INTRODUCTION
The cinnamates are UV filters that absorb around 310nm due to the unsaturated carbons conjugated system and, the presence of a methoxy group broadens the absorption range up to 350nm (Holser, 2008; Shaath, 2010). A typical example in commercial sunscreens is the octyl methoxy cinnamate (OMC) ester produced from 4-methoxycinnamic acid (4-MCA) and 2- ethyl-hexanol (Lee et al. 2006). Changing the lineal alcohol by glycerol leads to the 4-methoxycinnamoylglycerol (4-MCG) ester, which is more hydrophilic than the OMC, widening the range of solubilization vehicles than can be used during the sunscreen formulation, preserving the absorption ability of these cinnamates. The 4-methoxycinnamoylglycerol (4-MCG) is in form of mono- and di-esters when the esterification reaction is carried out in the presence of p-toluenesulfonic acid (p-TSA) (Holser, 2008; Holser et al. 2008). An improved conversion yield was reported by enzymatic synthesis using lipase, in addition to the prevalence of the monoester at short reaction times and less harmful side effects compared with the chemical route (Patil et al. 2011). It is well-know that lipases act as estearases in non-aqueous reaction media (Bernal et al. 2018; Fernandez-Lafuente, 2010), including the esterification of cinnamic acid (Patil et al. 2011) and/or glycerol (Yesiloglu & Kilic, 2004) and the obtained products have different industrial applications (Naik et al. 2010). The lipase activity and selectivity depend on water activity (Lee & Parkin, 2001) and solvent polarity (Kuo & Parkin, 1996) of the reaction media. Additionally, this selectivity can be modulated by immobilization in solid supports, as reported for different lipases adsorbed in Celite, for which the lipid modifications is selective to the size of the unsaturated carbon chains (Lee & Parkin, 2001). Moreover, their immobilization helps to their implementation at industrial level, allowing the easy recovery from the reaction medium in batch processes and increases the reuse cycles on packed bed continuous bioreactors (Babaki et al. 2016). The lipase B from the Candida antarctica yeast, immobilized on acrylic resin is commercially available (Novozym 435) and tested for the synthesis of 4-MCG (Patil et al. 2011) and other cinnamates (Lee et al. 2006) at temperatures between 40 - 60ºC.
The lipases from the thermophilic Thermomyces lanuginosus fungus is attractive for synthesis of different products through the esterification reaction, because it is thermophilic and active in a wide pH range (Fernandez-Lafuente, 2010). Its immobilization has improved its catalytic behavior in this kind of reaction due to the interfacial activation (Bassi et al. 2016; Zhou et al. 2012), thermal and conformational stabilization (Bernal et al. 2018; Matte et al. 2014) conferred by the support. Moreover, its immobilization by one-pot silica sol-gel process in the presence of polyethilenimine (PEI) is so simple for obtaining a biocatalyst with high protein load (90%), encapsulation yield (47%) and 247-times more stable at 65°C than the soluble enzyme (Escobar et al. 2018), which could increase the conversion degree of 4-MCA and glycerol during the esterification reaction at 65ºC. This immobilized-lipase has been not tested yet for production of 4-MCG.
On the other hand, we hypothesized here that the production of 4-MCG in form of nanoparticles could be another advantage for using this UV-filter in the sunscreen formulations. This is inspired in other reports that show the use of nanoparticles in sunscreens (Santos et al. 2019), evaluated according to the harmonized safety assessment guides (Katz et al. 2015), avoid the UV filters penetration through the skin and systemic cytotoxicity (Nohynek & Dufour, 2012). The free glyceryl entities and hydrophilic character of the 4-MCG can be exploited for its incorporation in N-Succinyl-chitosan (NSC) nanoparticles during the ionotropic gelation in the presence of tripolyphosphate (TPP). A low modification degree of NSC ((28%) and mixtures with non-modified chitosan favored the formation of nanoparticle with sizes lower than 160nm by ionotropic gelation and the purification by Ultrafiltration gave a monodisperse distribution (Monsalve et al. 2015). The NSC nanoparticles have been used as carrier of hydrophilic molecules such as encapsulated polyphenols (Palacio et al. 2020), grafted catechols (Sahatsapan et al. 2019) and lipoproteins (Zhang et al. 2014).
The objective of this paper was to demonstrate that esterification of 4-MCA and glycerol mediated by the immobilized-lipase of T. lanuginosus is selective towards 4-MCG monoester UV filter, whose chemical characteristics favor its incorporation in nanoparticles, during the ionotropic gelation of NSC.
MATERIALS AND METHODS
Esterification reaction. This was carried out by enzymatic synthesis, using the immobilized-lipase biocatalyst prepared by the one-pot silica/PEI sol-gel strategy (0.4mg mL-1 of lipase with 1300 - 1700U mL-1, 3.1 wt% PEI, pH 5.5, 50°C, 24h) (Escobar et al. 2018). Before its use, its kinetic parameters (Michaelis Menten constant, Km and maximum velocity, Vmax) and diffusion restrictions were measured by p-NPB and fluorescein dilaurate tests, respectively (Soto et al. 2017) and reported respecting to the soluble enzyme (Km = 4.92mM, and Vmax = 8.03mM min-1).
The esterification between dry glycerol and 4-MCA (5:1mmol ratio) was made in hexane or t-amyl alcohol (18mL) at 65°C (reflux) in the presence of 7mg of the immobilized-lipase, under magnetic agitation for 24h (eq. 1).
At the end, the liquid phase (that contains the reaction products) was separated from the biocatalyst by filtration. Both phases were characterized by different methods, described below. A partial purification of the produced esters from unreacted 4-MCA was carried out by liquid-liquid extraction, by using carbonate solution before to be submitted to characterization by HPCL, FTIR, 13C-NMR and UV-Vis analysis. The same esterification reaction was made in the presence of p-TSA (Holser, 2008) instead of the enzyme for making comparisons.
Preparation of 4-MCG nanoparticles. The incorporation of 4-MCG to NSC was carried out for preparing 4-MCG nanoparticles by ionotropic gelation. The NSC was obtained by the reaction of chitosan acetate (200mg) and succinic anhydride (39mg) in water (30L) under the conditions described by Monsalve et al. (2015) (eq. 2), for having a derivate exhibiting (28% of amine substitution degree and isoelectric point at pH = 7.2.
Then, 50mg of this NSC derivate in water (30mL) was put in contact with 4-MCG (12mg) during 24h under magnetic agitation. At the end, the product was dialyzed against water, lyophilized and characterized by FTIR.
After that, 5mg of this powder was dissolved in 1% acetic acid (5mL) by sonication during 1min (10 s ON and 10 s OFF, amplitude 90%), pH raising up to 5.0 and sonication under the same conditions. The tripolyphosphate (TPP) dissolved in miliQ water (1mg/mL, pH 7.0) was added at 25°C, varying the weight ratio for modulating the nanoparticle size and its distribution. The samples were purified by ultrafiltration (1 kDa cellulose membrane) and centrifugation at 4000rpm for 10min before the characterization by DLS. The quantification of 4-MCG in the nanoparticles was made by Uv-vis spectrometry by using a calibration curve represented by the equation Abs = 19483C+0.1521 at 310nm.
Characterization methods. All samples synthetized in this work were analysed by transmittance FTIR in a ZnSe plate in a Perkin Elmer spectrophotometer (450 - 3500cm-1 and 16 scans) for obtaining information about the ester formation between 4-MCA and glycerol, and the obtention of NSC. The chemical identity 4-MCG was corroborated by liquid 13C-NMR (Bruker Ascend III HD 600 MHz spectrometer with 5mm TXI probe) for which, the sample was dissolved in deuterated methanol. The conversion percentage of 4-MCA to 4-MCG was calculated from HPLC results (Agilent HPLC-DAD/FLD instrument, UV diodes detector, mobile phase methanol: H2O (70:30), C18 ZORBAX SB 5um, 250mm x 4.6um column). The peak area (for the pure 4-MCA and after reaction) was assumed proportional to the weight percentage of the 4-MCA for making the calculations. The 4-MCG molar extinction coefficient at 310nm was determined from the analysis 4-MCG solutions in methanol (concentrations between 2x10-7 to 2x10-4 M) by UV/Vis spectroscopy (Perkin Elmer model lambda 25 instrument). The same analysis was made for OMC solutions, under similar conditions. The size of 4-MCG-NSC nanoparticles was determined by Dynamic Light Scattering (Horiba LB 550).
The catalytic activity of the immobilized-lipase biocatalyst was measured by p-NPB UV/Vis activity assay (Escobar et al. 2018) before and after its use in the 4-MCG synthesis. The preserved (residual) activity was reported as percentage respecting to the initial activity.
RESULTS AND DISCUSSIONS
Esterification of 4-MCA and glycerol mediated by immobilized-lipase solid biocatalyst. The synthesis of 4-MCG was made at 65°C by esterification of 4-MCA and glycerol, catalyzed by immobilized-lipase in non-aqueous solvent. This biocatalyst was chosen because: (i) its preparation is very simple and reproducible by an one-pot silica/PEI sol-gel strategy (0.4mg mL-1 lipase, 3.1 wt% PEI, pH 5.5, 50°C, 24h); (ii) it is 247-times more stable at 65°C than the soluble enzyme (Escobar et al. 2018); (iii) it exhibits higher specific activity (600U g-1) (Escobar et al. 2018) than that immobilized by adsorption on mesoporous organosilicas or organic supports (Cipolatti et al. 2016); (iv) the solid siliceous solid support allows its easy recovering from the reaction media and the subsequent reuse. This is demonstrated here, retaining around 50% of activity after 4 catalysis cycles in hexane at 65°C (Figure 1A). This has a positive impact in the cost/effective balance of the organic synthetic processes.
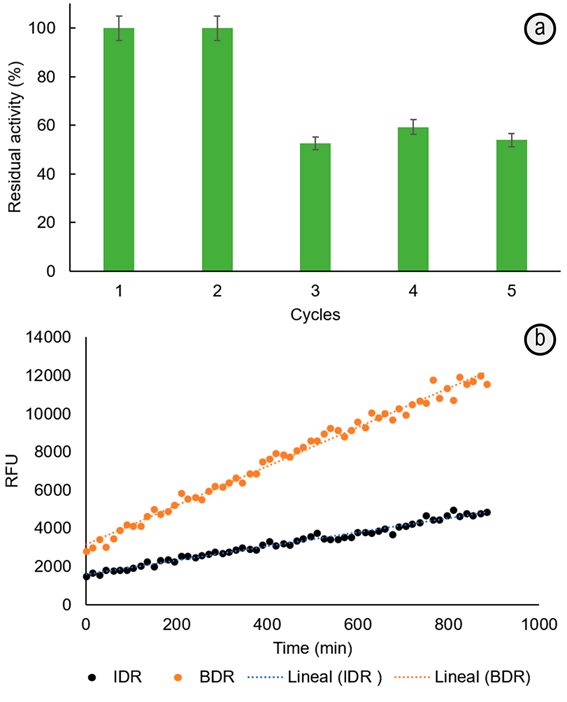
Figure 1 a. Reuse assay of immobilized-lipase biocatalyst in hexane at 65°C. The catalytic activity measured by p-NPB test. b. Diffusion test: Relative Fluorescence Units per minute (RFU, measured by Confocal Microscopy) from 0.1mM fluorescein dilaurate hydrolysis in the bulk (BDR= 10.4±0.7RFU min-1) and into the particles with immobilized-lipase (IDR = 3.6±0.1RFU min-1, average from 10 particles). All measurements were made by duplicate and the corresponding standard deviations are indicated.
On the other hand, the hexane and t-amyl alcohol were tested as non-aqueous solvents. The conversion of 4-MCA to 4-MCG, determined from HPLC analysis (Figure 2), is 14-times higher in hexane than in the t-amyl alcohol. Although the substrates are more soluble in later solvent, the hexane favors the shift of the hydrolysis-esterification equilibrium towards the ester formation. It is because its non-polar nature contributes to the lipase interfacial activation, and therefore, the improved interaction of the substrates with the active site of enzyme for their catalytic esterification (Patil et al. 2011; Bastida et al. 1998).
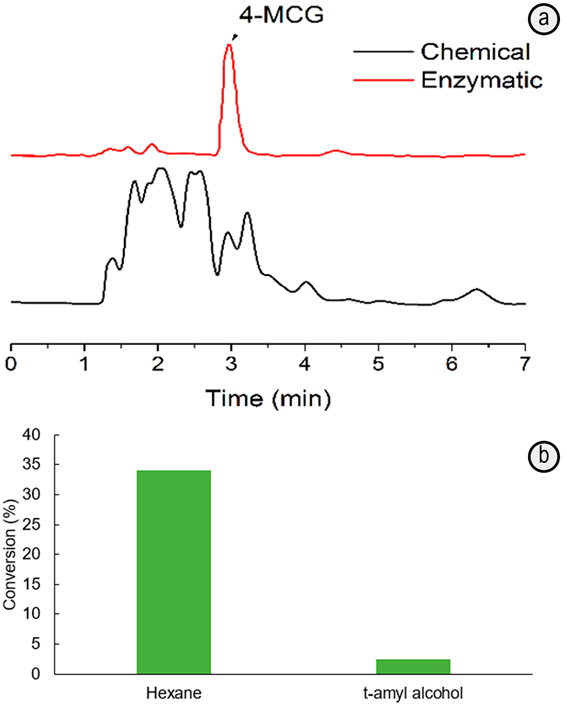
Figure 2 HPLC chromatograms for samples obtained by esterification of 4-MCA and glycerol mediated by p-TSA (Chemistry synthesis) or immobilized-lipase (Enzymatic synthesis) in hexane at 65ºC. a. Conversion degree of 4-MCA into 4-MCG mediated by immobilized-lipase biocatalyst at 65ºC in hexane or t-amyl alcohol; b. The data were obtained by triplicated and the significant difference evaluated with ANOVA test at (=0.05.
The HPLC chromatogram for the sample obtained after esterification in hexane at 65ºC by using the immobilized-lipase exhibits a major component (retention time (3min), well-solved from other traces peaks. In contrast, the sample prepared in the presence of p-TSA as catalyst and keeping constant the other parameters (including the HPLC analysis conditions), has a more complex chromatogram (Figure 2A). The relative contribution of the peak (3min, corresponding to 4-MCG, is more important in the sample prepared in the presence of the enzyme (78.56%) than with p-TSA (8.14%), even some 4-MCA and solvent traces could be present in both cases.
The chemical characterization of both samples by FTIR after purification by liquid-liquid extraction in the presence of carbonate solution (Holser et al. 2008) (Figure 3A) indicates the ester formation by the presence of carbonyl (-COOR) signals at 1700cm-1, which is shift to 1740cm-1 due to the presence of the unsaturated and aromatic carbon conjugated system (Holser, 2008) and C-O stretches in the 1300-1000cm-1. The band at 1688cm-1, typical of -COOH group in the cinnamic acid (Patil et al. 2011; Ferenc et al. 2012), disappears in the sample synthesized by the enzymatic pathway. Meanwhile, it is still contributing to the widening of the band centered around 1700cm-1 in the sample prepared with p-TSA (Figure 3A). This can be correlated with the higher consumption degree of the 4-MCA in the reaction mediated by the immobilized-lipase compared with that in the presence of p-TSA. The FTIR spectra also show the band at 3200-3600cm-1 (Figure 3A) corresponding to hydroxyls, which comes mainly from the glyceryl groups (Patil et al. 2011).
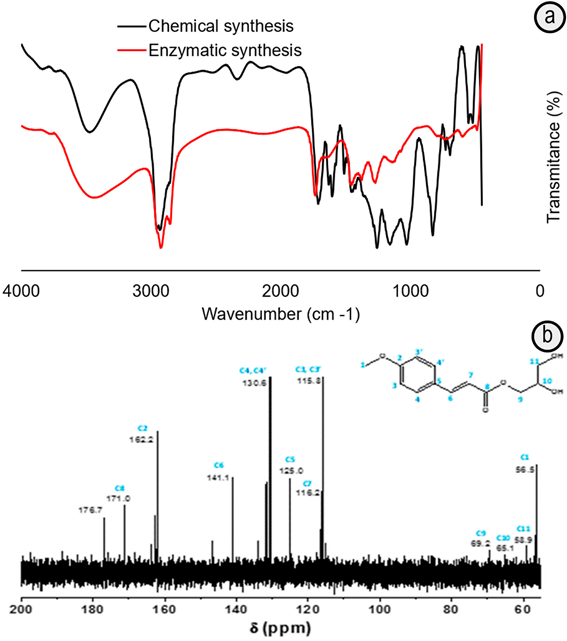
Figure 3 FTIR a. and 13C NMR b. spectra of samples obtained by esterification of 4-MCA and glycerol mediated by immobilized-lipase biocatalyst (Enzymatic) or p-TSA (Chemical) in hexane at 65ºC and purified by liquid-liquid extraction.
The signals of the 13C-NMR spectrum corroborate the ester formation by the shift at 171.0ppm (Figure 3B), characteristic of C=O ester bond (Sun et al. 2013). There is also a chemical shift at 178.0ppm of C=O bond of carboxyl acid, possibly, due to the presence cinnamic acid traces, evidenced in the HPLC chromatogram (Figure 2A).
These results indicate that the use of the immobilized-lipase biocatalyst avoids the formation of several by-products (such as monoester, diester, triester, degradation products), commonly found in the esterification of glycerol and cinnamic acid derivates in the presence of p-TSA (Holser, 2008; Lee et al. 2006).
The monoester could be formed through the primary hydroxyl, favored by the short reaction time (Holser et al. 2008), specificity and hyperactivation of the immobilized-lipase from T. lanuginosus in non-aqueous solvents (Bassi et al. 2016; Fernandez-Lorente et al. 2008). Moreover, the decrease of the immobilized-lipase affinity and maximum reaction velocity for substrates such as p-NPB (1.88- and 0.88-times higher Km and lower Vmax, respectively than for the soluble one) indicate that esterification product can be less prone to hydrolysis and formation of degradation products during the reaction time. The morphology, porosity and composition of the solid matrix make difficult the substrate mass transfer towards immobilized-lipase and, contributing also to the lowering of the hydrolysis rate. The Bulk/Intraparticle hydrolysis rates ratio > 1 (Figure 1B), measured from a confocal microscopy diffusion experiment, point out the mass transfer restrictions imposed by the solid support. These have consequences on the substrate interactions in the active site (Cipolatti et al. 2016; Soto et al. 2017) of the immobilized-lipase and therefore, affects the Km and Vmax kinetic parameters. The important thing in this work is that the tortuosity of the solid matrix could also hinder the formation of bigger by-products (diester, triester) or at least, lowering their diffusion up to the bulk solution. The presence of almost a unique product, with a higher conversion degree compared with that obtained by using C. antarctica lipase B (Novozym 435, immobilized on acrylic resin) as catalyst (Patil et al. 2011) allow a simple and faster purification process.
Absorption properties of 4-MCG and nanoparticle formation with N-succinyl-chitosan. The UV spectrum of 4-MCG was measured between 200-400nm (Figure 4), showing three main absorption bands in a wide UVB region (240-340nm), like those observed for the OMC molecule (Hanson et al. 2015). These bands are associated with transition π-π* in the near UV range. The molar extinction coefficient (ε) for 4-MCG at 310nm was 19483 M-1cm-1. This value is higher than that measured for the OMC (ε=12600 M-1cm-1), indicating a higher quantum efficiency of the 4-MCG. Taking into account that the ε4-MCG is in the range of values reported for other molecules used as UV filters (Shaath, 2010), the 4-MCG can be promissory for being used in sunscreen formulations, with the advantages of having glyceryl functional groups in its structure (Patil et al. 2011). They confer a higher hydrophilic character to the 4-MCG compared with the OMC, lowering the skin permeability, which can help to avoid systemic toxicity associated to the absorption of some sunscreen active ingredients (Nohynek & Dufour, 2012). On the hand, the hydrophilicity allows its incorporation on NSC nanoparticles, which call the attention for cosmetic formulations (Santos et al. 2019).
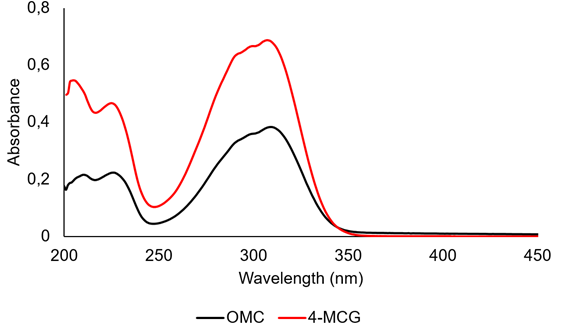
Figure 4 UV spectra of 4-methoxycinnamoylglycerol (4-MCG) and Octylmethoxycinnamate (OMC) measured in methanol.
In this case, the NSC was obtained by modification of chitosan with succinic anhydride. The FTIR spectrum (Figure 5) exhibits new bands at 1576cm-1 and 1465cm-1 from the amide II and -COOH symmetric stretching vibrations, which evidence the formation of NSC (Palacio et al. 2020; Sahatsapan et al. 2019). The absorption peaks at 1632cm-1, 1512cm-1, and 1385cm-1 can be attributed to band amide I, with the presence of the C=O stretching. The band at 1154cm-1 corresponds the stretching of C-O-C and the band at 1090cm-1 involved the C-O stretching.
The nanoparticles were prepared through ionotropic gelation method, varying the NSC:TPP weigh ratio (Table 1). The size of the nanoparticles decreased when the TPP concentration was increased, due to the increased cross-linking of the polymer. The NSC:TTP ratio equal to 1:1 lead to nanoparticles with the narrower nanoparticle size around 185nm. These are a little bigger than those obtained with NSC by ionotropic gelation (160nm) (Monsalve et al. 2015) because of the presence of 4-MCG. This preserved the UV absorption ability, because the aromatic ring is not affected during the polymer crosslinking. The incorporation degree was around 16.1(4.5% and there is not a significant variation when the TPP was changed, showing that it is more dependent on the interaction of the 4-MCG with the NSC before the formation of the nanoparticles, whose conditions were kept constant for all the samples. These 4-MCG/NSC interactions proceed through the protonated amines remaining on the NSC ((28% of substitution degree).
Table 1 Size of nanoparticles prepared by ionotropic gelation method with N-succinyl chitosan-4MCG and TPP at 25ºC and pH 5.0. (Samples obtained by triplicated, size reported as mean ( standard deviation). Significant differences evaluated with ANOVA test at (=0.05.
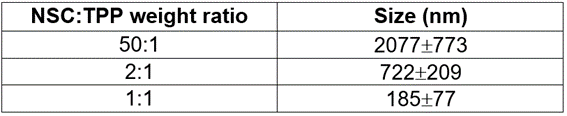
In conclusion, the immobilized-lipase from T. lanuginosus, exhibiting high activity and thermal stability, allowed the esterification reaction of 4-methoxycinnamic acid and glycerol, at 65ºC in hexane. It was a clean pathway, reaching (34% substrate conversion, and the reuse of the solid biocatalyst from the reaction media. This allowed simplifying the purification process.
The free glyceryl entities conferred hydrophilicity to the 4-methoxycinnamoylglycerol product. This favoured its incorporation on N-succinylchitosan and promoted the formation of nanoparticles with size around 185(77nm by ionotropic gelation. The UV absorption capacity of 4-Methoxycinnamoylglycerol in form of nanoparticles is promissory for sunscreens formulations.