INTRODUCTION
Undoubtedly, many of us who have worked with the bacterium B. thuringiensis are aware of the most recent advances in studying this bacterium, mainly as a controller of insect pests. However, the history of study and evolution in its knowledge has given rise to what is today B. thuringiensis. The history of this exciting bacterium goes back a little over 120 years. Studies on this bacterium, capable of initially controlling some insect pests, have suffered a series of successes and errors that have allowed the scientific world to have one of the most important biological resources for the friendly control of a wide variety of insect pests. The objective of this review is to make it known that beyond essential personalities on the subject, many other people contributed to the in-depth knowledge of this bacterium, at times with failures and others with successful results, contributing erroneous ideas that were taken for granted for decades and that were later corrected based on more modern studies to find out what we know today.
The beginning of Bacillus thuringiensis
Bacillus thuringiensis dates back to the early 20th century when Shigetane Ishiwata in 1901 (Ishiwata, 1901) studied a new disease in the silkworm Bombyx mori (Linnaeus) He discovered that a bacterium was the causative agent of the disease and named it Sotto-Bacillen (Sotto means a sudden collapse in Japanese) (Ishiwata, 1905). The Japanese researchers Aoki and Chigasaki worked with this bacterium during 1911. They described the disease, noting that the bacterium alone could not cause the disease but that sporulated cultures could paralyze the larvae in a short time (Heimpel & Angus, 1960). At the same time, the German scientist Ernst Berliner isolated a spore-forming bacterium that caused disease in the Mediterranean meal moth Ephestia kuehniella (Zeller). The diseased larvae were found in a shipment that Berliner received in the summer of 1909 from a flour mill in Thuringia, Germany (Steinhause, 1951). The disease of these larvae spread to other individuals of E. kuehniella, allowing Berliner to study the disease for several years, and in 1915 he named the causal agent Bacillus thuringiensis (Berliner, 1915). Berliner lost the originally isolated bacillus, but another German scientist Otto Mattes succeeded in re-isolating the bacillus from E. kuehniella in 1927. Edward Steinhaus in California received this isolate from Nathan R. Smith in 1942, who received it from J. R. Porter in 1940. Porter obtained it directly from Otto Mattes in 1936 (Steinhause, 1951).
Almost half a century later, in China studies on B. thuringiensis started when Prof. Ji Cao (1955) obtained a B. thuringiensis strain from a French commercial product, although it is not mentioned I supposed it was Sporeine, and prepared a bacterial powder to test against the European corn borer Ostrinia nubilalis (Hübner). In 1959, Prof. Chongle Liu introduced B. thuringiensis var. thuringiensis from Czech Republic (formerly Czechoslovakia) and Prof. Lvhong Zhang obtained three subspecies (thuringiensis, galleriae and dendrolimus) from the former Soviet Union. In 1961, Prof. Zhongyun Peng imported the Chongjun No. 3 a B. thuringiensis powdered pesticide from Soviet Union and isolated the strain. These four scientists tested the isolates against lepidopterans independently (Li et al., 2017). There are no reports from other countries that have had a strong activity in the investigation of B. thuringiensis. It seems that only the United States, Russia and China were leading the way when it comes to controlling insect pests. Most of the countries where these products began to be marketed imported the strains or the commercial products to control some of their pests.
Studies on mode of action
The B. thuringiensis mode of action was unknown in the past time, but some researchers took on the task of studying the process. The first description of the disease caused byB. thuringiensiswas made by Otto Mattes. He described the disease as consisting of 10 successive stages: 1) ingestion of the spores and germination in the intestine, 2 ) production of vegetative cells in the intestine, 3) damage to the intestinal epithelium by the enzymatic activity of the bacillus, 4) migration of bacteria between host cells in the body cavity, 5) abundant growth of cells in hemolymph, 6) disintegration of the internal tissues, 7) appearance of the external symptoms of the disease, 8) penetration of the bacteria into the tissues of the nervous system, causing the death of the larva, 9) disintegration of the tissues and mummification of the larva, and 10) formation of spores by bacteria (Mattes, 1927). Although the information was not entirely accurate, various researchers took it for granted and began investigating the toxic activity of B. thuringiensis on other insects. Everyone working with this bacterium thought or attributed the pathogenicity to the spores produced by the bacillus. However, in 1953 Hannay was the first to speculate on the possible association of the crystalline inclusions of B. thuringiensis to its toxic action. At first, he described what appeared to be diamond-shaped crystals during the spore formation process in B. thuringiensis. This microorganism was practically indistinguishable from B. cereus at that time, so this finding was relevant. Hannay defined that the crystals were insoluble in water and other polar solvents but were soluble in dilute alkaline conditions. When exposed to these conditions, the crystals increased in size, lost their refractability, and finally disappeared, leaving what appeared to be a membrane; despite these findings, Hannay argued that he was completely unaware of the composition (Hannay, 1953). However, two years later, Hannay and Fitz-James (1955) managed to separate, purify and analyze the crystalline inclusions concluding that it was a tetragonal protein composed of at least 17 amino acids. Later, Thomas A. Angus from Canada thought that the Bacillus sotto Ishiwata was different from the Bacillus thuringiensis Berliner since they could be distinguished by some morphological characteristics and differences in pathogenicity, being more pathogenic Bacillus sotto. However, through his various studies, Angus, as a doctoral student, published as part of his thesis the association of the toxic bacterial capacity with the crystalline inclusions. He also analyzed the protein toxin indicating that it was a stable toxin that caused paralysis and death in the larvae of B. mori and other lepidopteran insects (Angus, 1956a, 1956b, 1956c). At that time, the crystal activity was beginning to be understood; then, the question was how the crystal acted inside the insect. In 1959 Heimpel and Angus began to inquire about the possible site of action of the crystals in susceptible lepidopterans. They classified the susceptibility into three types. Insects belonging to Type I suffered a general paralysis in 1 to 7 h, an increase in blood pH was observed, and finally, they died. In these insects, the leakage from the gut began first in the second and third abdominal segments. Type II insects neither suffered general paralysis nor increased blood pH; however, larvae died in 2 to 4 days. Insects belonging to Type III died in 2 to 4 days with no symptoms of general paralysis; they argued that ingested toxins did not kill the Type III insects without spores. They attributed the spore germination to the crystal presence and its growth in the midgut. The only insect of this type was E. kuehniella; they suggested that “…the crystals are in some way necessary for the successful germination of the spore, and growth of the vegetative cell. The data do not justify extensive discussion, but it is obvious that the toxin alone is relatively harmless to E. kuehniella, as are spores, but this in combination can cause disease leading to death…” (Heimpel & Angus, 1959). Nothing could be further from the truth, however this classification prevailed for many years since Burges et al., (1976) argued that it was necessary a 1:1 combination of crystals and spores to kill Galleria mellonella larvae, even more they concluded that this insect could be classified within the type 3 in Heimpel and Angus (1959) classification.
McConnell, a doctoral student, and Richards, his advisor, in 1959, demonstrated that a toxic substance that killed Galleria mellonella larvae was a toxic thermostable factor and they argued that this factor was only produced when bacteria were living and growing. According to their experiments, which are incompletely described in the paper, and there is no mention of how solubility and dialysis were determined, they concluded that the compound was soluble in water, dialyzable, and it was neither protein nor lipid. They baselessly argued that the inclusion bodies discovered by Hannay in 1953 in B. thuringiensis, and the lecithinase studied by Heimpel in 1955 in B. cereus (Heimpel & Angus, 1960) were heat-labile substances and both were different to the substance they studied. Since this substance was thermostable, and active by injection, they assumed they have discovered a third toxic substance. Although they never defined the kind of thermostable toxin they found, Burgerjon and de Barjac (1960) thought that McConnell and Richards (1959) were dealing with the same toxin that they were working with, although they concluded that the toxin only acted by ingestion, what if it was in the agreement was the characterization of the toxin thermostability (120°C/15 min). The toxin activity was neither so fast and potent as McConnell and Richards argued nor it was totally dialyzable according to Burgerjon and de Barjac (1960). By the method of free ingestion, these French scientists were able to detect that larval mortality was caused by leaves treated with the spore-crystal complex and with the sterile filtrate of the same culture containing the toxin. A complete cessation of feeding was observed and they concluded that mortality caused by the filtrate containing the thermostable toxin was equal or greater than that observed with the spore-crystal complex (Burgerjon & de Barjac, 1960). In the same year Drilhon and Vago (1960) established some of the effects caused to the larvae after ingesting the spores, indicating four categories of the disease progression: type 1) control without infection, no signs of paralysis, type 2) appearance of first sights of cramps [3 h after infection], type 3) partial paralysis [3-5 h after infection], type 4) total paralysis [5-5.8 h after infection].
In 1970 Afify et al., described a series of histopathological damages in the mid-gut, the Malpighian tubes, salivary glands, fat body, nervous system, and skeletal muscles in E. kuehniella after the application of Biotrol BTB, Process 183, which was an experimental formulation produced by Nutrilite Products, Inc. However, they don’t mention how these damages were caused (Afify et al., 1970). Subsequently, Burgerjon (1972) studied some effects in the Colorado potato beetle Leptinotarsa decemlineata (Say) fed with the thermostable toxin sprayed on potato slices. The effects were stopped feeding in the middle of the intermolt and gradually died and some surviving individuals showed teratological features at the nymphal and adult stages.
Some researchers were trying to explain how the B. thuringiensis toxin acted in the intestine of susceptible insects, and Sacchi et al., (1986) had the idea that the first step in the interaction of the toxin with the peritrophic membrane of the midgut that gave it access to the epithelial cells should be a specific receptor. Based on a work by Wolfersberger presented in a meeting in 1985, but published until 1987 (Wolfersberger et al., 1987), dealing with the partial biochemical characterization of brush border membrane vesicles (BBMV) in the cabagge butterfly Pieris brassicae (Linnaeus), additional evidence for the presence of active K-dependent amino acid cotransport was obtained. Sacchi et al., (1986) demonstrated that small amounts of the B. thuringiensis toxin strongly increased the permeability of membrane K+, but not Na+ or H+. They, therefore, indicated that the luminal membrane of the larval midgut cells was the site of action of crystals of B. thuringiensis. Then Hofmann and Lüthy (1986) suggested that binding process of the delta-endotoxin to the target cells was an essential step for their interaction, they also indicated that binding was not as specific as they expected and not always coincide with activity, they were the first to say that “The microvilli are practically the only part of the gut epithelium exposed to the toxin in vivo. In fact, they represent the section of the cell where the action of the toxin can first be found”. By this time the Heimpel and Angus classification was still valid, according to this, Aronson et al., (1986) indicated that the majority of insects fell into type 1, as toxicity appeared to be due to the delta-endotoxin only. Then, Aronson and Arvidson (1987) studied the enzymatic digestion of a B. thuringiensis protoxin, simulating conditions of the midgut larvae. They followed the research of Cooksey (1968) who said “It is possible that there is a second distinct toxin present in the crystal and this too can be detected by its antigenic reaction”. Aronson and Arvidson found that trypsin was able to release and activate a toxin fragment which was toxic to lepidopterans. Knowles and Ellar (1987) proposed a two-step model for the action of delta-endotoxins in the plasma membrane after binding to the specific membrane receptor. The toxin generates small pores in the plasma membrane either by inserting into the membrane or perturbing resident plasma membrane molecules. The formation of this pore lead to a colloid-osmotic lysis causing an inflow of ions and influx of water, cell swelling and lysis. Then, one year later concluded that the membrane proteins, probably glycosylated, participate in the binding process. And the same year Hofmann et al., (1988) found that delta-endotoxin binds with high affinity to BBMV from the midgut causing toxicity, if this union did not occur then there was no toxic activity. Aronson et al., (1991) considered that toxicity differed among crystalline inclusions due to the protoxin composition and consequently the solubilizing conditions within the larval guts. As mentioned elsewhere, it is well known that spores may contribute to insect mortality, but the parasporal crystal is the cause of toxicity and they can exhibit different forms and sizes (Figure 1).
Bacillus thuringiensis classification
According to Edouard Kurstak, many B. thuringiensis isolates were found, but only 20 groups (varieties, strains, serovars) were recognized as different microorganisms. Kurstak isolated in 1962 a strain he called B. thuringiensis var. kurstaki from E. kuehniella larvae. Dulmage later isolated this same strain from pink bollworms and designated it HD-1 (Kurstak & Tijssen, 1982). Thanks to the comparison of antigenic properties of the flagella proteins, the electrophoretic patterns of esterases, and biochemical properties, B. thuringiensis serotypes and varieties could be distinguished (Kurstak & Tijssen, 1982). At first de Barjac and Bonnefoi (1962) subdivided the crystalliferous bacteria into six “biochemical groups” supported by the presence of an H antigen which is group specific. They found that two serotypes could be divided into “biotypes”, meaning they have the same H antigen, but some biochemical differences. Then Bonnefoi and Barjac (1963) proposed to form a group with the crystalliferous bacteria and classify the strains by serotypes. They also found that H4 antigen could be divided in subfactors. Four years later, Heimpel (1967) published a new key for B. thuringiensis classification, but interestingly he only retained two species: B. thuringiensis and B. finitimus. One year later, after a careful review de Barjac and Bonnefoi (1968) proposed a classification based on nine serotypes. The evolution of the serotypes is shown in table 1.
Table 1 Changes in B. thuringiensis serotype classification.
By 1977, 20 serovars with 16 serotypes were already known (Dubois & Lewis, 1981). Although this classification did not consider the pathogenic activity, many B. thuringiensis isolates were extensively studied to control a wide variety of insects, in some cases with successful results and others not so much. However, the discovery of many strains of B. thuringiensis producing different types of crystals and the variety in their activity made Crickmore et al., (1998) review the cry and cyt gene nomenclature based on protein sequence similarity. This new nomenclature overthrew the first nomenclature proposed by Höfte and Whiteley (1989) that classified these genes by their insecticidal activity.
Bacillus thuringiensis field trials
Mattes’ isolate was finally distributed to many laboratories worldwide, and the early attempts to control insect pests were conducted using this isolate in commercial products (Norris, 1970). The first trials with B. thuringiensis were conducted with the European corn borer, Ostrinia nubilalis (Hübner) because it was causing significant damage and economic losses in the United States. The International Group for Corn Borer Investigation suggested applying B. thuringiensis to control O. nubilalis. Under this program, field trials were conducted in Hungary in the late 1920s and Yugoslavia (known as Serbia and Montenegro until 2006, when they became independent states) in the early 1930s. Results from these trials were inconclusive but promising (Jacobs, 1950).
Some years later, Steinhause came up with the idea of causing a bacterial disease in the alfalfa caterpillar, an insect of primary importance in California, due to its short incubation period instead of using a slow-to-kill polyhedrosis virus. He remembered that a B. thuringiensis isolate had been kept in his refrigerator since nine years ago, and he decided to test it in experiments against the alfalfa caterpillar. Steinhause obtained successful results at the laboratory to control the alfalfa caterpillar Colias eurytheme Boisduval. These results encouraged him to conduct preliminary tests in an alfalfa field in California by applying a concentrated spore powder in a suspension diluted with water and spraying it. The spore powder was prepared in Povitsky bottles containing nutrient agar. After three days, the spores were collected with water, washed by centrifugation, and filtered through a cheesecloth; the contents were poured into an enameled pan and allowed to dry. Then the powder was scrapped from the pan and ground with a mortar to obtain a fine powder. The powder was sprayed uniformly over the alfalfa at a total concentration of 109 spores/ml. Results attributed to spores activity showed progress in the infection, and a large number of bacilli were found in the insect fluids and tissues. His work was published in Hilgardia (Figure 2), in my opinion I consider that this detonated the real start of B. thuringiensis as a control agent for pest insects. In his study, Steinhaus shows a photograph of B. thuringiensis spores from a three-day-old culture on nutrient agar. The spores and bipyramidal crystals can be clearly seen in the said photograph. However, he considered crystals only as remains of the vegetative cells (Figure 2B) (Steinhause, 1951).
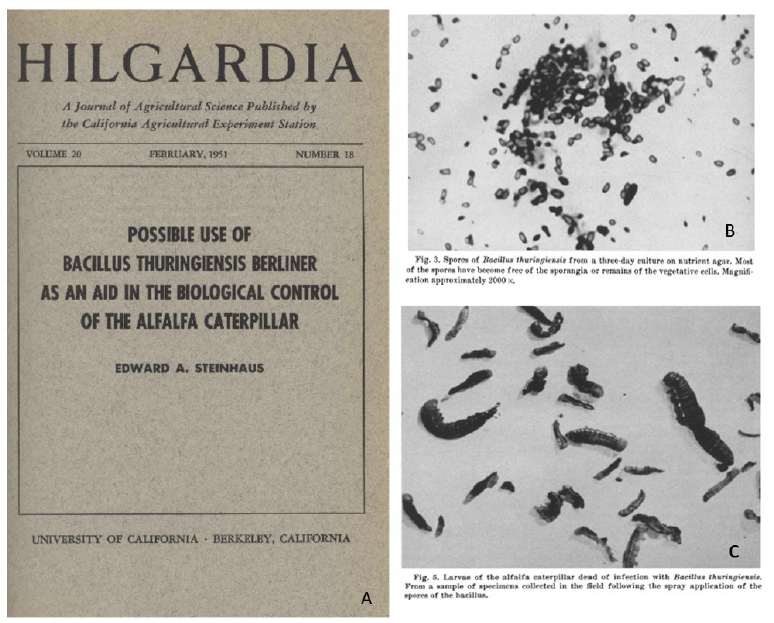
Figure 2 Pictures taken from the Hilgardia. A) journal cover. B) B. thuringiensis 3 day-old culture. C) alfalfa carerpillar infected with B. thuringiensis, (Steinhause, 1951).
Burgerjon and Grison (1959), in Laboratory La Minière in France, evaluated susceptibility to B. thuringiensis of insects belonging to ten families Pieridae, Arctiidae, Noctuidae, Lymnatridae, Notodontidae, Gemotridae, Lasiocampidae, Pyralidae, Tortricidae, and Tineidae finding out eight families susceptible, eight families moderately sensitive and eight families practically insensitive. They mentioned that their findings had only indicative and provisional values; they just wanted to know the possibilities of using microbiological control in insects. These works may be considered the first attempts in formulation development.
First commercial products based on Bacillus thuringiensis
Laboratoire L.I.B.E.C. in France started the commercial production of Sporeine, which became available in 1938. World War II stopped the production of Sporeine; however, Jacobs (1950) reported its effectiveness. The product was described as a powder containing 10% by weight of pathogenic bacterial spores formulated in bentonite, effective against E. kuehniella. The spore powder was mixed with flour, and a concentration of 0.3% was sufficient to prevent complete larval development. Although dusting with the spore powder gave satisfactory results, Jacobs considered assessing different application methods and amounts of this insecticide. Something interesting is that Jacobs did not mention the strain used for Sporeine production; however, Yamvrias (1962) mentioned that Sporeine was made of spores of B. thuringiensis var. thuringiensis.
From 1950 to 1970, more B. thuringiensis isolates were obtained, totaling 150 different isolates (Norris, 1970). A critical study was conducted by Hall and Dunn (1958); they tested B. thuringiensis Berliner on several insect pests. They used a highly concentrated spore suspension, 0.25 g, 1 g, 2 g, or 4 g/100 ml water; however, the results were variable on the insects tested.
The United States commercialization of bioinsecticides began on 27 September 1956 when Robert A. Fisher, Director of Research and Development of Pacific Yeast Products, Inc., advised by Steinhause, became this enterprise an insecticide manufacturer (Steinhaus, 1975). By 1958 Thuricide was the first commercial B. thuringiensis product in the U.S.A. produced by Pacific Yeast Products (then Bioferm Corp., later sold to International Minerals and Chemical Corp.) of Wasco, California, available for testing. The Pesticide Regulation Division of the USDA registered the trade name of Thuricide in 1961 (Reardon et al., 1994).
Maurie Semel from Long Island Vegetable Research Farm in Riverhead, New York, conducted field tests in cabbage against the cabbage looper Trichoplusia ni (Hübner). He tested a polyhedrosis virus with wettable powders made of B. thuringiensis from Nutrilites Products, Inc., and Rohm and Hass Co., arguing the virus was specific, but B. thuringiensis was able to attack several pests. Results indicated that the virus succeeded, but the B. thuringiensis wettable powders were ineffective or not easily self-dispersed to control the pest (Semel, 1961).
The Hoechst 2802 Biospor was the first industrial B. thuringiensis preparation in West Germany. This insecticide was officially recognized in 1964 by the Federal Biological Institute for Agriculture and Forestry. Farbwerke Hoechst AG Frankfurt-Höchst developed the product, and was produced as a spray powder (Herfs, 1965).
The first commercial B. thuringiensis product in China, Qingchong, was produced in late 1965 under the trade name San Wu Brand. Qingchong together, 424 Bt Preparation were launched on the Chinese market (Li et al., 2017).
Commercial production of B. thuringiensis products started in the Czech Republic (formerly Czechoslovakia) in 1958 with the trade name of Bathurin, produced by Chemapol-Biokyma, and in 1959 in what was formerly known as USSR, Entobacterine (based on var. galleriae) was produced by Glavmikrobioprom. With time USSR produced Dendrobacilline (var. dendrolimus), Toxobacterin and Insectine (both based on var. insectus), B.I.P. (var. caucasicus), Bitoxibacilline (var. thuringiensis), and Gomeline (var. galleriae). Turingin and Tumitox were produced in Romania, although the variety with which they were produced is not known; Dipel was also produced in the Republic of Bulgaria (var. kurstaki) under Abbot Licence, and Baktukal (probably var. thuringiensis) in the former Yugoslavia by Serum Zawod Kalinovica (Lüthy et al., 1982; Weiser, 1987).
Meanwhile, in the Netherlands, Van der Laan and Wassink, in 1962, reported severe trouble with elm trees in Amsterdam caused by the tent caterpillar Malacosoma Neustria (Linneaus). To control this pest, they used to apply Derris powder, a rotenone-based insecticide (Kalshoven, 1959), but they were seriously considering replacing such insecticide with some environmentally-friendly alternative. For this purpose, Bacillus thuringiensis Berliner insecticides, such as Bactospeine, Thuricide, and Biospor, were chosen for application on second to fourth instar larvae. Mortality caused by B. thuringiensis products was higher than that of Derris dust (Laan & Wassink, 1962). Interestingly, the three commercial insecticides were diluted with talc to 10% and applied as dust.
Borgatti and Guyer (1962) tested feeding sensitivity in quails to B. thuringiensis commercial formulations in the United States. Surprisingly, quails showed severe toxicity symptoms when fed with Agritrol (MB-3, Str. 0712, 1959) manufactured by Merck & Co and an Experimental Microbial Insecticide (Lot No. 3, 1959) produced by Rohm and Haas Co., because these materials were contaminated with chlorinated hydrocarbon insecticides. They found that Agritrol contained a mixture of DDT and aldrin, and despite various efforts to eliminate toxic substances from these products, the experimental microbial insecticide continued to be toxic to quails. However, these authors concluded that uncontaminated B. thuringiensis products could be ingested by animals safely at very high rates.
Since 1980 the insect pest, the spruce budworm Choristoneura fumiferana (Clemens) in Canada, the gypsy moth Lymantria dispar (L.) in the USA, and the Old World bollworm Helicoverpa armigera (Hübner), and the soybean looper Chrysodeixis includes (Walker) have been controlled with B. thuringiensis biopesticides (Polanczyk et al., 2017).
The commercial formulations used against the gypsy moth containing the Berliner strain were Thuricide and Biotrol, produced from 1961 to 1966. By discovering the HD-1 strain, pesticides such as Thuricide and Dipel were manufactured with this strain between 1971 and 1974 (Dubois & Lewis, 1981). Four companies, such as Abbot Laboratories (Dipel), Ecogen Inc. (Condor OF), Novo Nordisk Bioindustrials Inc. (Foray 48B and Foray 76B), and Sandoz Crop Protection (Thuricide), produced formulations based on HD-1-kurstaki. Additionally, Sandoz produced an aqueous flowable suspension on Thuricide with the strain B. thuringiensis var. kurstaki, named NRD-12, which was more potent than HD-1(Dubois & Lewis, 1981; Reardon et al., 1994). In the 1980s, the prevailing products on the market were produced by various companies from different serotypes, as shown in table 2.
Table 2 Bacillus thuringiensis-based products currently manufactured
Commercial brand | Country | B. thuringiensis subespecies | Company | Year |
---|---|---|---|---|
XenTari | USA | aizawai | Certis | 1991 |
Agree WG | USA | aizawai | Certis | 1992 |
Biobit HP | USA | kurstaki | Valent | 1971 |
DiPel DF | USA | kurstaki | Valent | 1971 |
Javelin WG | USA | kurstaki | Certis | 1971 |
Deliver | USA | kurstaki | Certis | 1971 |
Costar | USA | kurstaki | Certis | 1971 |
Condor WP | USA | kurstaki | Certis | 1994 |
Crymax | USA | kurstaki | Certis | 2002 |
boreGONE! | USA | galleriae | Phyllom BioProducts | 2013 |
Trident | USA | tenebrionis | Certis | 2016 |
Novodor | USA | tenebrionis | Valente | 1988 |
AquaBac | USA | israelensis | Becker Microbial Products | 1985 |
BMP 123 | USA | israelensis | Becker Microbial Products | 1993 |
Lepidocide* | Russia | kurstaki | Sibbiopharm | 1985 |
(Arthurs & Dara, 2019). *http://en.sibbio.ru/catalog/crop/lepidotsid/
Formulations standardization
Interest began to emerge in the standardization of B. thuringiensis preparations because Burgerjon (1959) believed that B. thuringiensis spore preparations should be standardized as any other insecticidal preparation so that they could be applied adequately in the crops. He proposed a Biological Unity, which expressed preparation toxicity, defined as "the ratio between the LD50 of a standard preparation and that of the test sample". The test was simple, and it was based on food consumption by larvae immediately after being fed with the B. thuringiensis preparation. Feed consumption was inversely proportional to the concentration used. This method was suitable for controlling the product crystal content at the commercial level. However, the method was limited and valid only if the manufacturing process remained unchanged, although they suggested it helped titrate crystals from different products. In addition, if different laboratories used the method, they must have the same homologous reference preparation (Burgerjon, 1965).
Some years later, Burges (1967) indicated that adopting the preparation E61 as an international standard provided a recognized material to compare other preparations. The preparation E61 (E for the French word Etalon for "standard," and the number 61 for 1961, the year of its production) was manufactured by the firm Roger Bellon, which produced 42.7 kg of the powder containing spore-crystal complex 21.5%, bentonite FB2 65.3% and Clarcel C 13.2%. Product stability was determined over a nine years period and was distributed by Laboratoire de Lutte Biologique, Pasteur Institute in Paris by Dr. H. de Barjac. At that time, the E61 was used to produce Bactospéine (international units: E. kuehniella) and all the preparations from I.N.R.A.-La Minière (international units: Pieris brassicae) in France. In the USA was used for Thuricide, Dipel, and Biotrol (international units: Heliothis virescens or T. ni). de Barjac and Burgerjon (1972) recommended that each producer develop their own standard, simply by maintaining a homogenous bacterial stock. If the mass production inoculum was based on a strain other than E61, it was preferably not to use E61. Also, it worked as a basis to determine toxicity units, but this material could work only with products containing the same strain as the standard. The standard should be homologous. However, this was not the panacea. The standard could be partially homologous with products from different strains, or the insect susceptibility could be different. Burges suggested the possibility of grouping insect species according to their susceptibilities; however, this would not work as moreB. thuringiensisstrains were discovered or genetically manipulated.
(Dulmage et al., 1970) isolated a B. thuringiensis var. kurstaki strain named HD-1, later used in the United States for commercial production. Then he established a bioassay with T. ni as a test organism. The idea was to determine the product potency through comparative bioassays vs. the standard preparation and express it in international units (IU). The international standard they used was E-61 (Burgerjon & Dulmage, 1977), which was arbitrarily assigned 1000 toxicological units (Pieris brassicae)/mg (Burgerjon, 1965). In 1972 the result of four evaluations conducted by USDA Laboratories in Brownsville, TX., Abbot Laboratories, International Minerals, and Chemical Corp., and Nutrilite Products, Inc.,yielded different and drastic values, so the four companies agreed to assigned potency of 18,000 IU/mg (value obtained by Dulmage) to the primary US reference standard coded as HD-1-S-1971 (HD-1 was for the B. thuringiensis isolate, S for primary standard, and 1971 for the year of preparation) (Dulmage, 1973).
In 1965, the International Organization for Biological Control (IOLB) designated the Bacteriological Control Service as the International Reference Center of the IOLB for Bacillus thuringiensis. After the discovery of the H-14 serotype (Goldberg & Margalit, 1977) and its ineffectivenes against lepidopteran caterpillars, but highly toxic against Diptera particularly mosquitoes and blackflies. This serotype described as B. thuringiensis var. israelensis became of great interest for several groups for large scale production and marketing. For this reason the IOLB in assciation with the World Health Organization (WHO) produced a new standard based on H-14 serotype to be used against Aedes aegypti (Linnaeus). This reference standard was name IPS-78 (International Pasteur Standard 1978) and was distributed worlwide (de Barjac, 1979).
In 1982 in Brownsville, TX, a new standard was developed because the previous one was running out. Abbot Laboratory, Biochem Products, Sandoz, Inc., Upjohn Co., and USDA Brownsville agreed to assign HD-1-S-1980 as the new standard with a potency of 16,000 IU/mg (Beegle et al., 1986).
In 1986, the Institute for the Control of Agrochemicals (MOA) in China, began to produce two B. thuringiensis standard preparations, CS3ab-1986 with a potency of 7,400 IU/mg and CS5ab-1986 with a potency of 8,600 IU/mg. Some time later standards were upgraded and two new preparations emerged, namely, CS3ab-1991 with a potency of 15,000 IU/mg and CS5ab-1991 with a potency of 20,000 IU/mg Then, with the purpose of regulating the B. thuringiensis industrial production, China enacted the standards HG3616-1999 as raw powder, HG3617-1999 as wettable powder, and HG3618 as suspending agent. In 1995, the MOA departmental standard for B. thuringiensis preparations (NY293-5) became effective, thus achieving concordance with international standards. Chinese standards were based on B. thuringiensis subsp. aizawai and B. thuringiensis subsp. kurstaki (Li et al., 2017).
At first, when it was thought that the spores killed the insect, the idea of standardization seemed simple, but after they realized that it was the delta-endotoxin that exerted the insecticidal activity, standardization became complicated. Most B. thuringiensis-based products today are not standardized and contain a legend indicating so.
What do we know so far?
Thanks to all the information acquired over a century, we know that B. thuringiensis is a Gram (+) bacterium, rod-shaped, facultatively anaerobic, chemoorganotrophic, with positive catalase activity, forming spores. The vegetative cell is 1 to 1.2 µm wide and 3 to 5 µm long and contains short peritrichous flagella (Iriarte & Caballero, 2001). One of the particular characteristics of B. thuringiensis, which makes it distinctive from other bacteria, is the production of parasporal inclusions during the sporulation process. This crystal is much more notorious; it is separated from the endospore and is released into the environment when the cell wall degrades (autolysis) at the end of sporulation. This crystal can represent up to 30% of the dry weight of the sporangium (Ibarra, 2005). This bacterium is ubiquitous in the environment and is widely distributed worldwide, mainly due to its sporulating capacity, which gives it high resistance to heat and dryness.
It is currently the natural insecticide par excellence, due to the large number of families of insects and other invertebrates that it can control. However, recently it has been discovered that the capacities of B. thuringiensis go beyond biological control; for example, some strains produce parasporins, which are proteins with antitumor properties (Santos et al., 2022) and offer an excellent alternative in cancer control. Or the discovery of a B. thuringiensis strain with the capacity to solubilize phosphates allows it the potential to develop new agrobiological products (Sauka et al., 2021).
The management of B. thuringiensis has focused on the constant search for Cry proteins with new biological activities, and the production and improvement of the already known Cry proteins, suitable niches, improving the forms of B. thuringiensis application and its persistence in the environment. To date, the "Bacillus thuringiensis Toxin Nomenclature" web page includes 858 different Cry toxins (Crickmore et al., 2018), which gives a wide range of possibilities for using this bacterium.
Something very current is the improvement of biological formulations so that they are effective towards the pest to be controlled and friendly to the environment, for which a wide variety of materials and types of formulations have been used. Among the most innovative are hydrogels, which function as controlled release systems comprising multi-component systems that absorb large amounts of water (Tay et al., 2020) and water-dispersible powders for mosquito control (Shankar et al., 2019).
The combined use of B. thuringiensis is a recent idea that has been used to offer new strategies for pest control. For example, B. thuringiensis has been used to enhance oral infectivity in combination with ascovirus (Yu et al., 2020). Combining Bt with baculovirus has been used to increase the pathogenicity of both agents (Guido-Cira et al., 2017). The use of the entomopathogenic fungus Isaria fumosorosea or Beauveria bassiana with B. thuringiensis has shown synergistic action toward the control of some pests (Nian et al., 2015; Wraight & Ramos, 2017).
Escherichia coli expression systems are currently used to express cry genes, especially for novel gene activities and genetic insecticidal mechanisms. The expression of cry genes can be directed by cry gene or non-cry gene promoters. Several cry genes have been used to produce transgenic plants to ward off insect pests (Peng et al., 2019). It has been used to express heterogeneous proteins such as Cyt1A and P20, making B. thuringiensis a recombinant bacterium with higher toxic specificity (Elleuch et al., 2014). Since the continued application of B. thuringiensis formulations has resulted in insect resistance development, B. thuringiensis varieties expressing two or more different toxins help regulate the resistance mechanism (Ahmad et al., 2021).
What intrigued scientists about using B. thuringiensis was its final fate in the environment. The idea of crystal deterioration caused by light was always accepted; today, it is known with more certainty that UV rays from sunlight quickly degrade the Cry proteins and that its half-life is one week (Hung et al., 2016). Likewise, there have been significant advances in the study of the resistance of B. thuringiensis to UV, which has made it possible to determine that the RecG protein, which is an ATP-dependent DNA helicase, is strongly involved in the resistance to UV (Xu et al., 2020). This problem has led to multiple studies that allow increasing its residuality in the environment. From natural products such as nixtamalized corn (Tamez-Guerra et al., 2000), starch, gelatin, corncob (Rosas -García et al., 2009) to the use of olive mill wastewater (Jallouli et al., 2014) and organic acids (Isayama et al., 2021), have been used with high success.
Bacillus thuringiensis was, is, and will continue to be studied because it still has a lot to contribute to science for the benefit of humankind.
CONCLUSIONS
Throughout history, scientists of different nationalities have contributed to the knowledge of B. thuringiensis, although it is true that many of the findings that were taken for granted at the time were utterly erroneous; however, progress in their study allowed those errors to be corrected for well-founded successful results. Bacillus thuringiensis has become a famous bacterium worldwide mainly for its ability to control pest insects, and although to date we have magnificent strains, we also have others that we have not found valid; hence this bacterium becomes even more interesting because 100 more years of study will not be enough to know it completely.