Introduction
The poultry sector is one of the foundations of the Colombian economy and diet. The majority of the country's poultry farms are found in the departments of Cundinamarca (28%), Santander (24%) and Valle del Cauca (13%) (Escalante et al. 2010). The different poultry farming processes generate waste such as laying-hen excreta, chicken excreta, dead birds, fatty waste and eggshells. This waste causes environmental contamination, public health and animal health problems (Delgado, 2011). Caged laying hen excreta (CLHE), a mixture of liquid and solid animal excreta, is the most representative organic waste generated by poultry farming because of its volume, as well as its physiochemical properties (Rosales et al. 2007). During the industrial process of specialized rearing of farm birds, an average quantity of 107.98 tons of CLHE is generated per one million birds (Escalante et al. 2010). Through biological and thermal processes, it is possible to use the byproducts of poultry processes in the preparation of new products such as poultry manure and meat meal (Huang et al. 2011; Rosales et al. 2007). However, these technologies are insufficient to treat the volumes of waste generated by the sector. CLHE is mainly comprised of protein (20.5 %), cellulose (20 %), hemicellulose (23.2 %), lignin (1.6 %) and volatile fatty acids (VFAs) (0.3 %). This high content of organic matter makes CLHE an attractive substrate for anaerobic digestion (AD) (Li et al. 2013). Anaerobic bioconversion systems are biological processes where organic material is reduced to methane and carbon dioxide by the activity of different microbial groups that work in syntrophy. The microorganisms involved in AD are comprised of a) fermentative and hydrolytic bacteria b) acetogenic bacteria, and c) methanogens. The first group is represented by the Peptostreptococcus, Propionibacterium, Bacteroides, Micrococcus and Clostridium genera, which produce enzymes that divide the organic polymers into easily fermentable monomers. Then the acidogenic bacteria metabolize the monomers to generate acetate, propionate and butyr-ate, which are later reduced by the acetogenic bacteria to acetate, carbon dioxide and hydrogen. Finally, the methanogens act on the acetate and hydrogen to transform them into methane (Gerardi, 2003). Studies on AD in mesophillic conditions have reported yields of methane production from 0.29 to 0.50 m3 CH4/kg VS, using CLHE as a substrate and anaerobic slurry from sewage treatment plants as an inoculum (Li et al. 2013; Nui et al. 2013).
An important operational parameter in AD is the organic load represented through the inoculum to substrate ratio (ISR) (Hashimoto et al. 1989). It has been demonstrated through experiments that the ISR affects the speed of the substrate's biodegradation. Therefore, this parameter allows the biomethane potential (BMP) to be specified and comparable AD studies to be conducted for any kind of substrate (Raposo et al. 2011). The first studies related to the influence of organic load on BMP in terms of ISR were carried out in discontinuous operation using corn silage as a substrate and bovine slurry as an inoculum in volume concentrations of 0% (control), 10%, 30%, 50%, 70% and 90%. The results show that for high organic loads (ISR values under 0.25), the speed of the substrate's biodegradation was slow compared to the biodegradration of the substrate for ISR values close to 2 (Hashimoto et al. 1989). Similar results were obtained by Raposo et al. (2006) for the AD of the same substrate using bovine slurry as an inoculum at an ISR from 1 to 3.
The influence of the ISR on BMP has also been studied for food and agribusiness waste. The existence of a declining linear relationship between biogas yields and ISR was proven (Liu et al . 2009). Neves et al . (2004) confirmed that for the AD of food waste using granular slurry and suspended slurry as an inoculum, the selection of the ISR becomes a greater determinant than the inoculum's own metabolic activity. Therefore, studies on AD require that an organic load is established based on an ISR, which permits the initiation and stabilization of the process.
The criteria to establish an ISR value revolve around the complexity of the substrate's biodegradation. In the case of CLHE, concentrations of volatile fatty acids (VFAs) between 2000-4800 mg/L and alkalinity (TA) between 4,700-13,600 mg/L allow adequate initiation of the anaerobic process (Salminen and Rintala, 2002; Callaghana et al. 2002). However, CLHE also contains ammoniacal nitrogen, which in concentrations above 5,000 mg/L, has a toxic or inhibitive effect on microbial activity, affecting the stability of the process (Nue et al. 2013).
Owen et al. (1979), established that ISR values of more than one allow the dilution of the concentration of toxic compounds present in the substrate. Additionally, Chynoweth et al. (1993) observed that for substrates such as cellulose, an ISR of 2.0 is required to prevent inhibition from the elevated accumulation of volatile fatty acids (VFAs). The acidification produced by the low buffer capacity in the anaerobic biodegradation of kitchen waste and the inhibition caused by the release of orthophosphates in the AD of algae were controlled by establishing an ISR of 1.0, allowing the process to be operated in pH values within the appropriate range (6.0-8.5) for AD (Zeng et al. 2010; Neves et al. 2004).
The objective of this work was to assess the influence of the substrate/inoculum ratio on the biomethane potential of CLHE. The development of this objective allows the effect of the substrate's variability on the digestion of waste with a high ammonium content to be established.
Materials and Methods
Substrate and Inoculum The substrate used in the experiment was CLHE collected from a poultry farm in the municipality of Lebrija-Santander, Colombia. The CLHE was kept refrigerated at a low temperature (4 °C) during the characterization and analysis stage. Bovine slurry from a slaughterhouse in Santander was used as the inoculum.
Experimental Design The biomethane potential (BMP) of the CLHE was established by experiment according to the protocol established by Angelidaki et al. (2009). The five organic loads were measured: 16.6 (ISR 1.0), 11.0 (ISR 1.5), 8.3 (ISR 2.0), 6.6 (ISR 2.5) and 5.5 (ISR 3.0) g VS/L, in biodigesters of 60 ml, with a fixed amount of inoculum of 0.58 g VS. To ensure anaerobic conditions, the biodigesters were purged with nitrogen for 2 minutes and sealed with butyl and aluminum stops. The biodegradation trials were carried out three times for 30 days in mesophillic conditions at a temperature of (39 °C±2) in an incubator (BD-53+ RS422 WTB BINDER).
Procedures and Methods of Analysis The volatile fatty acids (VFAs), total alkalinity (TA), pH, NH4 + and volume of accumulated methane were considered to be the response variables of the process. The VFAs were quantified by titration with NaOH (0.1 N) and the TA by titration with HCl (0.1 N) (Anderson and Yang, 1992). The pH was established using a pH meter (Metrohm 691) and the NH4 + according to the 4500-NH3D protocol from the Standard Methods for the Examination of Water and Wastewater (APHA, 2005). The biogas produced during the experiment time bubbled in an alkaline solution (NaOH) with phenolphthalein as an indicator and pH 12, in which CO2 is absorbed. The volume of displaced alkaline solution is equivalent to the volume of methane generated by the anaerobic system (Angelidaki et al. 2009). The methane was quantified in normal temperature and pressure conditions through the equation (1):
Where Vntp is the volume of gas in normal conditions (ml), V is the volume of gas generated during the biomethanation test, P o is the pressure of methane in normal conditions (1013.25 mbar), T is the environment temperature in which the measurement was taken, P is the vapor pressure (mbar) at temperature T, and P atm is the atmospheric pressure of the place in which the test was conducted. The GMP of the CLHE is expressed in terms of normalized accumulated methane volume over volatile solids added from the substrate (VS-Ad). The theoretical biomethane potential (BMP th) of the substrate was calculated through stoichiometry represented in the Buswell equation (2 and 3), which assumes the full conversion of all the organic matter into biogas (Buswell and Neave, 1930).
Where 22.4 is the volume of one mole of gas at standard temperature and pressure conditions. The experiment's results were analyzed with the StatGraphics plus 5.1, StatPoint ® Inc. (Virginia, USA) software. Fisher's exact test was used to verify the statistical differences between the results.
Results
Physiochemical Characterization of the Substrate and Inoculum Table 1 presents the physiochemical characterization of the substrate and inoculum, which was carried out according to the protocols of the Standard Methods for the Examination of Water and Waste-water (APHA, 2005).
The pH of the substrate and the inoculum is within the operating range for the development of AD reported in the literature (Raposo et al. 2006). The organic matter of the substrate represented by the concentration of total solids, volatile solids and volatile fatty acids of the caged laying hen excreta permit its identification as an attractive source of carbon for AD.
Evolution of the Acidogenic Stage of Anaerobic Digestion of Caged Laying Hen Excreta The kinetics of VFA consumption during 30 days of biodegradation is presented for the ISRs of 1.0, 1.5, 2.0, 2.5 and 3.0 (Figure 2). It is observed that for the different inoculum to substrate ratios, the start of the process presents the same exponential trend, indicating the inoculum's affinity toward the substrate (Quintero et al. 2012). It has been demonstrated that VFA concentrations above 6,000 mg/L may cause inhibition of the anaerobic process (Castillo et al. 2007). In this study, the maximum concentration of VFAs was 5,400 mg/L (ISR 1); a value that is below the limit that would cause inhibition by acidification. From the sixth day, the VFA concentration decreased to below 450 mg/L, which demonstrates a high degree of reduction of organic matter and consequently, metabolic activity of the acetogenic microorganisms capable of reducing the VFAs, even acetate (Wang et al. 1999).
Stability of the Anaerobic Process The anaerobic processes achieve stability in VGA/TA ranges from 0.2-0.8 (Raposo et al. 2011). VFA/TA ranges above 0.8 indicate that there is no balance between the population of acidogenic and methanogenic microorganisms (Wan et al. 2011). Figure 2a shows the change in stability of the anaerobic digestion process of laying-hen excreta for different ISRs.
It was demonstrated that the anaerobic digestion process of laying hen excreta is promoted by organic loads below 16 g VS/l, equivalent to ISRs over 1.0. However, at the start of the process, instability was evident in the system for all the ratios studied with VFA/ TA values over 0.8. This is due to the fact that the production speeds of acids are greater than the speeds of consumption of them. Therefore, the VFA/TA ratio is within the stability limits for the experiment with an ISR of 3. Due to the buffer capacity of the inoculum (0.23, see Table 1), the system achieves stability on the ninth day of biodegradation and the VFA/TA ratio maintains a range of 0.0 to 0.3, which is adequate to operate a process in stable conditions. These results coincide with the stability achieved (VFAs/TA = 0.4) for the digestion of bovine slurry and laying hen excreta diluted to 15% (Callaghan et al. 2002).
The pH evolution for the different ISRs is presented in Figure 2b. The pH for the measured ISRs oscillated within a range from 7.5 to 8.5, adequate values for the growth of methanogenic microbial consortia (Gerardi, 2003). During the first three days of the biodegradation process, the pH decreased as a result of increases in the concentration of VFAs. After the fourth day, the pH gradually increased until stabilizing. This behavior can be explained based on that reported by Lay et al. (1998), that the substrates with a high organic load, such as CLHE, tend to present rapid increases in the concentration of VFAs, causing strong drops in pH and destabilizing the reactor. However, progressive increases in ammonium concentration increase the pH, behaving like a base (Lay et al. 1998; Yenigun et al. 2013). Angelidaki et al. (2003) described similar results for systems with different organic waste, specifying that for the case of CLHE, interactions may arise between the production of VFAs and pH, which lead to a state of balance.
Effect of Ammonium Concentration on the Anaerobic Digestion of Caged Laying Hen Excreta Figure 3 shows that the variation in the ammonium concentration for each one of the measured ratios has a similar trend. The ammonium concentrations are proportional to the added organic load. The maximum ammonium concentration (2,066 mg/L) is presented at the start of the process for the ISR of 1.0, which is equivalent to an organic load of laying hen excreta of 16.6 g VS/L. The process stabilizes at the ninth day of digestion, reaching ammonium concentrations that oscillate between 225 mg/L and 550 mg/L. Taking into account that ammonium concentrations above 2,000 mg/L inhibit the anaerobic system, it is observed that the anaerobic digestion of caged laying hen excreta does not present an inhibitory effect from ammonium ions, except for organic loads above 16.6 g VS/l (equivalent to an ISR of 1).
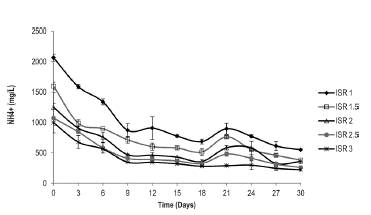
Figure 3 Variation of ammonium concentration in the anaerobic digestion of caged laying hen excreta.
Anaerobic Biodegradability of Caged Laying Hen Excreta According to the element composition, the caged laying hen excreta used in this study (%p/p C:28; H:2.6; O:18.4; N:3.8) theoretically has a biomethane potential (BMP) of 0.59 m3 CH4/kg-VS. The specific methane production of the inoculum (bovine slurry) was 0.17 m3 CH4/kg-VS. This value was subtracted from the respective BMP for each one of the trials (Figure 4). The maximum BMP was 0.58 m3 CH4/ kg-VS for an ISR of 1.0, achieving 98% process efficiency. The lowest methane production was 0.55 m3 CH4/kg VS for the 2.5 and 3.0 ISR values, which correspond to added organic loads of 6.6 g VS/L and 5.5 g VS/L, respectively. The values obtained in this study are greater than those reported by Li et al. (2013), who achieved productions of 0.30 m3 CH4/kg-VS from an organic load of 3.0 g VS/L of caged laying hen excreta. The above indicates that the anaerobic digestion process of caged laying hen excreta presents high yields of methane production.
The comparison of means for the BMP of caged laying hen excreta at the measured ISR (statistical analysis) showed that there are significant differences between each of the experiments with a value of p = 0.0001 (Figure 5). Therefore, it may be confirmed that organic loads of less than 17 g VS/L do not inhibit the anaerobic process of caged laying hen excreta, demonstrating an inverse effect between the organic load of caged laying hen excreta (expressed according to the ISR) and the biomethane potential of this substrate.
Conclusions
The specific production of methane from caged laying hen excreta favors the operation of the process with organic loads below 17 g VS/L . Consequently, inoculum (bovine slurry) / substrate (caged laying hen excreta) ratios above 1.0 reduce the inhibition of anaerobic digestion caused by the accumulation of volatile fatty acids and ammonium ions, given that the maximum concentrations of these compounds are 5,400 mg/L and 2,066 mg/L, respectively. As a result, the biomethane potential of caged laying hen excreta is 0.58 m3 CH4/kg-VS, achieving a process efficiency of 98%.
Given that the anaerobic digestion process is inhibited at organic load concentrations above 17 g VS/l, it is recommendable to focus research with caged laying hen excreta on the co-digestion of this substrate with industrial waste rich in carbohydrates, lipids and proteins, for example sugar cane molasses, residual glycerol and whey.