1. Introduction
Tartrazine (Trisodium 1-(4-sulfonatophenyl)-4-(4-sulfonatophenylazo)-5-pyrazolone-3-carboxylate) is a deep yellow to bright orange petroleum derivative with a molecular weight of 534.4 g/mol. It is an azo dye widely used in the pharmaceutical industry in the coating of medicinal capsules, syrups and cosmetics. It is also used in a variety of edible materials and candied products as a colorant 1. Its high solubility in water maximizes the probability of finding it contaminating industrial effluents. Tartrazine is considered responsible for allergy and toxicity problems in humans and can act as a catalyst in various behavioral problems such as hyperactivity. It can also cause asthma, migraines, eczema, thyroid cancer and lupus 2.
Due to its azo-anionic nature, tartrazine has low biodegradability under environmental conditions. For this reason, it is necessary to treat wastewater containing dyes before discharge into the environment. Several chemical and physical processes, such as photocatalysis 3, flocculation, chemical coagulation, precipitation and adsorption, have been widely used to treat dye-bearing wastewater, with adsorption being one of the most efficient methods 4. Among these methods, adsorption is one of the most widely used since it is inexpensive, works for low contaminant concentrations, and various materials can be used as adsorbents 5. Agricultural wastes are a good source for dye adsorption because they are cheap and abundantly available; they consist mainly of cellulose, hemicellulose and lignin, which bind as effective adsorbents for a wide range of contaminants since they contain hydroxyl, carboxyl, phenol, methoxy and amino functional groups 6. These natural lignocellulosic materials are good adsorbents for cationic contaminants such as heavy metals and dyes such as methylene blue due to the net negative charge on their surface 7.
Natural lignocellulosic materials are good adsorbents for cationic contaminants such as heavy metals and dyes such as methylene blue due to the net negative charge on their surface 8, bentonite modified with hexadecyltrimenthylammonium bromide 9, rice ash/polypropylene membranes 10, biochar from moringa 11 and Lantana camara12, and hen feathers 13. From the kinetic and equilibrium study, excellent results have been obtained, achieving high adsorption capacities of the pollutant, fast kinetics and a positive effect of the increase of the initial concentration at low amounts of adsorbents.
In the present investigation, we used cetyl trimethyl ammonium chloride (CTAC) to modify the cellulose extracted from the wheat husk (WR) for its evaluation in the removal of tartrazine; we obtained the new adsorbent MWC (modified wheat waste cellulose) and performed a comparative study with unmodified cellulose (WC). Characterization analysis was performed before and after adsorption using Fourier transform infrared spectroscopy (FTIR). The effect of adsorbent dosage, initial dye concentration and contact time on the removal efficiency was studied. The effect of contact time, adsorbent dose and initial concentration were determined. The adsorption equilibrium was studied, and the experimental data were fitted to the Langmuir and Freundlich models.
2. Methodology
2.1. Materials and reagents
The determination of tartrazine in solution was carried out in a UV/Vis spectrophotometer Shimadzu UV 1700 Hach DR 2700 (Japan). Hydrochloric acid and sodium hydroxide solutions at 0.1 M were used for pH adjusting. Tartrazine (Trisodium 1-(4-sulfonatophenyl)-4-(4-sulfonatophenylazo)-5-pyrazolone-3-carboxylate) >85% of dye content by Sigma Aldrich® (USA) was used for the preparation of the dye solution.
2.2. Variables and design of experiments
The experiment was carried out following a multilevel factorial experimental design 33. The response variable was the removal efficiency, and the independent variables were the variation of the adsorbent dose (15, 25 and 35 mg) and the initial concentration (40, 70 and 100 mg/L).
2.3. Biomass pretreatment
The wheat milling residues were washed with deionized water to remove soluble compounds and eliminate dirt and impurities, dried in an oven at 60 ºC until constant mass and reduced in size in a knife mill. The size classification was made in a Shaker type sieve shaker by means of a series of sieves, which present the opening sizes of 8, 6.3, 4.75, 3.35, 2.362, 2, 1.7, 1, 0.5 and 0.355 mm 14.
2.4. Cellulose extraction
The method proposed by Xu et al. 15 was used for cellulose extraction. Initially, the pretreated wheat milling residues were subjected to double alkaline extraction; for this, 20 g of dry biomass was immersed in 500 mL of 4%w NaOH solution, with the agitation of 200 rpm at 80 ºC in a plate with magnetic agitation for 2 h. Then, the sample was washed with abundant distilled water, and the process was repeated. Subsequently, delignification was performed to eliminate lignin or polyphenols and proteins as follow: to the sample was added a delignification solution prepared with 50 g of sodium chloride added to 500 mL of distilled water and 50 mL of glacial acetic acid. The mixture was put under contact for 24 h, at room temperature continuously stirred. The resulting mixture was filtrated and washed with distilled water. Finally, the resulting sample was dried at 50 °C for approximately 2 to 3 h until to constant mass.
2.5. Cellulose quaternization
The quaternization of cellulose was carried out with CTAC at a concentration of 100 mmol/L. It was added 10 mL of CTAC for each 1 g of cellulose, the mixture was placed in contact for 24h with the agitation of 300 rpm. Then, the sample was washed with abundant distilled water until neutral pH.
2.6. Adsorbents characterization
Wheat milling residues, wheat cellulose, and cellulose modified with CTAC were characterized by Fourier transform infrared spectroscopy (FTIR), before and after tartrazine removal, in order to determine the possible functional groups involved in the dye adsorption process 16. The zero charge point pH (pHpzc) was determined to establish the charge balance on the surface of the bioadsorbent 17.
2.7. Adsorption Tests
The adsorption tests were carried out following the experiments' proposed design, varying the adsorbent dose and the initial concentration. For this, 5 mL of contaminated solution was placed in contact with 250 rpm agitation at room temperature for 24 h, in a Thermo Scientific MAXQ 4450 orbital shaker. The remaining concentration of tartrazine was determined by infrared spectrophotometry at 500 nm. The removal efficiency (%R) was determined with the Eq.1 18.
Where
The statistical analysis was performed using Statgraphics Centurion XVIII.I.II software, determining the effect of each of the variables on the material removal efficiency, based on the analysis of variance, Pareto diagram and response optimization. The effect of contact time was determined at the best experimental condition of initial concentration and adsorbent dose found at 250 rpm and room temperature. Aliquots were taken at different time intervals (5, 10, 20, 30, 30, 60, 60, 120, 240, 480, 720 and 1440 min), and the remaining dye concentration was determined.
2.8. Adsorption isotherms
The adsorption isotherm was determined by varying the initial solute concentration (25, 50, 75, 100, 125 and 150 ppm), at the best condition of adsorbent dose and contact time found experimentally, at 150 rpm and room temperature 19. The experimental data were fitted to the Langmuir and Freundlich models in order to interpret the adsorbate-adsorbent interactions 20.
3. Results
3.1. Characterization of bioadsorbents
Figure 1 shows the IR spectra for wheat waste (WR), wheat cellulose (WC) and modified wheat cellulose (MWC) before and after tartrazine adsorption. Before adsorption, WR and WC showed a similar spectrum, with a strong and broad peak at 3350 cm-1 that is characteristic for stretching vibration of hydroxyl, carboxyl and amine group corresponding to functional groups' vibration in cellulose and hemicellulose 21. Amines and carbonyl's appearance by the stretching vibrations of the O-H bond is located between 3400 and 3500 cm-1 (22,23. At 2904 cm-1 a peak attributed to C-H bonds present in the CH2 and CH3 hydrocarbon groups is observed. Wavelengths between 2000 and 2500 cm-1 show slight signals from alkynes and carboxylic acids.
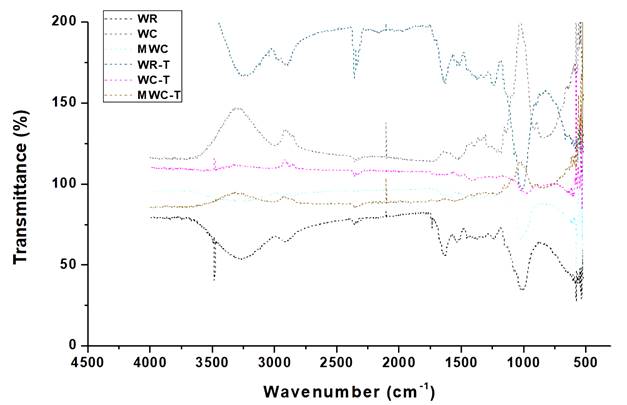
Source: own elaboration
Figure 1 IR spectra of bioadsorbents from wheat residues before and after adsorption of tartrazine.
The typical band assigned to cellulose is observed in the region between 1669 and 829 cm-1, exhibiting stretching and bending vibrations by -CH2 and -CH, -OH and C-O bonds in cellulose bonds, located from 1490 to 829 cm-1. The absorbance band around 1418 cm-1 is associated with the crystalline structure of cellulose, while around 900 cm-1 it is related to the amorphous region of cellulose 24. The presence of the aforementioned functional groups in the 400-4000 cm-1 spectrum is attributed to the high content of lignin, cellulose and hemicellulose in the structure of the biosorbents 25. After modification, an increase in the % transmittance of the functional groups is evident due to the increase in energy levels, as well as the broadening of bands between 800 and 1200 cm-1, 1250 cm-1, 2178 cm-1 and 2850 cm-1, attributed to perturbations in the cellulose structure due to the modification with CTAC.
The point at which the bioadsorbents exhibit zero net charge was determined using the pHPZC26. Table 1 summarizes the results for the bioadsorbents under study. Bioadsorbents prepared from wheat residues have an acidic pHPZC. The crude biomass and cellulose presented a pHPZC of 4.92 and 4.69, respectively, slightly lower than the pH range for crude celluloses (5.0-7.5) 27. When it is chemically treated with CTAC, its pH increases. From the pHPZC values, it was determined that the operating pH for the adsorption tests would be 4 for the three adsorbents; this, considering that their adsorptive capacity depends, to a great extent, on the electrostatic exchange with the contaminant; thus, the surface of the material would be positively charged and would attract the anions due to electrostatic forces 28.
3.2. Effect of adsorbent dosage and initial concentration
Figure 2a shows that the adsorption of tartrazine on WR showed negative values, which may be due to the fact that the active centers of the biomass would be negatively charged as a result of the presence of OH, carboxyl and amino groups; likewise, the biomass could contribute color to the contaminated solution 11. However, as the concentration of tartrazine increases, desorption is lower because the concentration gradient becomes smaller 12.
Figure 2b shows that WC presented a maximum adsorption peak of almost 40% for a dose of 35 mg and contaminant concentration of 40 mg/L. This low percentage of removal is due to the fact that the cellulose surface is anionic in nature thanks to the presence of OH- groups 29. This behavior on the part of the raw biomass and cellulose evidences the need to treat the biomaterials in order to increase the adsorption sites of anionic contaminants. When using MWC, a significant increase in tartrazine's removal efficiency was evidenced, achieving removal percentages >95 % in all cases, with positive influence of the initial concentration of tartrazine, which would favor diffusive phenomena and mass transfer from the solution to the free surface of the adsorbent 19. Nevertheless, the effect of incresing adsorbent dosage was negative, as it causes a split in the concentration gradient between the solute concentration in solution and on the adsorbent surface 30.
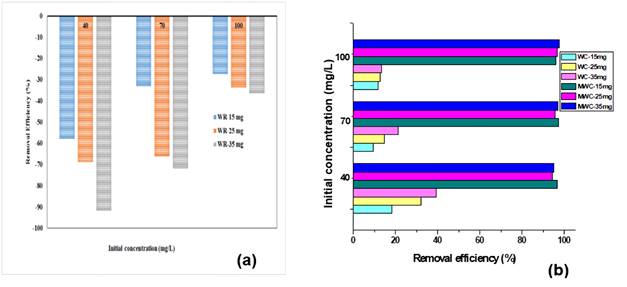
Source: own elaboration
Figure 2 Adsorption efficiency of tartrazine at different adsorbent dosage and initial concentration conditions using (a) WR y (b) WC y MWC.
The abouve would cause a decrease in the adsorption capacity due of competition of solute ions for the limited available binding sites, electrostatic interactions, overlapping or aggregation of adsorption sites, low surface area, interference between binding sites and lower ion contact at higher adsorbent densities 31. Similar results similar have been previously reported, showing the adsorption capacity reduction from 691.4 mg/g to 295.9 mg/g when evaluating different initial concentrations (300, 500 and 700 mg/L) and increasing the adsorbent mass from 0.2 to 1.0 g 32. Thus can be explained by agglomeration of particles at high doses of adsorbent, decreasing at same time specific surface area and the adsorption sites 33. Further, major adsorbent doses may increase the available adsorption sites, which could results in a lower saturation and a adsorption capacity 34.
3.3. Statistical analysis
From the results obtained, the F-factor values shown in Table 2 were determined for the percentage eliminations of tartrazine; the data reported for WR were not considered, due to their negative values. A confidence level for the P-Value of 95% was established. Therefore variables with a P-Value of less than 0.05 are considered significant. 11. It was found that the adsorption of the dye on WC showed statistical significance for the adsorbent dose (A), the initial concentration of tartrazine (B), and their interactions AB and BB 35.
Table 2 ANOVA for the removal efficiency of tartrazine on bioadsorbents prepared from wheat residues.
Source | Bioadsorbent | |||||
---|---|---|---|---|---|---|
WC | MWC | |||||
Sum of squares | F-Ratio | P-value | Sum of squares | F-Ratio | P-value | |
A:Adsorbent dosage | 197.021 | 94.14 | 0.0023 | 0.0216 | 0.08 | 0.7991 |
B:Initial concentration | 450.202 | 215.13 | 0.0007 | 2.7744 | 9.92 | 0.0513 |
AA | 1.4650 | 0.70 | 0.4642 | 1.9339 | 6.92 | 0.0783 |
AB | 93.9365 | 44.89 | 0.0068 | 2.3870 | 8.54 | 0.0614 |
BB | 76,0371 | 36.33 | 0.0092 | 0.3814 | 1.36 | 0.3272 |
Total error | 6.2782 | 0.8387 | ||||
Total (corrected) | 824.94 | 8.3369 |
Source: own elaboration
3.4. Effect of time
The effect of contact time at pH 4, initial concentration of 100 mg/L and 15 mg adsorbent dose was evaluated, showing the effect of time on the removal efficiency of tartrazine (Figure 3). It is observed that the equilibrium adsorption of the dye on WC occurred around 200 min, achieving a maximum adsorption efficiency of 30.94 % at 720 min. When using MWC, the adsorption rate was slow, reaching 76 % removal of tartrazine at 240 min and equilibrium at 720 min.
From the adsorption behavior of tartrazine on the bioadsorbents synthesized from residual wheat husk, adsorption-desorption phenomena during the process are evident. MWC presented an adsorption capacity at equilibrium of 13.25 mg/g while WC presented an adsorption capacity at equilibrium of 1.49 mg/g. Considering the trend followed by the adsorption kinetics, it can be said that adsorption occurred in two stages: first slow step which was dominated by diffusion from the solution to the adsorbent surface, followed by the second faster phase until reaching equilibrium dominated by mass transfer and electrostatic interactions between the active centers and the dye 30. Equilibration time has been reported between 3 and 4 hours when using defatted soybeans and bottom ash as adsorbent 13; an equilibrium time of 600 min was obtained when using bioadsorbents prepared from moringa seeds, achieving a removal efficiency of 99%, with adsorption capacities ranging from 3.3 to 3.9 mg/g 11, which were much lower than those presented in the present study for MWC.
3.5. Adsorption isotherms
From the parameters in Table 3, it can be said that the Freundlich model described the adsorption equilibrium of tartrazine on WC and MWC. This indicates that the limiting step controlling the process is the chemical adsorption that occurs in multilayers, with different adsorption energies and a non-uniform heat distribution; this is due to the diversity of the active centers of the two adsorbents 36.
Table 3 Fitting parameters to adsorption isotherm models
Model | Parameters | WC | MWC |
---|---|---|---|
Langmuir | qmax (mg/g) | 83.1002 | 752.1868 |
b (L/mg) | 6.0167E-04 | 0.0030 | |
R2 | 0.7009 | 0.7915 | |
SS | 0.0059 | 0.0321 | |
Freundlich | KF | 0.0474 | 2.2086 |
n | 0.9994 | 0.9999 | |
R2 | 0.9501 | 0.9910 | |
SS | 0.4544 | 1.4986 |
Source: own elaboration
Where: qmax (mg g-1) is the maximun adsorption capacity, b (L mg-1) is the Langmuir’s constant related with heat of adsorption, kF (mg g-1 (L mg-1)1/n)and n are the Freundlich’s rate constants refered to the adsorption capacity and adsorption intensity, respectively.
It can be said that active sorption sites are initially occupied by strong bonds, and this strength decreases as they are occupied by ions 36. The kf is higher when MWC is used as adsorbent, which is evidence that the selectivity of the active centers of that adsorbent for tartrazine is higher than those of WC. The values of Freundlich's constant n are close to 1. Then it is assumed that the chemical bonds formed between the adsorbents and the dye are strong, which could be due to the diversity of the active centers of the biomaterials 37.
Parameters From the analysis of the Langmuir model, the parameter "b" is higher when using MWC; this indicates that MWC presented higher affinity for the pollutant, the values of b ranged between 0 and 1, which indicates favorability of the process; as well as a higher adsorption capacity according to the qmax38. Different results have been previously reported for Langmuir's qmax parameter, obtaining values of 20.83 mg/g with activated carbon from mandioca peels 34, 24.57 and 21.59 mg/g with activated carbon from nutshells modified with phosphoric acid and potassium hydroxide, respectively 39, 617.8 mg/g on chitosan/polyaniline compound 19, 59.79 mg/g with iron oxide nanoparticles 8, 180 mg/g with methyltrioctylammonium chloride-modified cellulose nanosponges 16 and 40.79 mg/g with hexadecyltrimethylammonium bromide modified bentonite 9. It is observed that the results obtained in the present study when using WC are in the range of 20-617 mg/g reported in the literature, with the qmax of MWC being well above these values. This is due to the large surface area of this kind of adsorbent, especially after chemical and/or thermal modification.
4. Conclusions
The present study concluded that: (1) The prepared adsorbents present a diverse structure, with multiple absorbance bands of functional groups such as OH, carboxyl, amino and hydrocarbon compounds; band deformation was evidenced after adsorption, which is attributed to the presence of the dye in the bioadsorbent. (2) Wheat residues were found to require quaternization for use in tartrazine removal. (3) Increasing the adsorbent dose and initial concentration positively affects the removal efficiency, achieving 97.65 % removal with MWC. (4) Adsorption kinetics showed that equilibrium was reached at 480 min when MWC and WC were used. (5) Adsorption isotherms on MWC and WC were fitted by the Freundlich model, showing that the removal occurs in multilayers due to chemical interactions. (6) CTAC-modified wheat cellulose is a good adsorbent of tartrazine in aqueous solution.