Servicios Personalizados
Revista
Articulo
Indicadores
-
Citado por SciELO
-
Accesos
Links relacionados
-
Citado por Google
-
Similares en SciELO
-
Similares en Google
Compartir
Boletín Científico. Centro de Museos. Museo de Historia Natural
versión impresa ISSN 0123-3068
Bol. Cient. Mus. Hist. Nat. Univ. Caldas vol.17 no.2 Manizales jul./dic. 2013
PRODUCTION, ACCUMULATION, AND DECOMPOSITION OF LEAF LITTER IN A COLOMBIAN SUBANDEAN FOREST AND NEIGHBORING AREAS OF RESTORATION
PRODUCCIÓN, ACUMULACIÓN Y DESCOMPOSICIÓN DE HOJARASCA EN UN BOSQUE COLOMBIANO SUBANDINO Y ÁREAS ALEDAÑAS DE RESTAURACIÓN
Kelly Rubiano-Cardona1, Luisa Fernanda Arcila-Cardona1, Elizabeth Jiménez-Carmona1 and Inge Armbrecht1
* FR: 19-XI-2012 FA: 18-VII-2013
1 Departamento de Biología, Universidad del Valle, A.A. 25360, Cali, Colombia. Corresponding author: Inge Armbrecht. Address: Universidad del Valle, Dept. Biología. Calle 13 No. 100-00 Ed. 320. Of. 3025, Cali, Colombia. Phone number: 57(2) 3393243. FAX: 57(2) 3392440. E-mail: inge.armbrecht@correounivalle.edu.co
Abstract
Production, accumulation, and decomposition of leaf litter constitute an important entry route for nutrient cycling in ecosystems, but deforestation and soil productive exploitation can affect them. This study evaluated the dynamics of leaf litter in an Andean rural livestock landscape where, since 2003, restoration took place in four biological corridors connecting two forest fragments. The study comprised three periods and 11 sites classified into four types of vegetation cover: (1) forest; (2) pasture; (3) restored areas from pastures (RA-pasture); (4) restored areas from harvested pine and cypress forest plantations (RA-forestry). Methods involved 63 leaf litter collecting traps; 288 mulch samples; 192 decomposition bags, and five environmental variables measured. The environmental conditions of the RAs were similar to those of the forest and differed from that of the pasture. Production was highest in the forest. Accumulation in forests was similar to RA-forestry, but different to that of the pastures and RA-pasture. Decomposition was rapid (> 70% at 45 days) and greater in the RAs than in forests and pastures. Recovery of recycling processes is still far from reaching complete restoration, with RA-forestry being closest. Physical factors (precipitation, gravity) seem to play an important role in the dynamics in all the vegetation covers of this mountainous landscape.
Key words: ecological restoration, montane forests, nutrient cycling, organic matter, primary productivity.
Resumen
La producción, acumulación y descomposición de hojarasca constituyen una vía de entrada importante para el ciclaje de nutrientes en los ecosistemas, pero el aprovechamiento productivo del suelo y la pérdida de cobertura arbórea pueden afectarlas. Este estudio evaluó las dinámicas de hojarasca en un paisaje rural ganadero Andino, escenario en el 2003 de esfuerzos de restauración para conectar dos fragmentos de bosque a través de cuatro corredores biológicos. El estudio se realizó durante tres periodos en 11 sitios clasificados en cuatro tipos de coberturas vegetales: (1) bosque; (2) pastizal; (3) áreas restauradas a partir de pastizales; (4) áreas restauradas a partir de plantaciones forestales de pino y ciprés. Los métodos involucraron 63 trampas colectoras de hojarasca; 288 muestras de mantillo; 192 bolsas de descomposición y cinco variables ambientales medidas. Las condiciones ambientales presentes en las áreas restauradas fueron similares a las del bosque y diferentes a las del pastizal. La producción fue más alta en el bosque. La acumulación en los bosques fue similar a la encontrada en áreas restauradas a partir de plantaciones forestales, pero diferente a la de pastizales y áreas restauradas a partir de pastizales. La descomposición fue rápida (> 70% a los 45 días) y mayor en las áreas restauradas que en los bosques y pastizales. Los procesos de ciclaje aún permanecen lejos de alcanzar la restauración completa, siendo las áreas restauradas a partir de plantaciones forestales las que más se aproximan. Los factores físicos (precipitación, gravedad) parecen jugar un rol importante en las dinámicas de hojarasca de todas las coberturas vegetales presentes en este paisaje montañoso.
Palabras clave: restauración ecológica, bosque montano, ciclaje de nutrientes, materia orgánica, productividad primaria.
INTRODUCTION
Fall and decomposition of leaf litter constitute an important entry route for nutrient cycling in land ecosystems (RAI & PROCTOR, 1986). Both the amount and origin of leaf litter have an important relation with the formation of soil and maintenance of its fertility; thereby, quantification of its production and nature are important approaches to understand nutrient cycling in ecosystems. As leaf litter is produced and its fall occurs, it starts accumulating on the soil to originate the mulch. BEARE et al. (1995) call the mulch "detritosphere", given that it constitutes a complex habitat and one of the most important strata. The size of the mulch is determined in the decomposition through the balance between two contrasting processes: humidification and mineralization; in the first process, leaf litter partially decomposes and maintains adequate mulch levels over the soil (LAVELLE et al., 1993). In the second, the mulch's organic matter decomposes through the action of some soil organisms upon its most elemental components and it is eventually absorbed by plants in different ionic forms, ensuring the flow of energy and nutrients between the soil and the vegetation (VARELA et al., 2007).
In general terms, the dynamics of leaf litter (production, accumulation, and decomposition) is regulated by multiple biological factors like age of trees, plant species, ontogeny, composition of the forest, and soil conditions. It is also affected by weather factors like rain regime, temperature, and humidity, among others. Hence, although tendencies and general ecological mechanisms exist to predict these dynamics, it is difficult to obtain a holistic view of the process applicable to all zones, given that the characteristics of the geographic space in which research is being developed determine results that deviate from the predictions (CLARK et al., 2001). Loss of native tree cover and the soil productive exploitation can affect fauna and micro-soil organisms, simplifying the trophic networks and altering the ecological processes related to the dynamics of leaf litter and flow of nutrients in Tropical ecosystems (LAVELLE et al., 1993; MURGUEITIO et al., 2011). The Colombian Andes, one of the zones with the greatest endemism and biodiversity in the world, has lost more than 60% of the forest cover and its landscape is dominated by agricultural systems (ETTER & WYNGAARDEN, 2000). Particularly, the rural livestock landscape from the Barbas River (Filandia-Quindío) is not the exception. Given the fragmentation and isolation of the cloud forests, on December 2003, Colombian conservation entities began an ecological restoration of three areas seeking to improve the connectivity of the landscape. These three areas had been previously used for commercial exploitation of pine and cypress (Pinus and Cupressus). In June 2005, three additional areas began to be restored; this time their prior use was intensive cattle ranching without trees (VARGAS et al., 2009). The soils of the areas subjected to the restoration process (hereinafter restored areas, RAs) had previously remained under production systems for over 50 years. This study was carried out in 2010, after seven years of having started the restoration process and given that the native cover of the restored areas was noted highly recovered. The study was aimed to revise the processes related to the dynamics of leaf litter in four different vegetation covers: (1) forests, (2) pastures, (3) restored areas from forest plantations (RA-forestry), and (4) restored areas from pastures (RA-pasture).
METHODS
Study area
The landscape of the Barbas River Canyon is located on the western slope of Colombia's Central Mountain range, between coordinates 75°35'42''W; 4°40'48''N and 75°39'38''W; 4°42'47''N (Fig. 1) at the municipalities of Filandia (Department of Quindío) and Pereira (Department of Risaralda). The elevation is between 1700 and 2000 masl, with average yearly precipitation of 2515 mm, and mean temperature of 17 °C. The landscape contains native vegetation characteristic of Subandean "orobiome" from the humid Tropical "zonebiome" (RODRÍGUEZ et al., 2004). Livestock for milk production is the main production activity, followed by commercial forestry of introduced species (pine and cypress). Specifically, the rural livestock landscape from the Barbas River (Filandia-Quindío) is composed of two big native forest fragments; the forest from the Barbas River Canyon that covers about 790 ha and the Bremen Forest Reserve that has 336 ha of native forest and 447 ha of remaining gymnosperm forest plantations (Fig. 1).
The end result of the ecological restoration during these years (2003 and so on) involved the conformation of three connection corridors between the two forest fragments. Therefore, the whole connected structure involves three types of vegetation covers: forest fragments, riparian forests stemming from both fragments and restored areas (Fig. 1), all of them immersed in a pasture-dominated landscape.
To develop the objectives of the present study, we evaluated 11 sites classified into four types of vegetation cover: (1) forests, (2) pastures, (3) restored areas originating from open pastures of African introduced grasses (RA-pasture), and (4) restored areas originating from harvested gymnosperm forest plantations (RA-forestry). For each of the preceding four vegetation covers, three replicates were used, except for the forest, where only two were accessed.
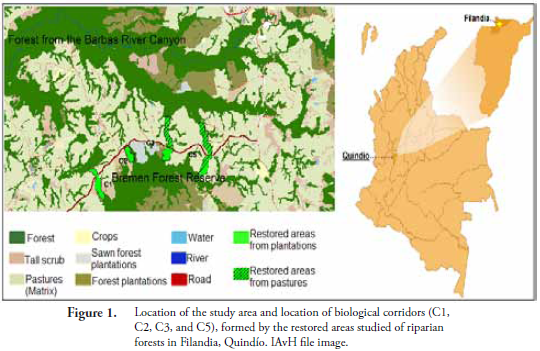
For the purpose of this study, the forest is considered as positive control, given that it is the ecosystem of reference use for the restoration. The pastures were considered negative control because they were the cover with the least native vegetation.
Environmental variables
A 150 m long transect was established per study site and in each of the 12 stations distributed in the first and final 50 m of the transect. Six data sets were taken of leaf litter depth (with a rigid ruler) and tree canopy cover (with a GRS densitometer). Five soil compaction registries were taken using a pocket penetrometer, and two temperature and relative humidity measurements were obtained by using an Extech Hygrometer. All the variables were taken at the start of the study.
Production of leaf litter
Leaf litter collecting traps were constructed with a 0.25 m2 collection area, elaborated in plastic mesh fabric with a 1 cm2 mesh opening. The traps were placed approximately 30 cm from the soil surface. In total, 63 leaf litter collecting traps were used, that is 21 traps to characterize each of the three vegetation covers (pastures were not sampled because leaf litter falls below this height). Seven traps were located per replicate (n = 3) along a random transect (except for forest with ten and eleven traps per transect), with at least 15 m between traps and 10 m from the border. The collecting traps were inspected in a quarterly frequency, during six months. Each of the samples was carefully washed with tap water, dried at 60 °C until constant weight, and weighed on an analytic scale (modified from SUNDARAPANDIAN & SWAMY, 1999). Collection of mulch
Twenty-four samples were collected in each of the four covers (eight samples x three replicates), each sample from a 20 x 20 cm surface of the leaf litter layer, for a total of 96 samples of mulch per quarterly sampling (24 samples x four covers = 96 samples) and 288 samples in total at the end of the six months (96 samples/sampling x three samplings = 288 samples). These samples were taken from the areas near the leaf litter collecting traps and kept in plastic bags until their destiny in the entomology laboratory at Universidad del Valle, Cali. Each of the samples was carefully washed with tap water to remove excess dirt or mud, dried at 60 °C to constant weight, and weighed in an analytic scale (modified from SUNDARAPANDIAN & SWAMY, 1999).
Decomposition of leaf litter
Bags (20 x 20 cm) were elaborated in greenhouse shade netting at 70%. Each bag received five grams of dry weight of "cordoncillo" foliage (Piper anisatum) and five grams of "kikuyu" grass (Pennisetum clandestinum). The plant material was previously furnace dried at 70 °C to constant weight (VARELA et al., 2007). Both, "cordoncillo" and "kikuyu" are relatively abundant in the study zone. Forty-eight bags per vegetation cover were placed, 16 per replicate (except for the forest cover with 24 per replicate), half of them were added one naphthalene ball (control bags with exclusion of fauna) and the remaining without it (treatment bags). Naphthalene has been used in other studies to keep soil fauna from entering the decomposition bags (SEASTEDT & CROSSLEY, 1983; HENEGHAN et al., 1998) and in the present study this was also the initial intention. The bags were located perpendicular to the transect with a 10 m separation between bags with and without naphthalene. Half the bags were gathered at random (four bags with naphthalene and four without naphthalene) at 45 days (T1) and the remaining at 90 days (T2) after their installation. The bags were carefully cleaned, and the leaf litter was deposited into a Berlese funnel for 12 hr. The extracted associated fauna was preserved in 96% ethanol. Leaf litter from each bag was dried at 70 °C to constant weight and the decomposition percentage was calculated through relation between the initial and final dry weight (ILANGOVAN & PALIWAL, 1996).
Statistical Analyses
Environmental variable data were analyzed through Principal Components Analysis (PCA). To determine if the biomass lost in the bags (decomposition), leaf litter biomass produced, and accumulated leaf litter biomass were different among the covers through time, non-parametric tests were performed given that the data did not comply with the assumptions of homogeneity of variance and normality. Kruskal-Wallis (K-W) tests and Mann-Whitney U were used for multiple and pairwise samples respectively. A multiple comparison test was performed when significance was found after K-W. The leaf litter accumulation data complying with the assumptions of the design normality and homoscedasticity were analyzed via Two Way Analysis of Variance (ANOVA), previous natural logarithm transformation of the data and followed by a Tukey test (ZAR, 1999). The ANOVA tests were performed twice: one of them comparing forest vs. RA-pasture vs. pasture, and the other one comparing forest vs. RA-forestry vs. pasture. This is because the restoration ages are different. The R program version 2.14.1 was used for all the statistical tests employed in this study.
RESULTS
Environmental variables
The PCA divided the measured environmental variables points into two classes. On the one hand, on the negative side of the first factorial axis was class one comprising 88 measurement points, 24 taken in the forest, 36 in the restored areas from forest plantations, and 28 in restored areas from pastures. On the other hand, on the positive side of this same axis was class two with 44 points, most of them registered on pastures (36) and eight in restored areas from pastures (Fig. 2).
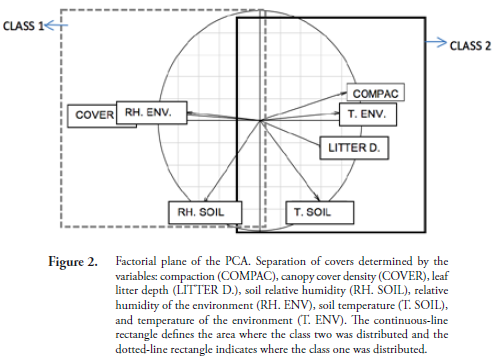
Class one was characterized for having 74.34% average canopy cover, 86.91% soil relative humidity, and 86.31% relative humidity of the environment. Class two had a compaction average of 1.07 kg/cm2, average soil temperature of 24.18 °C, environmental temperature of 23.02 °C, average leaf litter depth of 5.47 cm and all of the measurements for this class registered in pastures had no tree cover.
Production of leaf litter
The greatest mean production of leaf litter, at the end of six months and during each of the sampling periods, occurred in the forest. These values were extrapolated to one year, assuming a repeated weather behavior for the rest of the year (Table 1). During the study year, 2010, there were strong and massive rain falls, causing floods, landslides and mudslides throughout the country.
Statistically significant differences were found between the production of leaf litter occurring in the forest and in the RA-forestry at 90 days (U = 1367; p < 0.00; n = 84). Likewise, this test showed significant differences between said covers at 180 days (U = 371; p < 0.00; n = 84). Production in the forest was significantly higher than that of the RA-pasture, at 90 days (U = 337; p = 0.00; n = 84) and at 180 days (U = 350; p = 0.00; n = 84) (Table 1).
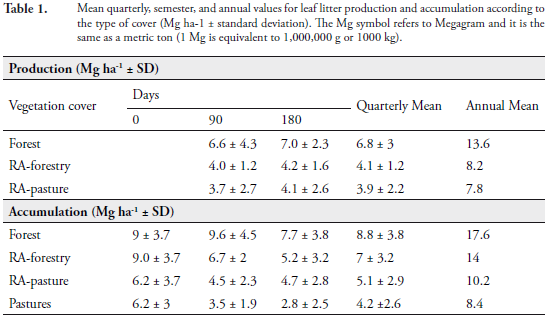
Accumulation of leaf litter
The mean accumulation of leaf litter mulch was always higher in the forest; this habitat also showed the greatest variation. A two-factor ANOVA (vegetation cover and time) among forest, RA-forestry, and pastures indicated that the weight of the mulch was affected by each of the factors (Coverage F[2,96] = 63.72, p = 2.00 E-16; Time F[2,96] = 21.24, p = 4.08 E-09) and by the interaction between them (F[4,288] = 4.06, p = 0.00). Given that the interaction was significant, the comparison of the means with the "coverage" factor may be overshadowed by this interaction; hence, the time factor was left fixed and a Tukey multiple comparison test was employed to observe differences in function of the accumulation, at 0, 90, and 180 days (Table 2). No significant differences were found in leaf litter accumulation between the forest covers and RA-forestry (p = 1.00 > 0.05), while such restored areas and the pastures did present differences in their mean accumulation (p = 0.02 < 0.05). Also, differences among the controls were evident (p = 0.02 < 0.05). Nevertheless, the mean accumulation of leaf litter differed among all the covers during the second sampling (Table 2).
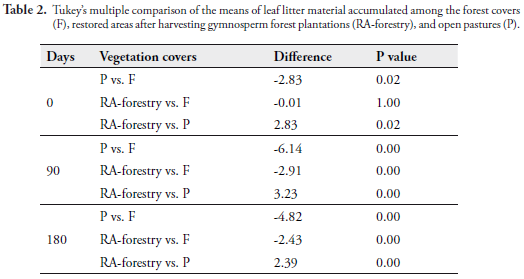
Another two-factor ANOVA this time comparing forest, RA-pasture, and pastures, found influence of each factor on the accumulation of leaf litter (Cover F [2,96] = 51.94, p = 2.00 E-16; Time F[2, 96] = 10.80, p = 3.44 E-05), and also interaction between both factors (F [4,288] = 3.69, p = 0.01).
Results of Tukey's multiple comparison test, leaving the time factor fixed in each of samplings (Table 3) showed that, at 0 and 90 days, the mean accumulation of leaf litter between RA-pasture and pastures was not different, while the forest mean was significantly different (higher) from the mean of RA-pasture in all the samplings.
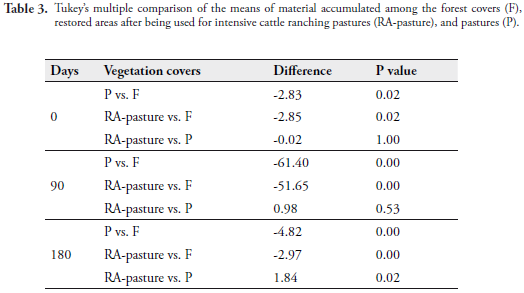
Decomposition of leaf litter
At 45 days (Time 1) most of the leaf litter was lost in all the decomposition bags (above 70% in each cover). The leaf litter remaining in the bags evidenced advanced trituration, so that both plant species litter were not visibly differentiated (P. anisatum and P. clandestinum). At 90 days (T2), the percentages of average decomposition of the covers increased, but not as notorious as previously (Table 4).
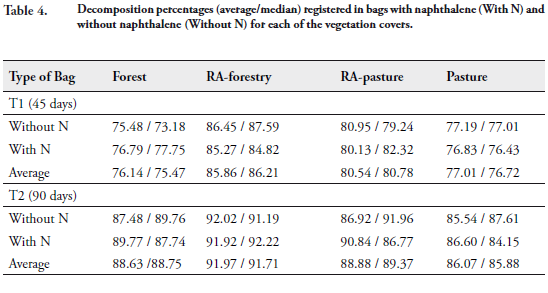
Naphthalene did not exclude soil fauna from the decomposition bags under the climatic conditions of the study zone. The number of individuals of soil fauna in bags with and without naphthalene was the same (554 individuals). The mass lost in both treatments was not statistically different (U-Mann Whitney p > 0.05).
The order of the vegetation covers, in terms of highest to lowest percentage of decomposition was the same during both times evaluated. RA-forestry showed the highest decomposition percentages averages (T1: 85.86% and T2: 91.97%), followed RA-pasture, then the forest, and lastly the pastures (T1: 77.01% and T2: 86.07%) (Table 4). The Kruskal-Wallis tests and respective pos Hoc (multiple comparison test), demonstrated that the biomass lost at 45 and 90 days in RA-forestry was statistically different from the mass lost in forests and pastures. A similar trend was found with respect to restored areas from pastures, at 45 days the biomass lost in this cover differed from that found in forests and pastures; however, at 90 days these differences were no longer maintained.
DISCUSSION
An important attribute generated by ecological restoration is the recovery of native vegetation in the restored ecosystems (KATTÁN & NARANJO, 2007). VARGAS et al. (2009), indicated that in restoration processes based on acceleration of succession (the technique used to restore the study zones), a tree cover is expected to be formed, and habitat conditions such as environmental variables, leaf litter production, accumulation, and decomposition should become more similar to the reference forest ecosystem. The results obtained with the environmental variables, partially agree with that stated by these researchers (VARGAS et al., 2009). Seven years after restoration showed a tree canopy cover above 60% in the RAs and their abiotic conditions are becoming similar to that found in the forest.
However, the data obtained for leaf litter dynamics did not show the same tendencies obtained with the environmental variables. The significant differences found in leaf litter production between the forest and each of the restored areas reflect that the short period or age since restoration has not been sufficient for the recovery of this process. LEBRET et al. (2001) reported that leaf litter production increases in positive relationship with the age of the vegetation. The aforementioned is evidenced in the greatest leaf litter production registered in RA-forestry, which are one year older than RA-pasture. Under this scenario, the productivity of leaf litter in restored areas could hardly reached the forest productivity, at least in the short and midterm. Nonetheless, it is highlighted that the values of leaf litter production in these restored areas were within the range of leaf litter production reported for Tropical forests (JORDAN, 1983; TAKEDA, 1996; CLARK et al., 2001).
The different vegetation covers in this study were located at the same altitude over sea level. Various authors accept the relationship the altitudinal gradients have over leaf litter production, coinciding in that this production diminishes upon increasing altitude (BRAY & GORHAM, 1964; VENEKLAAS, 1991; SUNDARAPANDIAN & SWAMY, 1999). Hence, the value of yearly production registered in this study for the forest (13.6 Mg ha-1 year-1) is considered high, considering that it is a high-land montane forest. According to BROWN & LUGO (1990) although PROCTOR (1983) states that some high values corresponding to leaf litter production are the result of extrapolations from measurements made over a short period, during a peak in leaf litter productivity; it is known that leaf litter production in secondary forests occurs in accelerated manner during the first 12-15 years, establishing a production limit around 12-13 Mg ha-1 year-1, independent of the climatic conditions (BROWN & LUGO, 1990).
Regarding leaf litter accumulation, the significant differences found upon comparing RAs, to forests and pastures during the second and third sampling indicate that although these restored areas do not yet reach the forest's accumulation levels, their accumulation is distinct from what occurs in pastures, suggesting that said areas are in transition to recovery (Table 2). Also, the lack of marked differences between the accumulation from the forest and the RA-forestry suggests that the biomass accumulation permits the formation of mulch which presumably facilitates the circulation of nutrients between the soil and the plant (Table 2).
Lack of significant differences in mean leaf litter accumulation between the RA-pasture and pastures may be due to the lesser time since restoration to stabilize their ecosystem processes, given that the RA-forestry, which are one-year older, showed differences with the pastures. Nevertheless, the historical use of the current restored areas for pastures and intensive cattle- ranching could be considered a hidden treatment as the cause of decreased rate of recovery, given that an ecosystem's capacity and ease of recovery will depend on the degree of degradation (GÁLVEZ, 2002). The fact that harvested gymnosperm forests were restored one year before the pasture lands makes it impossible for us to separate the effects of history and type of previous land use. BROWN & LUGO (1990), after comparing several studies, indicated that the time for a secondary forest to reach biomass accumulation levels similar to those present in mature forests is of 40-50 years, independent of the intensity of the land use.
Regarding leaf litter decomposition, lack of differences between both controls (forest and pasture) suggest that the mass loss registered in the decomposition bags may be due to a mechanical triturating effect affecting all the covers in like manner and not one decomposition per se that depends on soil organisms associated to each vegetation cover in particular. Two direct pieces of evidence additionally support this interpretation. On one side naphthalene did not affect the lost leaf litter biomass nor were a great amount of organisms found in the bags. On the other, high precipitation (1508 mms year -1) occurred during the study (IDEAM, 2011) and this factor joined to others like leaching and runoff could have affected all the covers causing the leaf litter to get out of the bags due mainly to comminuting of the material. Biomass loss registered in this research was generally high compared to other montane Tropical forests, again suggesting mechanical triturating as an important factor. VARELA et al. (2007) for example, in a cloud forest to the southwest of the Bogotá Sabana in Colombia, obtained 60% decomposition after 365 days of experimenting with an initial 10 g of Agropyron cristatum, just 10% less than what was obtained in this research in only 45 days. In 2004, these same researchers registered decomposition percentages at 55% during the same time interval (VARELA et al., 2007). Likewise, RAVE et al. (2013), in a low montane forest located also in Quindío, reported over 60 days decomposition percentages below 30% by using Juglans neotropica leaf litter.
PRESCOTT (2005) indicated that it is necessary to ponder why and how have the decomposition rates been measured. For example, it is difficult to ensure, with the method of decomposition bags, that the leaf litter disappearing is really leaf litter that decomposes; nonetheless, this is the most frequent way how the data are erroneously interpreted, reaching misleading conclusions. Based on the previously stated, the results from this study suggest that biological activity was not the main factor causing the lost of leaf litter. It may rather be stated that the dynamics of leaf litter loss is accelerated and that an effective flow of material takes place, whether incorporated to the soil, leached out of the system through runoff, or migrating to other uses of the soil.
In summary, it is suggested that ecological restoration in the studied landscape has until now managed to recover part of the processes that support proper functioning in terms of leaf litter dynamics. Considering the short period elapsed since the restoration to date, it is highlighted that important ecological characteristics have been recovered in the RA-forestry that permit and favor adequate flow of nutrients in these ecosystems. In general terms, although still far from the goal, that is, the forest ecosystem, the ecological attributes evaluated in the restored areas, especially the RA-forestry, evidences an appropriate trajectory towards the native subandean tropical forest.
IMPLICATIONS FOR PRACTICE
After seven years, the restored Andean areas resemble native forests in environmental conditions but the recycling processes are still far from reaching complete restoration.
The leaf litter bag method for studying decomposition may hide operating mechanical effects, or hidden treatments (precipitation, gravity) that may conduce to misinterpretation of decomposition dynamics.
ACKNOWLEDGMENTS
The authors thank O. Ascúntar, J. Celis, O. Mejía, C. Solís, E. Soto, and J. Herrera for help in field and laboratory; to the farms owners; to Universidad del Quindío and workers at Bengala farm. Corporación Autónoma Regional del Quindío (CRQ) conceded the study permission # 720. The Research Vicerrectory, Univalle financed translation to English. This Research was funded by Universidad del Valle and the Environment and Habitat Program of COLCIENCIAS Code 1106-489-25496, Contract 443-2009.
BIBLIOGRAPHY
BEARE, M.H., COLEMAN, D.C., CROSSLY, D.A., HENDRIX, P. & ODUM, E.P., 1995.- A hierarchical approach to evaluating the significance of soil biodiversity to biogeochemical cycling. Plant and soil, 170: 5-22. [ Links ]
BRAY, J.R. & GORHAM, E., 1964.- Litter production in forest of the world. Advances in Ecological Research, 2: 101-157. [ Links ]
BROWN, S. & LUGO, A.E., 1990.- Tropical secondary forests. Journal of Tropical Ecology, 6: 1-32. [ Links ]
CLARK, D.A., BROWN, S., KICKLIGHTER, D.W., CHAMBERS, J.Q., THOMLINSON, J.R., NI, J. & HOLLAND, E.A., 2001.- Net primary production in tropical forest: An evaluation and synthesis of existing field data. Ecological Applications, 11: 371-384. [ Links ]
ETTER, A. & WYNGAARDEN, W.VAN., 2000.- Patterns of landscape transformation in Colombia, with emphasis in the Andean region. Ambio, 29: 432-439. [ Links ]
GÁLVEZ, J., 2002.- La restauración ecológica: conceptos y aplicaciones. Revisión bibliográfica. Universidad Rafael Landívar, Facultad de Ciencias Ambientales y Agrícolas. Instituto de Agricultura, Recursos Naturales y Ambiente. Serie de Documentos Técnicos No. 8. 21p. [ Links ]
HENEGHAN, L., COLEMAN, D.C., ZOUB, X., CROSSLEY, D.A. & HAINES, B.L., 1998.- Soil micro arthropod community structure and litter decomposition dynamics: a study of tropical and temperate sites. Applied Soil Ecology, 9: 33-38. [ Links ]
IDEAM, 2011.- Registros medios mensuales multianuales de precipitación. Estación Salento. Instituto de Hidrología, Meteorología y Estudios Ambientales, Bogotá D.C., Colombia. [ Links ]
ILANGOVAN, M. & PALIWAL, K., 1996.- Changes in mass and nutrient during decomposition of Leucaena leucocephala and Cymbopogon citrates and the effect of substrate quality. Weather variables on mass loss during decomposition in a semi-arid ecosystem, Madurai, India. Journal of Tropical Forest Science, 8: 317-332. [ Links ]
JORDAN, C.F., 1983.- Productivity of tropical rain forest ecosystems and the implications for their use as future wood and energy sources: 117-135 (in) GOLLEY, F.B. (ed.) Ecosystems of the World, Vol. 14A, Tropical Rain Forest Ecosystems: Structure and Function. Elsevier, Amsterdam, Netherlands. [ Links ]
KATTÁN, G. & NARANJO, G., 2007.- Regiones biodiversas: herramientas para la planificación de sistemas regionales de áreas protegidas. Fundación EcoAndina WCS/WWF, Bogotá D.C., Colombia. [ Links ]
LAVELLE, P., BLANCHART, E., MARTIN, A. & MARTIN, S., 1993.- A hierarchical model for decomposition in terrestrial ecosystems: application to soils of the humid tropics. Biotropica, 25: 130-150. [ Links ]
LEBRET, M., NYS, C. & FORGEARD, N., 2001.- Litter production in an Atlantic beech (Fagus sylvatica L.) time sequence. Annuals of Forest Science, 58: 755-768. [ Links ]
MURGUEITIO, E., CALLE, Z., URIBE, A. & SOLORIO, B., 2011.- Native trees and shrubs for the productive rehabilitation of tropical cattle ranching lands. Forest Ecology and Management, 261: 1654-1663. [ Links ]
PRESCOTT, C., 2005.- Do rates of litter decomposition tell us anything we really need to know?. Forest Ecology and Management, 220: 66-74. [ Links ]
PROCTOR, J., 1983.- Tropical forest litter fall. I. Problems of data comparison: 267-273 (in) SUTTON, S.L., WHITMORE, T.C. & CHADWICK, A.C. (eds.) Tropical rain forest: ecology and management. Blackwell Scientific Publications, Oxford, U.K. [ Links ]
RAI, S.N. & PROCTOR, J., 1986.- Ecological studies on four rainforests in Karnataka, India. II. Litterfall. Journal of Ecology, 74: 455-463. [ Links ]
RAVE, S., MONTENEGRO, M. & MOLINA, L. J., 2013.- Caída y descomposición de hojarasca de Juglans neotropica Diels (1906) (juglandaceae) en un bosque montano andino, Pijao (Quindío), Colombia. Actualidades biológicas, 35: 33-43. [ Links ]
RODRÍGUEZ, N., ARMENTERAS, D., MORALES, M. & ROMERO, M., 2004.- Ecosistemas de los Andes Colombianos. Instituto de Investigación de los Recursos Biológicos "Alexander von Humboldt", Bogotá D.C., Colombia. [ Links ]
SEASTEDT, T.R. & CROSSLEY, D.A., 1983.- Nutrients in forest litter treated with naphthalene and simulated through fall: a field microcosm study. Soil Biology and Biochemistry, 15: 159-165. [ Links ]
SUNDARAPANDIAN, S.M. & SWAMY, P.S., 1999.- Litter production and leaf-litter decomposition of selected tree species in tropical forests at Kodayar in the Western Ghats, India Forest. Ecology and Management, 123: 231-244. [ Links ]
TAKEDA, H., 1996.- Templates for the organization of soil animal communities in tropical forest: 127-226 (in) TURNER, I.M., DIONS, C.H., LIM, S.S. & NG, P.K.L. (eds.) Biodiversity and the dynamics of ecosystems. National University of Singapore, Singapore. [ Links ]
VARELA, A., CORTÉS, C. & COTES, C., 2007.- Cambios en edafofauna asociada a descomposición de hojarasca en un bosque nublado. Revista Colombiana de Entomología, 33: 45-53. [ Links ]
VARGAS, A., LOZANO, F.H., VARGAS, W., ARISTIZÁBAL, S., MENDOZA, E. & CAYCEDO, D.P., 2009.- Seguimiento y evaluación de la estrategia de conservación en el paisaje rural (Fase IV): 161-177 (en) LOZANO-ZAMBRANO, F.H. (ed.) Herramientas de manejo para la conservación de biodiversidad en paisajes rurales. Instituto de Investigación de Recursos Biológicos "Alexander von Humboldt" y Corporación Autónoma Regional de Cundinamarca (CAR), Bogotá D.C., Colombia. [ Links ]
VENEKLAAS, E.J., 1991.- Litterfall and nutrient fluxes in two montane tropical rain forests, Colombia. Journal of Tropical Ecology, 7: 319-336. [ Links ]
ZAR, J.H., 1999.- Biostatistical analysis. Prentice Hall, New Jersey, USA. [ Links ]