INTRODUCTION
The Trans-Andean shovelnose catfish, Sorubim cuspicaudus, is a migratory species that inhabits the Magdalena, Cauca, Sinú, and Catatumbo basins. It is categorized as vulnerable due to a gradual decline in its capture volumes in recent years (Mojica et al., 2012). In 2006, the Magdalena river's basin reported a capture volume of 416.5 tons, which drastically decreased to 65.8 tons in 2017, marking a reduction of 84% (INCODER, 2006; De la Hoz Maestre et al., 2017). Over the past decades, the Magdalena basin has experienced substantial environmental changes resulting from mining, oil extraction, agriculture, damming, and other anthropogenic activities. These alterations have led to habitat loss and degradation of water quality, consequently affecting native fish populations (Mojica et al., 2012; Lozano et al., 2017).
Ex situ conservation strategies such as captive reproduction allow the massive production of fingerlings of fish species with some vulnerability (Prieto-Guevara et al., 2015). Atencio et al., (2010), state that three phases can be identified in the production of fingerlings: management of broodstock, induced reproduction, and larviculture. Considerable progress has been made in the initial two phases (Prieto-Guevara et al., 2015). However, the most notable challenges have been observed in larviculture, particularly during the initial feeding phase. During this period, mortality attributed to cannibalism is documented (Atencio et al., 2010) along with elevated stress levels that disrupt the fish's physiological equilibrium (homeostasis). This disruption leads to immunosuppression, increased diseases susceptibility, and decreased survival rates (Aerts et al., 2018). Consequently, it is imperative to explore alternatives or strategies to enhance the performance of larviculture for this catfish. Among the successful options used in other fishes, probiotics stands out. These microorganisms play a pivotal role in maintaining the intestinal microbial balance, providing nutritional benefits, offering protection against pathogens, and contributing to both development and immune responses (Pérez-Sánchez et al., 2018; Paixão et al., 2020).
Probiotics are used in domestic animal production systems and have been introduced in recent years in commercial aquaculture, both in larviculture and in grow-out cultures(Martínez Cruz et al., 2012; Newaj-Fyzul et al., 2014; Pérez-Sánchez et al., 2014; Ribas & Piferrer, 2014; Kumar et al., 2016; Hoseinifar et al., 2016; Cai et al., 2018). The beneficial effect of probiotics on aquaculture production is attributed to their ability to improve water quality, reduce accumulated organic matter, inhibit pathogens through various pathways, enhance the immune system, and provide nutritional benefits by influencing digestibility and feed utilization (Carnevali et al., 2006; Nayak, 2010; Cai et al., 2018). Additionally, probiotics presents functions similar to antibiotics without generating drug resistance (Thurlow et al., 2019).
Nevertheless, it is imperative to consider factors such as the selection of strains capable of giving benefits to the host and maintaining stability at sufficient densities over time (Balcázar et al., 2006; Begley et al., 2006; Interaminense et al., 2018). Similarly, the host species, developmental phase (larvae, fingerlings, grow-out), environmental culture conditions, and administration route must be considered (Soltani et al., 2019; Ringø et al., 2020). Probiotics can be applied orally through food, primarily influencing the intestine, or indirectly by administration into the culture water to enhance its quality (Qi et al., 2009).
The studies reveal that the use of mixtures of probiotic microorganisms is more effective than the independent strains in the control of pathogens, in the improvement of productive performance parameters and in the establishment of microorganism populations, observing synergistic processes (Van Doan et al., 2018; Soltani et al., 2019; Kuebutornye et al., 2020). Among the bacteria with probiotic capacities is the genus Bacillus; one of the most used in aquaculture (Ringø et al., 2020). Bacillus is spore-forming bacteria highly resistant to aggressive physical and chemical conditions, surviving in various environments, including freshwater, marine sediments, sand, thermal waters, arctic soils, and the gastrointestinal tract of fish, crustaceans, and mollusks; providing a wide range of beneficial effects (Soltani et al., 2019).
Bacillus species can play an important role in removing waste products for more sustainable aquaculture, reducing stress in fish, leading to better immunophysiological balance, better growth and higher survival in aquatic animals (Soltani et al., 2019). In addition, Bacillus stimulates the presence of beneficial bacteria and decreases the load of pathogens in ponds. Most species in this genus are producers of various secondary metabolites containing antibiotics, helpful chemicals, and enzymes (Zokaeifar et al., 2012; Cai et al., 2018; Ringø et al., 2020).
The objective of the study was to evaluate the effect of Bacillus subtilis and Bacillus licheniformis as a probiotic at different levels of inclusion in the water on the growth and gastrointestinal development of the catfish Sorubim cuspicaudus larvae.
METHODOLOGY
Biological material and treatments. The research was conducted at San Silvestre S.A. Fishculture Station (Barrancabermeja, Santander, Colombia), situated at geographic coordinates 7°06'31" north latitude and 73°51'25" west longitude, at an elevation of 80 m.a.s.l. and average annual temperature of 28.4°C. Larvae were obtained through the hormonal induction of S. cuspicaudus broodstock originating from the middle basin of the Magdalena River. These broodstock had been adapted to captive conditions for over a year, being fed ad libitum with forage fish and supplemented daily with a diet of 34% crude protein based with a 1% fish biomass equivalent ratio.
The females and the males were induced at 10 µg/Kg of GnRH analog of live weight (Pardo-Carrasco et al., 2015). The embryos were placed in incubators with up-flow water (4L/min), and newly hatching larvae were transferred to circular pools with constant aeration at a density of 80 larvae/L. 2720 larvae of 42 hours post hatching (HPE) were placed in 16 aquariums of 10L. Dissolved oxygen, pH, temperature, electrical conductivity, and dissolved solids were measured daily with a multiparameter probe (YSI, Pro Plus, USA), while hardness and alkalinity by titration (HACH, 1690001, USA), and turbidity with a turbidimeter (LaMate, 2020we, USA) at the beginning, in the middle and at the end of the experiment.
For 22 days, four probiotic inclusion levels were evaluated in the water containing 50% Bacillus subtilis and 50% Bacillus licheniformis: 0 (control), 5, 10, and 20 ppm each with four replicates per treatment. In each aquarium, 170 larvae were stocked, fed twice a day (morning and afternoon), with recently hatched brine shrimp nauplii (instar I) as follows: days 1 to 5, 10 nauplii/ml were offered; from 6 to 14 20 nauplii/ml were offered and from 15 to 22 40 nauplii/ml were offered.
Growth and survival. At the end of the experiment, 15 larvae were taken per experimental unit for growth evaluation. The larvae were weighed on an analytical balance (Chyo, JS-110, Japan) and the total length was measured with an image analyzer (ImageJ, USA). The following parameters were estimated:
Weight gain (Gw) = Wf - Wo; where Wf and Wo correspond to the final and initial weight of the larvae. Length gain (Gl) = Lf-Lo; where Lf and Lo correspond to the final and initial total length of the larvae. Specific growth rate (G) = 100x[Ln(Wf)-(Ln(Wo)]/t; where t is the culture time expressed in days and Ln is the natural logarithm. The final survival (S) is estimated by dividing the final number of larvae by the total number of initial larvae in each experimental unit.
Stress resistance test. 10 larvae were taken per replicate and placed out of the water on absorbent paper for eight minutes, then transferred to a container with water from the same replicate for 15 minutes, and survival was estimated based on the method used by Atencio Garcia et al., (2003).
Microbiological analysis of water. On days 0 and 22 of the experiment, bacterial count was conducted using nutrient agar plates. For this purpose, 0.1 ml of water from the aquariums was extracted, diluted to 1/1000 with a 0.9% saline solution, and then incubated at 30±2°C for 48 hours. Counts ranging from 30 to 300 colony-forming units (CFUs) were considered for enumeration (CFU/ml x103).
Histological analysis. Fifteen larvae per replicate were collected at the initial and final of the experiment. These larvae were anesthetized using eugenol (40 ppm), fixed in 10% buffered formalin, embedded in paraffin, and subjected to histotechnical processing. Subsequently, 5 µm histological sections were generated employing a rotary microtome (Leica, RM2125, Germany) and stained with hematoxylin-eosin. A histological description of the digestive tract was performed, and the length of the intestinal folds in the anterior, middle, and posterior regions was measured (n=3 per region). This measurement was conducted utilizing an image analyzer (Image J, USA) employing the methodology suggested by (Mokhtar et al., 2015).
Statistical analysis. A completely randomized design was employed, and all evaluated parameters were subjected to normality tests (Shapiro-Wilk test) and homoscedasticity (Levene test). Subsequently, they were subjected to ANOVA, followed by a Tukey Multiple Range test. In all instances, a significance level of p<0.05 was adopted to indicate statistical differences among treatments. The analyzed parameters were presented as mean ± standard deviation (SD). Statistical analysis was executed using the SAS program (version 9.1, 2004, USA).
All animals handling procedures followed the standards for using laboratory animals outlined by the Committee on the Care and Use of Laboratory Animal Resources of the National Research Council (National Academies, USA). This research had a permit issued by the Colombian National Aquaculture and Fisheries Authority - AUNAP (Resolution 0955 of May 27, 2020).
RESULTS
Growth performance. The growth, survival, and stress resistance outcomes are presented in Table 1. Larvae treated to a probiotic inclusion level of 20 ppm exhibited higher Gw, Gl, and G, displaying a statistically significant difference from the other treatments (p<0.05). In contrast, the control group showed lower values. Survival rates (between 31.0% to 23.1%) and stress resistance (between 87.5% to 97.5%) displayed no significant statistical difference among the treatments (p<0.05).
Table 1 Growth performance of catfish Sorubim cuspicaudus larvae, treated to different levels of inclusion of probiotics in the water. G, specific growth rate; Gl, length gain; Gw, weight gain. Different letters in the column indicate a significant difference (p<0.05).
Mean values followed by different letters in the same column differ significantly (P<0.05).
Digestive tract and intestinal folds.Figure 1 shows the histological features of the intestine in S. cuspicaudus larvae at the beginning of the experiment. In the control treatment, more pronounced development was evident in the intestine middle and posterior sections than in the anterior section. Among larvae receiving probiotic supplementation (5, 10, and 20 ppm), the most caudal portion of the esophagus exhibited finger-like folds oriented both caudally and, to a lesser extent, cranially. These folds were covered by a simple cylindrical epithelium supported by the basal lamina, and, under this, a thin layer of loose connective tissue known as the lamina propria. Moreover, enhanced fold development was observed in the foregut, demonstrating a nearly similar relationship with the middle and posterior regions, when contrasted with the larvae in the control group.
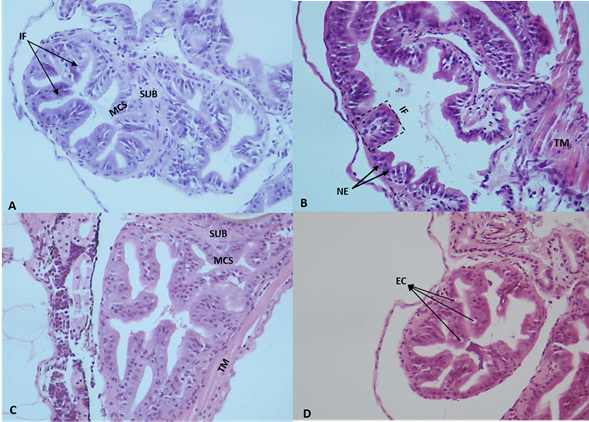
Figure 1 Photomicrographs of intestinal folds of catfish Sorubim cuspicaudus larvae at the beginning of the experiment treated to different inclusions of probiotics in the water. 0 ppm, control (A), 5 ppm (B), 10 ppm (C), 20 ppm (D). EC: epithelial cells; EN: stratified flat epithelium nuclei; IF: intestinal folds; L: lumen; MT: muscular tunic; TA: mucosal tunic; PL: propia layer; SCE: simple cylindrical epithelium; ST: serosa tunic (H&E, 40X).
At the end of the experiment, less development of the esophagus, stomach and intestine was observed in the control treatment concerning the treatments including probiotics, especially at 20 ppm (figure 2). In addition, differences in size, length, width, and development of intestinal folds of the larvae treated with the probiotic and the control group were observed. Also, in the treatments with the inclusion of probiotics, it was observed that in the middle portion of the intestine, there was a greater presence of goblet cells in the epithelium and, in the posterior portion, the presence of multiple epithelial cells with small intracytoplasmic vacuoles.
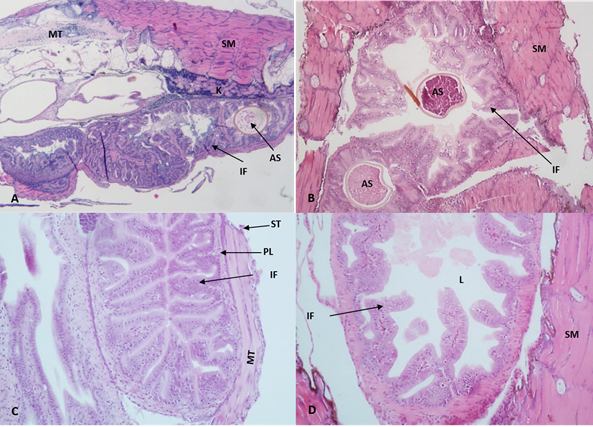
Figure 2 Photomicrographs of intestinal folds of catfish Sorubim cuspicaudus larvae at the end of the experiment treated to different probiotic inclusions in the water. 0 ppm, control (A), 5 ppm (B), 10 ppm (C), 20 ppm (D). AS: artemia cyst; EC: epithelial cells; IF: intestinal folds; K: kidney; L: lumen; PL: propia layer; MT: medular tissue; NE: nuclei of the stratified squamous epithelium; SM: skeletal muscle; ST: serous tunic; MT: muscular tunic (H&E, 20X).
Figure 3 shows the measurement of the intestinal folds at the experiment's beginning and end. Initially, no significant difference was observed between treatments (p<0.05), which allows us to infer that the catfishes presented the same initial conditions. At the end of the experiment, a significantly greater length of intestinal folds (p<0.05) was found in larvae with the inclusion of 20 ppm of probiotics (205.3±72.7 µm), compared to the control (119.7±31.4 µm); but without statistical difference with the larvae maintained at 5 ppm (162.2±34.5 µm) and 10 ppm (179.0±39.9 µm).
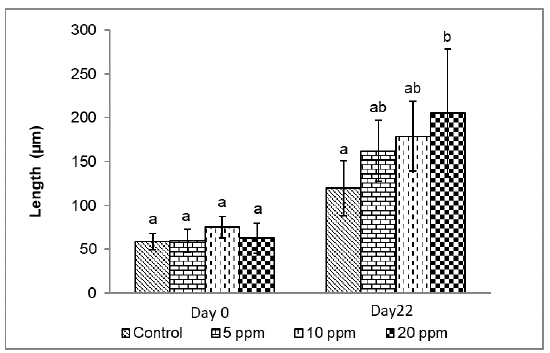
Figure 3 Length of intestinal folds of catfish Sorubim cuspicaudus larvicuture treated to different levels of inclusion of probiotics. Different letter indicate a significant difference (p<0.05).
Physicochemical parameters of water. The average values of the water quality parameters are presented in table 2. The higher temperature occurred in the 5 ppm treatment (26.8±0.6°C) and the lower in the control group (26.6±0.5°C), observing statistical difference between these values (p<0.05). The higher dissolved oxygen concentration was recorded in the control group (7.1±0.4 mg/l) and the lower in 5 ppm (6.7±1.0 mg/l), showing significant differences (p<0.05). For the parameters pH, electrical conductivity, dissolved solids, hardness and total alkalinity, the higher averages occurred at 20 ppm treatment, while the lower were at 5 ppm (Table 2). The most elevated turbidity (7.7±0.2 NTU) was recorded in the 20 ppm treatment and the lowest (0.6±0.25 NTU) in the control group, observing a statistical difference between these values (p<0.05).
Table 2 Physicochemical parameters of water during larviculture of catfish Sorubim cuspicaudus treated to different levels of probiotic inclusion.
Different letters in the same column indicate significant difference (p<0.05).
Microbiological analysis of water.Figure 4 presents the counts of colony-forming units (CFU) in the culture water in the different treatments. At the beginning of the experiment, a higher number of CFU was observed at 20 ppm (138.7±81.9 CFU/ml x 103), being statistically different from the control group and at 5 ppm (p<0.05). At the end of the experiment (day 22), a higher CFU concentration was recorded in the treatment with 20 ppm inclusion (83.5±64 CFU/ml x 103) without observing a significant statistical difference when the probiotics were included at 5 ppm (36.5±16.0 UFC) and 10 ppm (55.0±29.5 UFC), but with the control group (5.3±1.2 UFC) (p<0.05).
DISCUSSION
The results of this study suggest that the mixture of probiotics (50% Bacillus subtilis - 50% Bacillus licheniformis) supplied in the culture water benefited effect the catfish larviculture. The larvae with 20 ppm inclusion of probiotics showed better growth (Gw, GL, G) than the larvae of the control group and the other treatments (5 and 10 ppm). Some authors maintain that probiotics promote digestive activity early, through the release of enzymes (proteases and amylases), and facilitate the production of vitamins, prevent intestinal disorders, and promote and improve the digestibility of food (Zhang et al., 2010; Avella et al., 2012; Carnevali et al., 2017; Interaminense et al., 2018; Van Doan et al., 2018).
This action of the probiotics possibly accelerated the growth of S. cuspicaudus larvae, especially in the treatment with 20 ppm inclusion. In other studies, similar effects have been observed in the parameters of productive performance as in the herbivorous carp Ctenopharyngodon idellus when 2.4x107 CFU/g of B. subtilis were added for 42 days (Tang et al., 2019). In Oreochromis niloticus, the inclusion of three probiotic bacteria in a 1:1:1 ratio with 1x108 CFU/ml of Bacillus velezensis TPS3N, B. subtilis and B. amyloliquefaciens improved final weight, weight gain and feed conversion ratio. In addition, the probiotics separately also improved the final weight and weight gain compared to the control (Kuebutornye et al., 2020). A better productive performance has also been reported in crustaceans such as M. rosenbergii, when 9.35 x 108 CFU/g of B. licheniformis MTCC 429 was added for 90 days (Sudha et al., 2019) and in L. vannamei with the addition of 109 CFU per kg/56 days of B. subtilis (Tsai et al., 2019).
It is possible that, in the present study, the interaction of B. subtilis and B. licheniformis in the larviculture of S. cuspicaudus has potentiated the individual effect of each one of the probiotics, and thus explains the better productive performance obtained in the treatments with greater inclusion of probiotics (10 and 20 ppm).
Among the beneficial effects attributed to probiotics of the genus Bacillus is the generation of bacteriocins, which can exert a favorable impact on the growth and resilience to pathogenic microorganisms in aquatic organisms (Soltani et al., 2019; Ringø et al., 2020) which could be considered among the factors that improved the growth of the catfish larvae treated with probiotics.
In this study, introducing Bacillus subtilis and Bacillus licheniformis into the culture water prompted the proliferation of intestinal folds. This expansion likely augmented the nutrient absorption region and presumably influenced the larval intestinal well-being. The histological analyses of the catfish's digestive tract indicated an increasing trend in the length of the intestinal folds as the inclusion of Bacillus increased. A potential advantage of extending the length of the intestinal folds is the likely enlargement of the intestinal absorptive surface (Cao et al., 2019; Sewaka et al., 2019; Kong et al., 2021). In the catfish larvae, the length of the intestinal folds increased, with notable structural advancement in treatments supplemented with probiotics (5-20 ppm) regarding the control group. Comparable findings have been noted in Channa argus larvae when their diet was enriched with Lactococcus lactis, Enterococcus faecalis, or their combination (1x108 CFU/g); these larvae exhibited a significant increase in intestinal fold height compared to the group without probiotics (Kong et al., 2021). The intestine holds vital significance in nutrient digestion and absorption in fish, and the integrity of its tissue structure significantly impacts animal growth (Nicholson et al., 2012; Kong et al., 2021). Parameters such as muscle thickness, height, and fold width indicate intestinal health in aquatic organisms (Kong et al., 2021).
In the present study, in larvae treated with probiotics, a greater presence of goblet cells was recorded, particularly in the middle portion of the intestine and the presence of multiple epithelial cells with small intracytoplasmic vacuoles, as well as greater development of the esophagus and stomach. Similar results were obtained in tilapia (Oreochromis niloticus) fed with diets supplemented with enzymes and probiotics (20 ppm) containing Bacillus subtilis, Bacillus licheniformis and Bacillus pumilus, finding a greater number of goblet cells and a greater diameter of intestinal folds (Adeoye et al., 2016).
Even though studies affirm that applying probiotics, including bacteria of the Bacillus genus, is a beneficial practice for aquaculture since it improves animal welfare and the immune system, increasing resistance to diseases (Interaminense et al., 2018). There are also reports of adverse effects, such as those in Cerezuela et al., (2012) in sea-bream Sparus aurata (50 g of weight), who reported signs of edema, intestinal inflammation and decreased height of the intestinal folds when they were treated with Bacillus subtilis (1x107 CFU/g) in the food; which suggests that the effect of probiotics is species-specific and therefore it is necessary to evaluate the probiotic, the dosage and route of application for each species.
In the present study, there was no significant difference in survival and resistance to stress in the different treatments, possibly because the culture conditions were adequate in all the evaluated treatments. Similar results have been found in other studies. For example, with juveniles of Amphiprion ocellaris, Paixão et al., (2020) found that at the highest concentration of Lactobacillus plantarum (1x108 CFU/g), the productive performance parameters improved without affecting survival. The larval phase of aquaculture species is critical and with high susceptibility to diseases given the poor development of the immune system (Prieto-Guevara et al., 2015; Aerts et al., 2018); therefore, increasing disease resistance in larviculture and enhancing feed efficiency has led to the use of probiotics as a prophylactic alternative (Sorieul et al., 2018; Ringø et al., 2020).
However, an adequate dosage of probiotics improves animal welfare conditions and water quality and allows for obtaining animals of excellent quality and resistance (Midhun et al., 2019; Mukherjee et al., 2019; Paixão et al., 2020). It has been reported that adding 2x108 CFU/g of B. subtilis for 56 days increased resistance to stress to disease caused by A. hydrophila in the sturgeon Acipenser dabryanus (Di et al., 2019).
Cai et al., (2018) found that the probiotics B. licheniformis and B. flexus improved water quality in L. vannamei postlarvae cultures. Ringø et al., (2020) stated that spore-producing bacteria such as Bacillus can be supplied directly in the culture water because it is an efficient and economical transport route and improves water quality. In the present study, the electrical conductivity, dissolved solids, and turbidity increased significantly in the treatments with 10 and 20 ppm inclusion of probiotics, possibly caused by metabolites or excess of microorganisms.
In addition, the application of probiotics influenced the number of microorganisms present in the culture water, being higher (p<0.05) when it was included at 20 ppm compared to the control group. Nevertheless, although the CFU showed higher values when the probiotics were included at 20 ppm, these values did not show a statistical difference to those registered when the probiotic was included at 5 and 10 ppm (p>0.05). These results suggest that the inclusion of probiotics influenced the concentration of microorganisms (UFC/ml), which affected the increase in the length of intestinal folds. Other studies have reported similar results (Gisbert et al., 2013; Carnevali et al., 2017; Sewaka et al., 2019; Kong et al., 2021).
CONCLUSIONS
Based on the findings of this study, it can be concluded that a combination of Bacillus subtilis and Bacillus licheniformis in a 1:1 ratio holds promise as a beneficial supplementation strategy in the larviculture of S. cuspicaudus. This supplementation positively impacts on development, growth, and overall productive performance. Notably, it contributed to the enhancement of microbiota, gastrointestinal tract development, and particularly the elongation of intestinal folds.