100 ppm.