1. Introduction
Several emerging pollutants present in personal care products, drugs, perfumes and cosmetics have been pouring into water resources frequently and extensively in recent years. These emerging pollutants found in water have been considered endocrine disruptors 1, therefore, they are of potential risk to the health of living beings, especially some that are bio-accumulative.
Some emerging pollutants such as bisphenol A, phthalates, parabens and synthetic thematic pyrethroids are not persistent because they are easily decomposed in the environment, being metabolized in the body, however, they are also endocrine disruptors and are suspected of affecting human reproduction and development 2. Among these pollutants, Triclosan (TCS) is frequently used in cleaning products, food industries, personal care, such as toothpaste, liquid soaps, textiles, polymers, among others. TCS is detected in various water sources such as raw, waste and drinking water, despite passing through water treatment plants, it remains in treated water 3-5.
Evidence has been found linking exposure to TCS with reproductive and developmental health of animals 6. Adverse health effects have been reported in aquatic organisms attributed to endocrine disruptors, such as developmental, neurological, endocrine, and reproductive disorders 7,8.
Aranami and Readman 9 state that TCS acts as an endocrine disruptor for various aquatic organisms, especially fish, since it bioaccumulates in them and has a direct correlation with the time of exposure to pollutants during their lifetime. Likewise, the concentrations of endocrine disruptors are associated with the risk of subfertility due to the hormonal alterations that they generate 10. Fish are also significantly affected in their reproductive activity, delaying the hatching time of the eggs, as an effect of endocrine disruption 11. Goodbred et al. 12 evaluated the incidence of 252 organic chemical compounds, among them TCS. They showed that high concentrations of polychlorinated biphenyls (PCBs), Dichloro Diphenyl Trichloroethane (DDT), polybrominated diphenyl ethers (PBDEs), galaxolide and methyl triclosan decrease the concentration of ketostestosterone. Although gonadosomatic index and sperm motility did not differ in results based on sampling sites, sperm count decreased by 50%, reducing reproduction due to exposure to contaminants. On the other hand, the fish generically called "tilapia" have received perhaps more attention than any other group of fish in the world. The species Oreochromis mossambicus belongs to the Cichlidae family in the order of the perciformes of the genus Oreochromis, it is considered a freshwater species native to Africa, which spread to the north of Israel and Jordan. After the Second World War these were introduced in countries of Asia and America 13 for commercial activities such as aquaculture.
Tilapia is highly consumed by humans due to its protein richness, its content of essential amino acids, nutrients such as vitamins and minerals, and it is of great value for aquaculture production. At the global level, fish consumption has remained above population growth, growing at an average annual rate of 3.2% (1961-2013) compared to world population growth, which was 1.6% in this period 14.
Unfortunately, the environmental conditions of tilapia can be altered by the presence of emerging pollutants in rivers, lakes and freshwater ponds that can cause adverse effects on the fish's organism 4. Therefore, it is necessary to know the response of tilapia to the presence of emerging pollutants. In this study, we propose as an objective to know how TCS affects sperm motility in tilapia fish.
2. Methodology
2.1. Endorsement of the ethics committee
The experimental phase was approved in minutes 99 on September 29, 2015 by the Ethics Committee for experimentation with animals of the University of Antioquia, Colombia.
2.2. Obtaining biological material and experimental design
For the experimental phase, 24 sexually mature male tilapia (Oreochromis spp.) with weighing between 293.3 ± 46.8 g were obtained from the ponds of the San José del Nus fish farm in Antioquia (University of Antioquia). The selected specimens were transferred to circular basins with a capacity of 65 liters for each individual for a period of 4 weeks in order to adapt them to the experimental conditions to reduce the stress caused by handling and change of environment. Subsequently, each specimen was transferred to a tank with a capacity of 40 liters, to start the experimental phase. Table 1 shows the temperature and pH conditions of the water for each treatment.
Table 1 Temperature and pH parameters of the treatments.
pH 7 | pH 9 | |||||||
---|---|---|---|---|---|---|---|---|
0 µg/L | 50 µg/L | 120 µg/L | 190 µg/L | 0 µg/L | 50 µg/L | 120 µg/L | 190 µg/L | |
T (°C) | 23.3 ± 0.3 | 22.9 ± 0.4 | 23.8 ± 0.9 | 23.8 ± 0.9 | 23.1 ± 0.3 | 22.8 ± 0.3 | 22.9 ± 0.2 | 22.8 ± 0.2 |
pH | 7.02 ± 0.2 | 7.1 ± 0.2 | 7.1 ± 0.2 | 7.1 ± 0.2 | 8.9 ± 0.1 | 9.01 ± 0.03 | 8.9 ± 0.04 | 9.01 ± 0.04 |
2.3. Obtaining semen
Each one of the fish was extracted from the tank, the abdomen of the fish was dried and abdominal pressure was applied in a cephalocaudal direction to cause the expulsion of urine, water or faeces, avoiding contamination to the sperm sample. Abdominal pressure was again performed to collect the semen sample in dry, sterilized 1.5 mL glass tubes.
2.4. Determination of sperm motility
The sperm motility of the spermatozoa was analyzed, taking into account their fast, medium and slow motility as response variables. For this, 0.13 µL (n = 3) of semen was taken from each of the fish in a Neubauer counting chamber (Marienfeld, USA); later the mobility was activated by adding 40 µL of distilled water at neutral pH, completely filling the chamber. Response variables were measured between 15 and 20 seconds after activation expressed as a percentage. 200 sperm were counted, the fast sperm were those with speeds greater than 100 μm/s, the medium ones with speeds between 50 and 100 μm/s and the slow ones with speeds between 10 and 50 μm/s through a Carl Zeiss Axio imager microscope (Zeiss, Germany) at 40x. Obtaining an average data per sample of the variables listed above, the product of three analyzes per field within the time after the activation of sperm motility.
2.5. Statistical analysis
A design with K = 3 factors was made: TCS with four levels (0, 50, 120 and 190 µg/L), pH with two levels (7.0 and 9.0) and response measurement time at 0, 3 and 7 days with three individuals per treatment. All the data of the evaluated variables were expressed as mean ± standard deviation. Tests were performed for the residuals complying with the parameters of normality, homogeneity of variance, randomness and data independence. A transformation of the data with arc sine was carried out to obtain a normal distribution and in this way, they were presented in the results. The significant statistical analyzes were approved by an analysis of variance (ANOVA) followed by the Tukey test of multiple comparisons between treatments (p˂0.05) through R Project Statistical computing.
2.5.1. Effects model
The model of effects between the variables and the factors evaluated in the experimental design is presented in Eq. 1.
In this case:
A: Number of TCS concentration levels: 4 (0, 50, 120 and 190 µg/L)
B: Number of pH levels (7, 9)
C: Number of levels respect at time (Days 0, 3 and 7)
i: i-th level of TCS concentration factor, in this case associated with the i-th TCS concentration (0, 50, 120 and 190 µg / L), for this case i = 1,2,3,4 because are 4 levels associated with concentration factor.
j: j-th level of pH factor, in this case associated with the j-th pH content (7 and 9), in this case j = 1,2 because there are 2 levels associated with the pH factor.
K: K-th level of the time factor, in this case associated with the K-th content of the measurement time (0, 3 and 7 days), in this case K = 1,2,3 because there are 3 levels associated with the variable's measurement time factor reply.
y ijk : evaluation of sperm motility of tilapia spp. at the i-th level of TCS concentration factor of the j-th level of the pH factor and K-th level of the time factor and the l-th replication performed.
μ:Global mean.
α𝑖: Effect due to the 𝑖 - th level of factor A (TCS concentration).
β j :Effect due to the j-th level of factor B (pH).
γK: Effect due to the K-th level of factor C (Time).
(αβγ)ijk: Effect of the interaction in the combination ijk (Interaction between concentration, pH and time).
ε ijk : Random error associated to factors
3. Results
3.1. Rapid motility
Treatments exposed to 0, 50 and 120 µg/L of TCS, they did not show significant differences in the first measurement of exposure to TCS evaluated at pH 7, however, treatment exposed to 190 µg/L decreased significantly (p ˂0.05) its rapid motility respect to 50 and 120 µg/L treatments (Figure 1). Treatment with 50 µg/L of TCS at pH 7 significantly decreased 34% and 42% for the second and third measurement respectively compared to the first measurement. In addition, no significant differences were observed between the measurements at 0, 3 and 7 days for the concentrations of 0, 120 and 190 µg/L of TCS at pH 7, as shown in Figure 1.
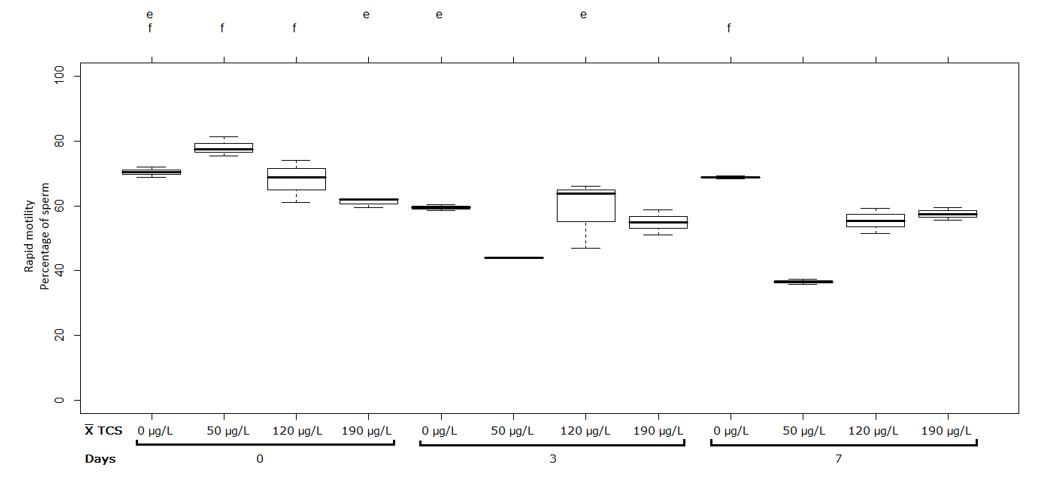
Figure 1 Sperm with rapid motility (%) in semen of Oreochromis spp. Treatment at pH 7. Between half letters different are significantly different (p˂0.05) n = 3
The treatments at pH 9 showed significant differences (p˂0.05) in the first measurement between the control group (0 µg/L) and the treatment at 120 µg/L of TCS. In the second and the third measurement, there was a significant difference between the control group (0 µg/L) and the treatments at 50, 120 and 190 µg/L of TCS because the rapid motility decreased significantly (p˂0.05) for the concentrations: 50 µg/L with 73.10 ± 12.03% and 38.76 ± 6.30%; 120 µg/L with 83.95 ± 2.68% and 33.83 ± 7.70%; 190 µg/L with 79.11 ± 2.31% and 28.28 ± 9.61% for the first and the second measurement, respectively.
However, no significant differences were observed between the second and the third measurements at 50, 120 and 190 µg/L of TCS at pH 9 (Figure 2). On the other hand, the ANOVA table of the rapid motility variable is presented, which shows the summary of significance of each factor and its interaction (Table 2).
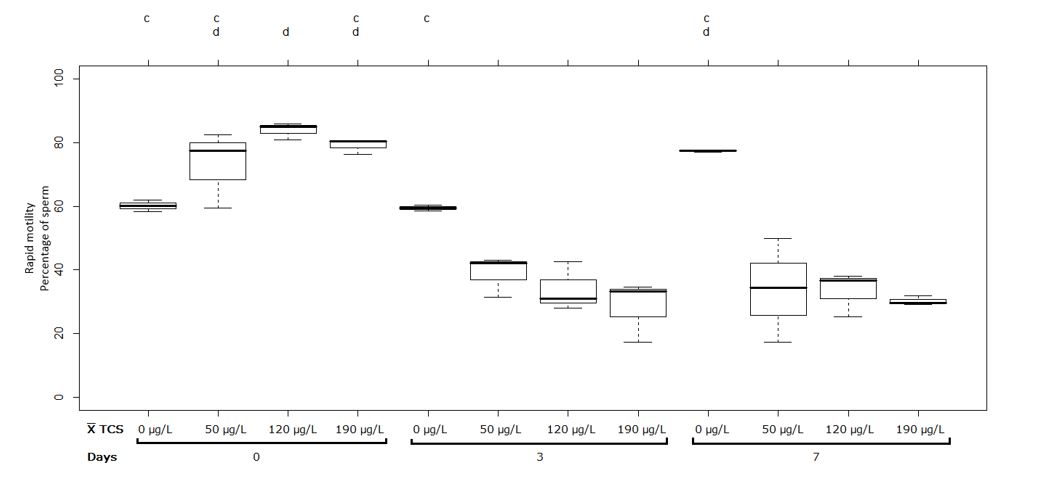
Figure 2 Sperm with rapid motility (%) in semen of Oreochromis spp. Treatment at pH 9. Between half letters different are significantly different (p˂0.05) n = 3
Table 2 ANOVA variable rapid motility
Factors | Sum of squares | Degrees of freedom | Middle square | F 0 | value- p | ||||
---|---|---|---|---|---|---|---|---|---|
A | 𝑆𝑆 A | 232 | 𝑎−1 | 2 | 𝑀𝑆 A | 116 | 𝑀𝑆 A / 𝑀𝑆 E | 2.41 | 0.10 |
B | 𝑆𝑆 B | 1083 | 𝑏−1 | 1 | 𝑀𝑆 B | 1083 | 𝑀𝑆 B / 𝑀𝑆 E | 22.48 | 3.29 e-05 |
C | 𝑆𝑆 C | 12175 | 𝑐−1 | 2 | 𝑀𝑆 C | 6088 | 𝑀?? C / 𝑀𝑆 E | 126.35 | ˂2e-16 |
A*B | 𝑆𝑆AB | 152 | (𝑎−1) (𝑏−1) | 2 | 𝑀𝑆 AB | 76 | 𝑀𝑆 AB / 𝑀𝑆 E | 1.58 | 0.21 |
A*C: | 𝑆𝑆AC | 1271 | (𝑎−1) (𝑐 −1) | 4 | 𝑀𝑆 AC | 318 | 𝑀𝑆 AC / 𝑀𝑆 E | 1.62 | 0.18 |
B*C | 𝑆𝑆 BC | 2329 | (𝑏−1) (𝑐 −1) | 2 | 𝑀𝑆 BC | 1165 | 𝑀𝑆 BC / 𝑀?? E | 24.17 | 2.21 e-07 |
A*B*C | 𝑆𝑆 ABC | 1271 | (𝑎−1) (𝑏−1) (𝑐 −1) | 4 | 𝑀𝑆 ABC | 318 | 𝑀𝑆 ABC / 𝑀𝑆 E | 6.59 | 0.00043 |
Residual | 𝑆𝑆𝑟 | 1734 | 𝑎𝑏𝑐(𝑛 −1) | 36 | 𝑀𝑆𝑟 | 48 |
3.2 Medium motility
Between the treatments 0, 50, 120 and 190 µg/L in the first measurement did not show significant differences at the two pH levels (Figure 3 and 4). The treatment at 50 µg/L of TCS at pH 7 increased the medium motility significantly when comparing the first measurement of 11.80 ± 2.85% with the second measurement 47.08 ± 0.79%, the other treatments at this pH level did not show significant differences as shown see in Figure 3.
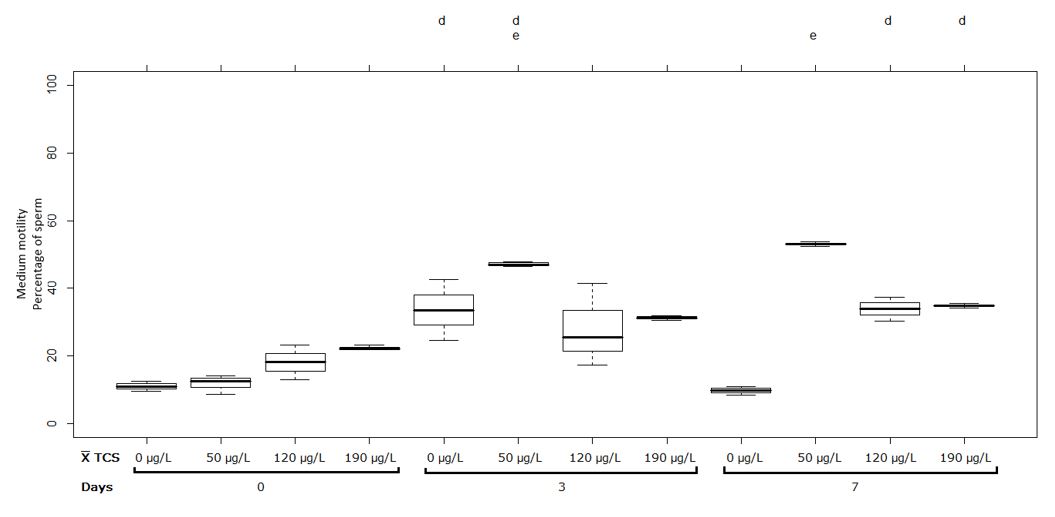
Figure 3 Sperm with medium motility (%) in semen of Oreochromis spp. Treatment at pH 7. Between half letters
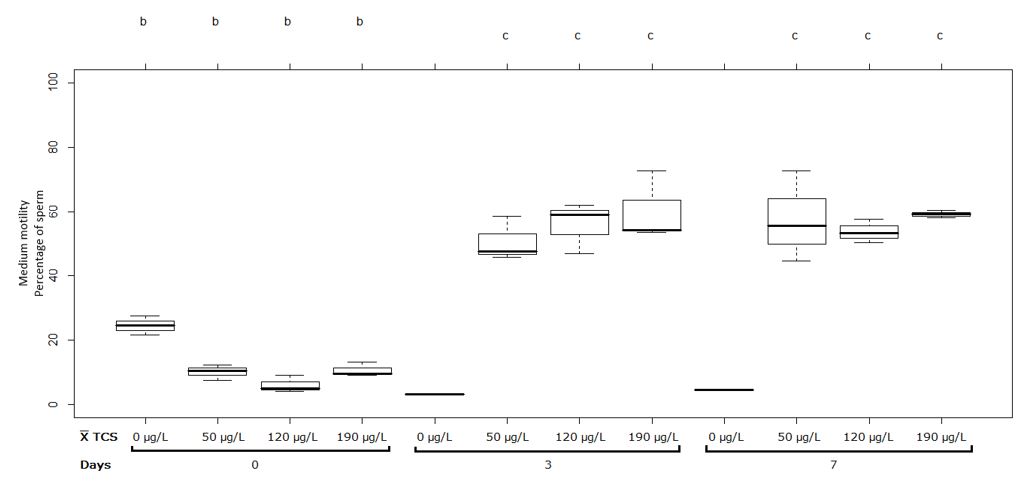
Figure 4 Sperm with medium motility (%) in semen of Oreochromis spp. Treatment at pH 9. Between half letters different are significantly different (p˂0.05) n = 3
For the treatments at pH 9, no significant difference was observed in the first measurement, however, in the second and the third measurement the treatments at 50, 120 and 190 µg/L showed a significant increase (p˂0.05) in the medium motility respect to the control group (0 µg/L) as presented in Figure 4.
Thus, the medium motility increased significantly (p˂0.05) for the concentrations: 50 µg/L with 10.08 ± 2.33% and 50.61 ± 6.94%; 120 µg/L with 6.04 ± 2.68% and 55.97 ± 8.02%; 190 µg/L with 10.63 ± 2.27% and 60.23 ± 10.88% between the first and the second measurement respectively (Figure 4). Between the second and the third measurements, no significant differences were observed for treatments 50, 120 and 190 µg/L of TCS at pH 9 (Figure 4).
It should be noted that in Figures 3 and 4 in comparison with Figures 1 and 2, the medium motility increased through the measurements that were made, contrary to what happened in the evaluation of rapid motility.
The following is the statistical summary of the ANOVA in Table 3 for this variable.
Table 3 ANOVA variable medium motility
Factors | Sum of squares | Degrees of freedom | Middle square | F 0 | value ̶p | ||||
---|---|---|---|---|---|---|---|---|---|
A | 𝑆𝑆 A | 5.17 | 𝑎−1 | 2 | 𝑀𝑆 A | 2.58 | 𝑀𝑆 A / 𝑀𝑆 E | 5.19 | 0.010 |
B | 𝑆𝑆 B | 12.77 | 𝑏−1 | 1 | 𝑀𝑆 B | 12.77 | 𝑀𝑆 B / 𝑀𝑆 E | 25.67 | 1.22 e-05 |
C | 𝑆𝑆 C | 288.09 | 𝑐−1 | 2 | ??𝑆 C | 144.05 | 𝑀𝑆 C / 𝑀𝑆 E | 289.71 | ˂2e-16 |
A*B | 𝑆𝑆AB | 5.0 | (𝑎−1) (𝑏−1) | 2 | 𝑀𝑆 AB | 2.50 | 𝑀𝑆 AB / 𝑀𝑆 E | 5.02 | 0.011 |
A*C: | 𝑆𝑆AC | 6.91 | (𝑎−1) (𝑐 −1) | 4 | 𝑀𝑆 AC | 1.73 | 𝑀𝑆 AC / 𝑀𝑆 E | 3.47 | 0.016 |
B*C | 𝑆𝑆 BC | 39.73 | (𝑏−1) (𝑐 −1) | 2 | 𝑀𝑆 BC | 19.86 | 𝑀𝑆 BC / 𝑀𝑆 E | 39.95 | 7.24 e-10 |
A*B*C | 𝑆𝑆 ABC | 13.83 | (𝑎−1) (𝑏−1) (𝑐 −1) | 4 | 𝑀𝑆 ABC | 3.46 | 𝑀𝑆 ABC / 𝑀𝑆 E | 6.95 | 0.0002 |
Residual | 𝑆𝑆𝑟 | 17.90 | 𝑎𝑏𝑐(𝑛 −1) | 36 | 𝑀𝑆𝑟 | 0.50 |
3.3 Slow motility
As can be seen in Figure 5, the treatments at pH 7 at 50 µg/L had a significant increase (p˂0.05) between the first and the second measurement of 1.4%. In addition, the treatments at 120 and 190 µg/L did not show significant differences between these measurements. However, it showed a significant decrease (p˂0.05) in slow motility at 190 µg/L, between the second and the third measurements with 3.06 ± 0.55% and 0.76 ± 0.25% respectively.
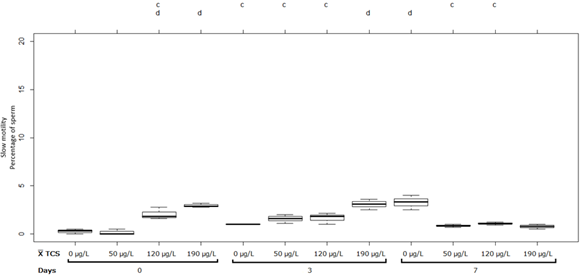
Figure 5 Sperm with slow motility (%) in semen of Oreochromis spp. Treatment at pH 7. Between half letters different are significantly different (p˂0.05) n = 3
On the other hand, the treatments evaluated at pH 9 did not show significant differences between treatments for slow motility (Figure 6).

Figure 6 Sperm with slow motility (%) in semen of Oreochromis spp. Treatment at pH 9. Between half letters different are significantly different (p˂0.05) n = 3
In order to evaluate the influence of the factors and the possible interactions between them for slow mobility, the summary of significance between factors is presented, as shown in Table 4.
Table 4 ANOVA variable slow motility
Factors | Sum of squares | Degrees of freedom | Middle square | F 0 | Value ̶p | ||||
---|---|---|---|---|---|---|---|---|---|
A | 𝑆𝑆 A | 8.15 | 𝑎−1 | 2 | 𝑀𝑆 A | 4.07 | 𝑀𝑆 A / 𝑀𝑆 E | 8.70 | 0.0008 |
B | 𝑆𝑆 B | 11.18 | 𝑏−1 | 1 | 𝑀𝑆 B | 11.18 | 𝑀𝑆 B / 𝑀𝑆 E | 23.88 | 2.12 e-05 |
C | ??𝑆 C | 3.23 | 𝑐−1 | 2 | 𝑀𝑆 C | 1.61 | 𝑀𝑆 C / 𝑀𝑆 E | 3.45 | 0.042 |
A*B | 𝑆𝑆AB | 2.18 | (𝑎−1) (𝑏−1) | 2 | 𝑀𝑆 AB | 1.09 | 𝑀𝑆 AB / 𝑀𝑆 E | 2.33 | 0.11 |
A*C: | 𝑆𝑆AC | 4.25 | (𝑎−1) (𝑐 −1) | 4 | 𝑀𝑆 AC | 1.06 | 𝑀𝑆 AC / 𝑀𝑆 E | 2.27 | 0.08 |
B*C | 𝑆𝑆 BC | 5.29 | (𝑏−1) (𝑐 −1) | 2 | 𝑀𝑆 BC | 2.64 | 𝑀𝑆 BC / 𝑀𝑆 E | 5.65 | 0.007 |
A*B*C | 𝑆𝑆 ABC | 6.39 | (𝑎−1) (𝑏−1) (𝑐 −1) | 4 | 𝑀𝑆 ABC | 1.59 | 𝑀𝑆 ABC / 𝑀𝑆 E | 3.41 | 0.018 |
Residual | 𝑆𝑆𝑟 | 16.86 | 𝑎𝑏𝑐(𝑛 −1) | 36 | 𝑀𝑆𝑟 | 0.46 |
4. Discussion
Sperm mobility could be altered by environmental factors in the water due to the presence of certain contaminants, affecting the sperm quality of the fish, and therefore, affecting their genetics and physiology 15. Several organic pollutants found in water sources are biologically active and persistent 15,16.
Personal care products such as triclosan can promote the modulation of thyroid hormone 17,18. Thus, triclosan has been shown to have negative effects on human sperm motility 19 and on the decrease in sperm count in Gambusia affinis20.
In this study, it was found that the exposure of male tilapia to TCS at the concentrations evaluated produced a significant decrease in sperm motility. Although the fertilization capacity was not evaluated at these concentrations of TCS, spermatozoa that have a high mobility have a greater opportunity of fertilization of the oocyte 21.
On the other hand, it was evidenced that the control fish (0 µg/L) showed rapid motility at pH 7 and 9 between 73 and 95%. The results are consistent with the studies conducted by Navarro Rodrigo et al. 22 who verified similar results in sperm motility studies reported in Oreochromis spp. between 89 and 100%; Guerrero - Quiroz et al. 23 observed motility percentages higher than 94% in this species; Triastuti et al. 24 reported motility percentages of 93.4% in tilapia.
In the present study, a significant decrease in the fast motility variable was found when the fish were exposed to 50 µg/L of TCS at pH 7 and for treatments at 50, 120 and 190 µg/L at pH 9 respect to the measurement of the beginning and half of the treatments. However, no significant difference was observed between the second and the last measurement for all treatments. Consequently, some emerging pollutants and their metabolites in water sources have been inversely related to sperm motility 25,26.
On the other hand, Wang et al. 27 indicate that organochlorine groups have a higher risk of abnormality in the sperm quality of sperm; Goodbred et al. 12, who compared the body loads of 252 chemical substances considered pollutants in fish, showed that they have different influence on sperm motility. In this study was found that at 50 µg/L of TCS at pH 7 the rapid motility decreased to 42% in the third measurement compared to the first; at 50, 120 and 190 µg/L at pH 9 the motility was less than 39% in the second measurement. Triclosan has a pKa of 8.14 28, which indicates that at pH above 8.14 triclosan is in the form of phenoxide and below 8.14 as phenol, which indicates that they are 2 different substances, which they do not affect with the same intensity as endocrine disruptor. These results are contrasted with those obtained by Goodbred et al. 12 who reported that PCBs, DDTs, galaxolide PBDEs and methyl triclosan affect the sperm count, at values below 50%. On the other hand, this decrease in sperm mobility and quality has been evidenced in other male fish species, such as Gambusia affinis exposed to 101.3 µg/L of TCS significantly reduced the sperm count 20; the sperm quality of goldfish exposed to 100 μg/L TCS was significantly reduced after a 4-week exposure period 29.
According to Van Look and Kime 30, the loss of sperm motility may be associated with alterations in cell structure, such as changes in the central part of the flagellum, an area that contains mitochondria and is involved in energy production. In addition, the interaction of the fish with the contaminant caused a disruptive process altering mobility, since ATP synthesis is necessary for the activation and sustainability of sperm mobility 31,32. Therefore, the arrest or prolongation of mobility depends on the availability of ATP 33. In this way, the decrease in mobility can be associated with damage to the mitochondria because it is the energetic organelle of the sperm 34. Consequently, the results for medium motility increased significantly by 35% in the treatment at 50 μg/L at pH 7 and between 40 to 49% for the treatments at 50, 120 and 190 μg/L at pH 9 between the first and the second measurements. For this reason, the increase in the variables medium and slow sperm motility could be due to the influence of the pollutant on the endocrine system 35, influencing the decrease in sperm motility that could cause the ability of sperm to penetrate the micropyle is weak, and therefore could affect the fertilization rates of the species 24.
Likewise, the increase in slow mobility indicates that the male reproductive system is susceptible to exposure to environmental pollutants, as confirmed by Pflieger-Bruss and Schill 36. It has also been shown in other studies that the accumulation of contaminants in fish is a very critical factor, as it can negatively affect the process of spermiogenesis and the interruption of reproduction 37,38.
5. Conclusions
The exposure of Oreochromis spp. to the factors evaluated in this investigation showed significant results regarding the evaluation of sperm motility. Rapid motility was reduced due to the time of exposure to TCS having a higher incidence at the highest pH level evaluated. On the other hand, the variables of medium and slow motility for some treatments increased significantly, which could indicate an alteration in the sperm cell membrane. Furthermore, an affectation at the mitochondrial level could be inferred due to a possible reduction in ATP synthesis and therefore would explain the reduction in rapid motility.