Introduction
During flooding or waterlogging, the transfer of oxygen and other compounds between soil and the atmosphere is blocked, and the limited dissolved oxygen available in the water is consumed by the plants, soil fauna, and microorganisms until it is completely exhausted (Joshi and Kumar, 2012). When there is low availability of oxygen (hypoxia) or absence of oxygen (anoxia), the normal operation of plants is mainly affected by the decrease in the synthesis of ATP, causing withering of waterlogged plants (Sairam et al. 2008). Despite the energy crisis suffered by the plants due to the deficiency of oxygen caused by flooding, adaptation skills have been discovered in different crop species that allow them to tolerate the anaerobic conditions caused by waterlogging (Nishiuchi et al. 2012). These adaptive skills include anatomic changes such as the formation of adventitious roots with longitudinal interconnections of air-filled cavities called aerenchyma, which permit internal aeration between shoots and roots (Voesenek et al. 2006), or the formation of barriers to prevent radial oxygen loss into the rhizo-sphere (Armstrong et al. 2000). Metabolic changes are also produced, which include a decrease in absorption and assimilation of sources of nitrogen; activation of anaerobic respiration; maintenance of the supply of carbohydrates for anaerobic respiration; control of cytoplasmic acidification; and development of the antioxidant defense system (Chen & Qualls, 2003; Kumutha et al. 2008; Oliveira et al. 2013; Roberts et al. 1984). These changes allow the plants to keep growing and survive in surroundings with low availability of oxygen (Medina et al. 2009).
Oxygen is vital for the cellular energy production, and its presence is crucial for metabolic activities and production of ATP by being the electron acceptor in the pathway of oxidative phosphorylation. Therefore, much of the research on plants has been focusing on the presence and operation of fermentation pathways as a mechanism of metabolic rescue when respiration stops due to anoxia from waterlogging of the soil (Dennis et al. 2000). In plants, the fermentation pathways do not happen under normal oxygen conditions, but their rapid induction from a low supply of oxygen suggests a survival mechanism when there is little oxygenation. The root system is the main target when there is a deficiency of oxygen from waterlogging, because of the different metabolic disruptions that occur in the root tissue, which ultimately, affect the global yield of the plant (Kulkarni & Chavan, 2013). Studies on the root response of corn seedlings to anaerobic treatment have revealed that the normal synthesis of aerobic soluble proteins is reduced, while a set of 20 anaerobic proteins are selectively synthesized, these being identified as enzymes that intervene in glycolysis and in fermentative metabolism (Sachs et al. 1980). Out of this group of anaerobic proteins, the enzyme that catalyzes alcoholic fermentation, alcohol dehydrogenase (ADH), is the most studied, and its greater induction from the effect of anaerobiosis has been demonstrated in species of economic importance, such as Zea mays, Glycine max, Euterpe oleracea, Oryza sativa, Hordeum vulgare, Pennisetum glaucum, Dioscorea cayenensis, Ipomoea batatas and Echinochloa crus-galli (Borella et al. 2014; Fukao et al. 2003; Gaut & Clegg, 1991; Huang et al. 2002; Johnson et al. 1994; Menezes et al. 1995; Oluoha, 1995; Wignarajah et al. 1976). This significant increase in the activity of the ADH enzyme is a considered characteristic of tolerance to stress from excess water. Therefore, it is important to explore metabolic resistance mechanisms in species of economic importance, such as yellow passion fruit, which could be used in crop improvement programs (Roorkiwal & Chand, 2012).
The yellow passion fruit (Passiflora edulis var. flavicarpa Deg) is one of the most sought after fruit crops for consumption in many countries of the world, finding large agricultural crops in Colombia, Venezuela, Ecuador, Peru, Brazil, Hawaii, Taiwan, India, South Africa, Sri Lanka, Kenya, New Guinea and Australia (Potosí et al. 2008). In Colombia in the department of Valle del Cauca, there are 919 planted hectares of yellow passion fruit crops, out of which, 619 hectares (~67%) are in the north of the department in the municipalities of Roldanillo, La Unión and Toro, an area called the RUT District. This area of Valle del Cauca comprises a large part of the valley of the Cauca River, and it has a large capacity for agricultural production with flat and fertile lands. It presents certain limitations for cultivation because of the climatic variations with periods of drought and flooding, the latter being caused by the Cauca River and its tributaries bursting their banks (Sierra, 2008). Said floods in Colombia, especially those that occurred in the second half of 2010 and again in April 2011 due to the La Niña phenomenon, generated partial and total losses of the yellow passion fruit crops in the area (CORPOICA, 2011). These crops went from having the best yield of passion fruit in the country of 35 ton/ha in 2010 to 8.6 ton/ha in 2011 (National Administrative Department of Statistics, DANE for the Spanish original, 2012). Taking into account the effect of the floods on the yield of the passion fruit crop, it is very important to intensify the studies aimed at designing alternative strategies to maintain the crop in adverse environmental conditions in order to prevent the total loss of passion fruit production.
One of the important pillars to develop this kind of strategy is to understand the mechanisms that the plants have to respond to specific stress; activation of anaerobic metabolism being one of the responses to stress from waterlogging. This study compared the activity of the ADH enzyme in the roots of yellow passion fruit seedlings in conditions of waterlogging and normal risk conditions. The results demonstrate that the activity of the ADH enzyme in the roots of yellow passion fruit seedlings in conditions of waterlogging significantly differs from its activity in roots under normal risk conditions. This significant increase in the activity of the ADH enzyme in roots of yellow passion fruit seedlings subjected to conditions of flooding demonstrates the presence of a possible short-term survival strategy of the passion fruit seedlings in the event of waterlogging.
Materials and Methods
Plant Material and Growing Conditions
Yellow passion fruit (Passiflora edulis var. flavicarpa) seeds from the north of the Valle del Cauca Department were selected and provided by the farmers of irrigation district of Roldanillo, La Unión and Toro (RUT District) in the valley of the Cauca River. The seeds were planted in polyethylene bags measuring 9 by 12 inches with enriched soil substrate treated with TECTO 500 SC fungicide. They were kept for two months with sufficient water supply in the Soil and Water Laboratory (LASA, for the Spanish original) of the Universidad del Valle, Meléndez Campus. After the two months, the seedlings measuring 15 to 20 cm in height were transplanted to individual 12-liter plastic trays with the same soil and watering conditions as described above. At the start of the experiment, 18 three and a half months-old seedlings were taken after they germinated, and nine of the containers were randomly selected for treatment with flooding. This condition was achieved by flooding the container, maintaining the sheet of water 2 cm above the soil level, and the other nine containers were maintained in normal watering conditions. The experiment lasted fourteen days in semi-controlled conditions in the LASA greenhouse. For each recipient in conditions of flooding, the content of dissolved oxygen (O2D) and temperature (°C) of water in each unit of time were monitored using a YSI 550A oximeter.
From these variables, the percentage of oxygen saturation in the water of the soil under the flooding treatment was determined; it showed a decrease throughout the period of the experiment (Figure 1).
Sampling
Samples measuring 2 cm were taken from the apical area of the roots on Day 0, 1, 3, 7, 9 and 14 for both treatments. The root samples of seedlings in the flooding treatment after Day 14 were taken from adventitious roots, except Seedlings 4 and 7, which presented little emergence of said roots (approximately 0.3 cm long on average for each seedling) compared to the others that presented adventitious roots of up to 2 cm in length. The samples were immediately processed for biochemical analysis. The samples were taken from the apical area of the roots because of their importance in the metabolism of the root and because of their sensitivity to anoxia (Chen & Qualls, 2003).
Extraction and Testing of Alcohol Dehydrogenase (ADH)
The extraction and testing of the ADH (Alcohol: NAD oxidoreductase, E.C.1.1.1.1) enzyme activity was conducted as described by Fukao et al. (2006). The crude protein was extracted from approximately 100 mg of fresh tissue of each sample with a mortar pre-cooled over ice and 0.4 ml of cold extraction buffer that contained 100 mM Tris-HCl (pH 9.0), 20 mM MgCl2 and 0.1% (v/v) mercaptoethanol. The extracts were centrifugated for 15 min at 13,500 rpm and subsequently, the supernatant fraction was analyzed for the enzyme activity of ADH. The reaction mixture (1.2 ml) included 60 Ml of extract, 50 mM Tris-HCl (pH 9.0) and 0.10 mM NAD. Ethanol (60 μL) was added to start the reaction, and the activity of ADH was determined by spectrophotometry at 340 nm by the reduction of NAD+ at room temperature for two minutes. Additionally, the protein concentration in the crude extracts was determined by the Bradford protein assay (1976) using BSA for a standard curve.
Study Design and Statistical Analysis
The experiment was exploratory with the aim to evaluate the differences of the activity of the ADH enzyme in the roots of three yellow passion fruit seedlings in conditions of flooding compared to three seedlings in normal conditions (without flooding). The experiment was conducted three times and a descriptive analysis was carried out to observe the behavior of the data on the seedling's enzyme activity in the treatment with and without flooding and across the time of duration of the experiment (unit of time: Day 0, 1, 3, 7, 9 and 14). Taking into account the measures of the response variables (enzyme activity and the specific enzyme activity of ADH) in different units of time in each experimental unit (seedling) for both treatments, the analysis was proposed as a repeated measures design to be able to control the effect of unequal times on the development of the response in each seedling for each treatment. For the purposes of the statistical analysis, the variations of the experiment's repetitions were not taken into account, because they did not present significant differences.
The data were transformed through natural logarithm (NL) to adjust them to a mixed linear model of random coefficients with residuals that have a normal, multivaried distribution, and there is homogeneity in the variance for both response variables (Figure 2). From the fixed effects of the repeated measures, the linear model was estimated for both response variables over time. A contrast analysis was also carried out between the repeated measurements to determine the significance of the differences found between the treatments, the units of time and the interaction for the data of enzyme activity and the specific enzyme activity of ADH. Finally, the differences between both treatments on each day were established by comparing the main effects. This allowed the significance of the time/ treatment interaction to be specified (Arnau & Bono, 2008). For these statistical analyses, PROC MIXED of SAS 9.3 was used with the license of the School of Statistics of the Universidad del Valle.
Results
The enzyme activity of ADH in roots of yellow passion fruit seedlings rapidly increased after the first day of flooding until the ninth day. On Day 14, the response of the activity of ADH decreased a little. However, it continued to be greater than the stability of the activity in the seedlings under normal conditions without flooding (Figure 3).
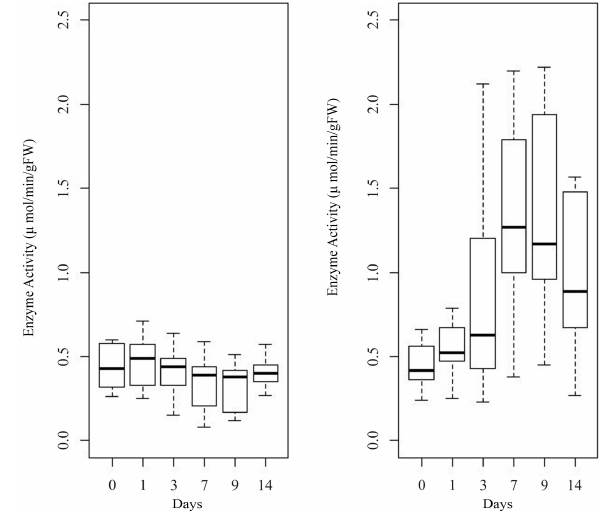
Figure 3 Diagram of cases of ADH enzyme activity in the different units of time. Treatment without flooding (left). Treatment with flooding (right). gFW= gram of fresh weight.
After nine days of flooding, the enzyme activity of ADH showed the maximum increase, three times greater than in zero time of flooding, as well as a high variation in data. In contrast, in the seedlings without flooding, the enzyme activity remained stable and with little variation of data over time (Table 1).
Table 1 Means and standard deviation of the ADH enzyme activity of roots from yellow passion fruit seedlings in each treatment (without flooding compared to with flooding) by unit of time (Day 0, 1, 3, 7, 9 and 14). Mean ± standard deviation (SD).
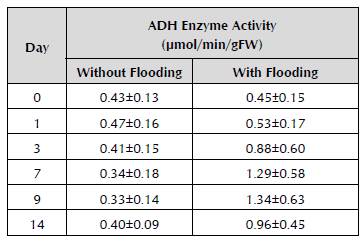
The statistical analysis of the data described in Figure 3 showed that the differences found in the response of the ADH enzyme to the treatments with flooding and without flooding are significant (p < 0.1), like the differences between the units of time and the treatment/ time interaction of the enzyme activity (Table 2).
Table 2 Comparison of the fixed effects and their interaction for the variables of enzyme activity and specific enzyme activity of ADH in the roots of yellow passion fruit seedlings.
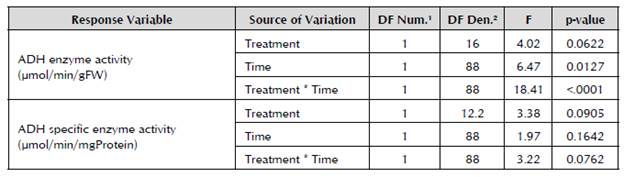
1 Degrees of freedom of the numerator. 2Degrees of freedom of the denominator.
After comparing the values of the enzyme activity between the treatments in each unit of time, it was demonstrated that the significant differences (p < 0.1) occur from Day 3 until Day 14 when the experiment is completed (Table 3).
Table 3 Differences between the treatments with flooding and without flooding in each unit of time for the response variables of enzyme activity and the specific enzyme activity of ADH in the roots of passion fruit seedlings.
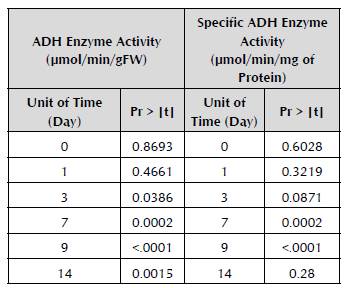
Taking into account the response of the enzyme activity in each one of the seedlings in the experiment, a varied response was discovered over time in each one of the individual seedlings. The great increase in the enzyme activity in the roots of the flooded seedlings compared to the ones not flooded is notable. Additionally, the little variation in enzyme activity in Seedlings 4 and 7, which also presented differences in the formation of adventitious roots, presented a lower speed of emergence (less than 0.05 cm/day) than the rest of the seedlings (approximately 0.3 cm/day) in conditions of flooding. The tendency of the enzyme activity in Seedling 7 is similar to the trend of the plants in normal conditions (without flooding). Seedling 4 tends to increase its enzyme activity but in smaller proportion from the rest of the plants in conditions of flooding.
The model finally found for the activity of the alcohol dehydrogenase enzyme over time in each treatment (Figure 5) shows a growing pattern in the response of enzyme activity in the yellow passion fruit seedlings to the effect of the flooding treatment and the effect of the time of exposure to flooding compared to the treatment without flooding, which does not show a tendency to increase, but instead to remain stable over time.
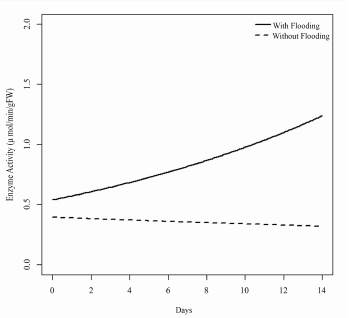
Figure 5 Estimation of the model for the response variable of the enzyme activity of alcohol dehydrogenase in the treatments with flooding and without flooding over time.
Meanwhile, the specific enzyme activity of ADH shows a tendency to be greater in the seedlings subjected to flooding compared to those in normal conditions, but a marked effect is not seen over time (Figure 6).
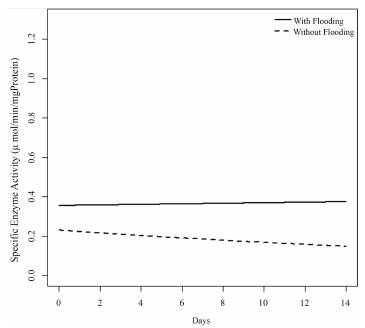
Figure 6 Estimation of the model for the response variable of the specific enzyme activity of alcohol dehydrogenase in the treatments with flooding and without flooding over time.
Despite the contrast between the measurements showing these differences in the treatment but not in time for the specific activity, it was found that there is an effect of the interaction between the treatment and the unit of time (Table 2). Analysis of the differences of the treatment/time interaction is observed in Table 3, which demonstrates that there are significant differences between the treatments with flooding and without flooding from Day 3 until Day 9 of the experiment.
Discussion
The low availability of oxygen is a very important component in the metabolic response of plants to stress from waterlogging. Studies regarding the effect of flooding on the response of the activity of anaerobic metabolism enzymes such as ADH suggest that this response is a tolerance mechanism to the stress caused by the oxygen deficit (Agarwal et al. 2007). The tendency to increase discovered in the enzyme activity of ADH in the roots of yellow passion fruit seedlings in conditions of waterlogging compared to the activity in normal conditions provides evidence that alcoholic fermentation is activated in these plants as a mechanism for the production of energy in conditions of stress caused by the absence of oxygen. The reason why alcoholic fermentation is very important during flooding is the continuous regeneration of NADH that occurs in the cytoplasm during this process (Kimmerer, 1987). Therefore, the process of glycolysis continues and energy is generated in the form of ATP for the maintenance of the roots' metabolism for the plants' survival, because in the absence of oxygen (anoxic conditions), Krebs cycle and the oxidative phosphorylation dependent on the availability of oxygen are blocked (Hossain & Nasir, 2011).
Despite greater enzyme activity of ADH being shown in the roots of the passion fruit seedlings in conditions of waterlogging compared to normal watering conditions, it was found that over time, the enzyme activity only increases after the first day until the ninth day of flooding, and then it decreases. The limited enzyme activity during the first day of flooding was possibly due to the availability of oxygen still present during this period of time. This is because, taking into account the percentages of oxygen saturation of the flooding water (Figure 1), it was found that according to the water quality index introduced by Dunnette in 1979, the percentage of oxygen saturation for Day 0 indicates a good environment (75-89%), and for Day 1, it indicates limited availability of oxygen (60-74%), showing that it is less than at Day 0, but that it is still available for the plant. From Day 3, the environment starts to present less oxygen saturation (< 60%) until the end of the experiment at Day 14, which corresponds to the rapid induction of enzyme activity from this day (3) until the Day 9. Different possible causes were found for the lower enzyme activity at Day 14 than at Day 9. The fact that the samples of this day have been taken from adventitious roots and not from underground roots has an influence, because the adventitious roots are located close to the water surface, where there is greater availability of dissolved oxygen, taking into account that the amount of oxygen dissolved in the water is inversely proportional to the depth (Cifuentes & Torres, 2003). Additionally, there is the possibility that these adventitious roots generated by the seedlings present air-filled cavities (aerenchyma), which according to the study by Escobar (2013), are generated in the adventitious roots of yellow passion fruit in conditions of waterlogging, thus allowing plants to use the oxygen available in the water surface, as well as the oxygen stored in the air-filled cavities, to activate their normal metabolism and reduce their dependency on obtaining oxygen through fermentation. Another one of the possible reasons for which the enzyme activity was less at Day 14 is the time of exposure to the conditions of waterlogging. Studies on Arabidopsis have shown that the activity of ADH is necessary for resistance to waterlogging in the short term (Ellis et al. 1999). This means that its activation is only important during the first days of flooding. Comparing these studies with the response of the enzyme activity of ADH in passion fruit, it can be demonstrated that this rescue mechanism through the activation of the anaerobic metabolism is very important for the plant's survival in the first nine days of flooding. Possibly, the induction of ADH for periods of flooding of more than nine days is not the only cause of survival of the passion fruit seedlings during waterlogging.
The response of the activity of the ADH enzyme presented a great variation between the individual seedlings in the flooding treatment, discovering seedlings that significantly increased their enzyme activity, as well as seedlings such as Seedlings 4 and 7 that presented the characteristic of expressing little activation of the enzyme compared to the rest of the flooded plants, and did not generate adventitious roots at the same speed as the rest of the seedlings in the experiment subjected to the flooding treatment. These variations found in the population could suggest a genetic variability that is generating this behavior of the ADH enzyme activity in the different plants. For example, the characteristic observed in Seedlings 4 and 7 could be related to the variation of an induction factor that affects both attributes in the plants (the enzyme activity of ADH and the formation of adventitious roots). Here, the expression of one gene could have an influence and finally regulate the response to flooding in different characteristics including ethanol fermentation (Fukao et al. 2006). This is a theoretical approximation of what may be occurring in the molecules to result in the variations observed in the yellow passion fruit seedlings in flooding conditions.
This study provides evidence of a possible survival mechanism in yellow passion fruit seedlings in conditions of hypoxia or anoxia in the short term as a result of flooding. This consists of activating the ADH enzyme implicated in anaerobic metabolism, where the waterlogged seedlings present an enzyme activity of ADH up to three times greater than in the seedlings in normal watering conditions. The exploration of other features is proposed, such as the presence of barriers to prevent the radial oxygen loss and regulation and signposting mechanisms in the molecules responsible for triggering responses to tolerate flooding. This could be important for knowledge of the behavior of the passion fruit crop in conditions of waterlogging.