INTRODUCTION
Soil organic carbon (SOC) comes as a stage of the global cycle of this element and it is the largest sink of the biosphere, as it stores twice the amount of the atmosphere and three times what is found in biomass (1550 vs 750 vs 550Pg, respectively) (Bolin & Sukumar, 2000). This way, SOC is an important sink against climate change, which is why many projects involve soil, such as afforestation and reforestation (Pearson et al. 2005). Knowing SOC reserves and the impact of land use systems in this edaphic feature allows managing the landscape in order to increase the offer of this ecosystem services. Carbon contained in biomass then moves into necromass due to tissue senescence. After that, part of that carbon becomes CO2 and it is released into the atmosphere, but another part merges with soil as organic matter, which contains carbon (FAO, 2017).
In addition to help as a climate change mitigator, SOC contributes to improve several biological and physicochemical properties of soil (Agostini et al. 2014). Those authors state that SOC favors soil aggregation and intervenes in pore distribution, favoring humidity retention, water and gas movement in the soil, increases cation exchange capacity, and tampon capacity over soil reaction (pH). SOC also works as an energy source for heterotrophic organisms that live there.
Some carbon projects, such as Clean Development Mechanisms, and volunteer markets, have included soil as one of the components to generate a carbon addition and reduce emissions of greenhouse gases (GHG). The “4 by 1000” initiative, which identified the key role of soils for food security, the increase of carbon for soil fertility, came out recently in the COP21, adapting agriculture to climate change without compromising food production (https://www.4p1000.org/es). On this matter, the Colombian State has started the implementation of the Colombian Strategy of Low Development on Carbon Emissions, identifying mitigation actions in the sector to reduce GHG and, with this same effort, generate environmental, social, and economic development in the rural area (Mendieta, 2011).
There are still several efforts to develop in order to take advantage of soils with high capacity to capture atmospheric carbon. These soils are representative of the valleys of the great rivers that are born in the Eastern Mountain Range and that, arriving in the plains, form alluvial fans with the material they transport. The foothill is a geographic area along the edge of this mountain range and it includes an ecological transition area between woodland forests and the savannah with sedimentary soils, with a higher fertility than the one for those deeper into the Orinoquia (IGAC, 2003).
Quantifying the ability of plants to store SOC for a long term is important for climate change mitigation and soil fertility improvement (Mathew et al. 2020). The goal of this study is to estimate SOC storage in the first 30cm of depth in the most predominant land uses in the foothill of the municipality of Yopal, which covers 33.925ha (Alcaldía de Yopal, 2013). Likewise, it is intended to estimate what the change in SOC reserves could be if land use were altered, so that the potential of CO2 emissions or carbon additionality in this component could be tested. Results will work in the formulation of policies and projects that will include this ecosystem service as a way to improve the income of these rural citizens.
MATERIALS AND METHODS
Study area. located in the Corregimiento of Tacarimena, consisting of eight villages: El Nocuito, Manantiales, Sirivana, Palomas, La Calceta, La Manga, Tacarimena, and El Tiestal (Figure 1). The Corregimiento of Tacarimena has a surface of 339,25km2, it is located to the southwest of the urban area of the Municipality of Yopal (Alcaldía de Yopal, 2013), between the coordinates 5°20’12” N; 72°10’05” W; 5°15’07” N; 72°10’14” W; 5°22’12” N; 72°25’10” W; 5°19’46” N; 72°15’07” W.
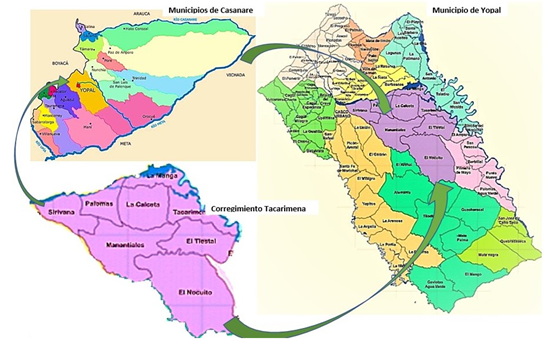
Figure 1 Location of the study area in Corregimiento of Tacarimena, municipality Yopal - Casanare, Colombia.
Soils in the area of study are located in the plains, composed of both fine and thick alluvial sediments that flow along the plain of the valley of Cravo Sur river. There, the local agricultural activity concentrates with plantain, cassava, and corn crops in traditional farming with some level of technology. This land unit is located in parallel with big hydric currents, which transport soils from the Eastern Mountain Range that renew periodically, thus having an incidence in fertility. The IGAC (2014) indicates that these soils are mildly acid, with a medium level of organic matter, low cation exchange capacity, medium to high base saturation, and natural fertility and limitations due to aluminum toxic content. The area consists of soils from the Fluventic Humic Dystrudepts-Typic Fluvaquents Association.
According to IDEAM (2018), weather in Yopal is warm-humid, with a mean annual precipitation of 2270mm, with a dry season (December to March), and a rainy season (April to October). The month with the highest mean precipitation is May, with 334mm, while the lowest is in January, with 9mm. Altitude of the area of study is located between 320 and 350m, and average temperature is around 26.9ºC.
Experimental Design. A random design with seven land uses was utilized: 1) agroforestry systems (AFS) of plantain with shade (AFS+plantain), 2) cocoa with shade (Co+S), 3) citrus (C), 4) low silvopastoral system (LSPS), 5) high silvopastoral system (HSPS), 6) gallery forests (GF), and 7) bush forest (BF), with five repetitions each. It was sought that these sampling units were in the same relief and showed similar soil and topography in order to avoid confused effect of treatments.
SOC estimation was performed at a 0-30cm depth, based on two variables: SOC concentration and bulk density (BD) (González-Molina et al. 2008; Alvarado et al. 2013). Gross fragment content was overlooked as it was too low (<5% volume). The first variable was estimated taking compound samples with 25 sub-samples per plot, which were studied in the soil laboratory from Universidad de los Llanos, using the Walkley & Black method (1934). BD was estimated with the known method of the volume cylinder, which was calculated as the relation between the weight of the dry soil and the internal volume of the cylinder.
In the simulation of the land use change effect on SOC, the same BD was used as baseline (that of GF), to compare based on a same soil mass and not volume, which can change when compacted and thus alter the results, meaning that an increase effect on SOC storage due to BD was avoided. This possible change was estimated as the difference of SOC between the current and future land use that may occur, taking values to CO2, with the 44/12 relation, that corresponds to 3.67. Positive values suggest additional carbon fixation, whereas negative ones indicate CO2 emission.
Statistical Analysis. A variance analysis with seven systems of land use as treatments and five repetitions was used before validation of hypotheses and an average comparison test with the LSD Fisher test (⍺ = 0.05), using Infostat.
RESULTS AND DISCUSSION
Bulk density and organic carbon concentration in the soil. BD changed significantly (p<0.05) among land use systems. BD of soils differs statistically (p<0.05), among GF, C, AFS+plantain, and HSPS. Thus, BD values between 1.33-1.48g/cm3 were found, being GF and BF soils the ones that show the highest value in this edaphic variable. On the contrary, HSPS, AFS+plantain, and C had the lowest BD. This physical variable of soils turned out similar among the HSPS, AFS+plantain, and C systems (1.33-1.35g/cm3), while Co+S, LSPS, and BF showed a BD between 1.40 and 1.43g/cm3 (Figure 2). This tendency was also evinced in the findings of Andrade-Castañeda et al. (2016), who found values of BD for riparian forests, riparian forest margins, and agricultural matrix (rice, pastures), of 1.7, 1.1, and 1.0g/cm3, respectively. This contrasts with the findings of Andrade et al. (2018), where no BD differences in agriculture and riparian forests were detected in the basin of Combeima river (Ibagué, Colombia). Agricultural activities caused a reduction of BD, which is possibly due to plowing activity on the soils. These results were not expected, since GF and BF have been the lowest anthropically intervened.
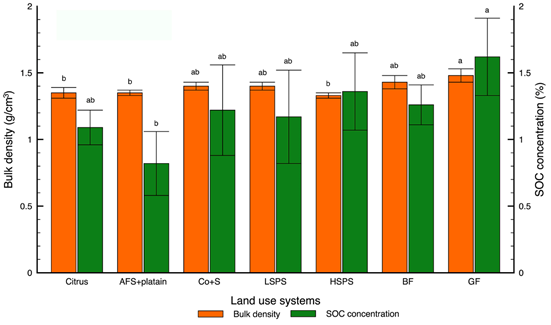
Figure 2 Bulk density and soil organic carbon (SOC) concentration in the land use systems of corregimiento of Tacarimena, municipality of Yopal. Error bars correspond to standard error. Means with common letter are not significantly different (p>0.05). AFS+plantain: plantain with shade; Co+S: cocoa with shade; LSPS: low silvopastoral systems; HSPS: high silvopastoral systems; BF: bush forests; GF: gallery forests.
Peña et al. (2009) estimated BD between 1.16 and 1.66g/cm3 in Tauramena, Eastern mountain range in Casanare, Colombia, and indicate that these values are considered as a limitation for normal radical development. Rivera et al. (2013) studied the BD in the Llanos Orientales from Colombia, where values of 1.11-1.42g/cm3 were found, which are very similar to the findings of this current study. Literature reports that BD increases due to the activities of repetitive work with agricultural machinery. However, the study is performed in areas of natural vegetation and perennial agricultural zones, which are not affected by recurrent mechanization or work. Difference of BD in the soils of the systems studied can be attributed to the materials that compose these soils and not to possible deterioration due to the land use and management.
SOC concentration also showed statistical differences (p<0.05) among land use systems (Figure 2). This variable showed a range between 0.82 and 1.62% in the layer of 0 to 30cm, being the soils of GF, HSPS, and BF the ones with the highest concentration (1.62, 1.36 and 1.26%, respectively) (Figure 2). On the other hand, AFS+plantain showed the lowest SOC concentration (0.82%). Likewise, SOC concentration differed significantly between the AFS+plantain and GF uses (p<0.05), but these are similar (p>0.05), between C, LSPS, Co+S, BF, and HSPS (Figure 2).
Andrade-Castañeda et al. (2016) report an SOC concentration of 1.7% in soils of dry riparian forests of Tolima, which is coherent with the current estimates for GF. Cantú-Silva & Yáñez Díaz (2018) reported SOC concentrations for bushes of 2%; grasslands, 19%; forest plantations, 1.7%; and agricultural areas, 1.3%, at depths between 5 and 30cm, in the municipality of Linares, in the state of Nuevo León, México; values that are similar to those found on the study of interest. On this current study, differences in SOC concentrations are attributed to the change of land use, caused by the development of mechanization and management activities, such as the application of supplies that deteriorate the vegetal cover and with it, carbon accumulation in productive systems. On the contrary, there are affectations in SOC percentage of BF and GF, thus improving their ability for carbon accumulation.
Organic carbon reserves in the soil. Statistical differences were detected (p<0.05) among the land use systems studied, which ranged between 33.4 and 72.7Mg C/ha (Figure 3). GF showed the highest SOC reserves (72.2 ± 13.8Mg/ha); whereas Co+S and C stored 50.6 and 43.9Mg/ha, respectively and silvopastoral systems had reserves between 49.2 and 54.1Mg C/ha (Figure 3). Significant differences (p<0.05) were found between the SOC reserves of GF and AFS+plantain. Meanwhile, C, LSPS, Co+S, BF, and HSPS stored similar values (p>0.05) and showed a range between 43.9 and 54.1Mg/ha. Mandal et al. (2020) state that agriculture is a dominant land use and that carbon sequestration under different agroecosystems is an important option to fight climate change.
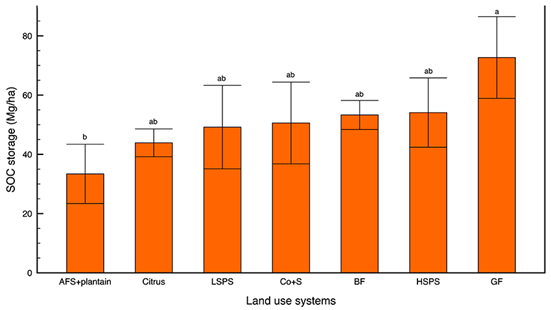
Figure 3 Soil organic carbon (SOC) storage in land use systems of corregimiento of Tacarimena, municipality of Yopal. Error bars correspond to standard error. Means with common letter are not significantly different (p>0.05). AFS+plantain: plantain with shade; LSPS: low silvopastoral systems; Co+S: cocoa with shade; BF: bush forests; HSPS: high silvopastoral systems; GF: gallery forests.
Values found in the study are coherent with the reports by Andrade-Castañeda et al. (2016) in dry riparian forests of Tolima, Colombia (65.6Mg C/ha). Ortiz et al. (2008) report SOC storage for AFS with Cordia alliodora and cocoa of 43-62Mg C/ha; and Arce et al. (2008) estimated 32Mg C/ha in these same systems in the area of Valles de Talamanca, in Costa Rica. Meanwhile, Poveda et al. (2013) report 47.5Mg C/ha, a value similar to the results of this current study. Tree density in the systems studied by Arce et al. (2008) was of 676 trees/ha, with the highest number for shade individuals, which is consistent with the results on this study in Co+S, where it was found that it stored 50.6Mg C/ha. SOC difference between AFS+plantain and GF is explained with the change in land use and the management activities performed on the first one. This management exposes soils to adverse conditions that cause SOC deterioration (Ussiri & Lal, 2013). This does not happen with soils occupied by GF and BF, which are dedicated to hydric conservation and regulation, reason why continuous losses of SOC would not be expected (Cosentino & Costantini, 2000).
SOC dynamics is related to the changes of organic matter and its evolution, conditioned to variables such as vegetation (incorporation of residues due to plant decomposition), soil properties (texture, structure, clay mineralogy, and PH), weather (temperature and humidity), which play a direct role in C incorporation and fixation (FAO, 2001). This process causes C storage, protected by fine particles, such as clay (Lal, 2012; Huang et al. 2014). Likewise, Rothon (2000) found a positive correlation between the stability percentage of aggregates and soil organic matter (SOM) content. Mathew et al. (2020) claim that SOC deposition is positively related to carbon translocation in the radicular zone.
SOC favors soil fertility and improves physical characteristics, since the particle aggregate improves pore distribution and the ability to retain and supply water to the plants. These features contribute to run-off reduction and susceptibility to drought (Lal, 2012).
Mineralization, lixiviation, and erosion cause around 20% of global CO2 emissions (Intergovernmental Panel on Climate Change - IPCC, 2007), expressed in the following terms: “Variation of greenhouse gases (GHG) and aerosols in the atmosphere, and variations in earth cover and solar radiation, alter the energetic balance of the climate system.” The most intense SOC losses take place in climates with high temperatures and humidity, like in the tropic (Crowther et al. 2016). This effect takes place in the area of interest in transient crop lands, given the climate conditions of Colombian lowlands, where altitudes below 400m, annual precipitation of 2,309mm, and average temperature of 26.9°C, are the specific conditions given in the area of the study.
Effects of land use change in C fixation of CO2 emission. A reduction in SOC sequestration was when found tree cover is diminished, as in the change of GF and BF, on agricultural crops (Table 1). The most positive land use changes to mitigate climate change take place when AFS+plantain moves to GF and HSPS, with additionalities of 130.3 and 88.0Mg CO2/ha, respectively (Table 1). In contrast, deforestation, to find areas for agricultural production, could cause emissions between 6.2 and 130.3Mg CO2/ha (Table 1). Several studies on land use changes in the Orinoquia region have detected alterations in SOC due to the conversion (Ramírez-Contreras et al. 2021), showing net carbon sequestrations or CO2 emissions (Rojas et al. 2018).
Table 1 Potential impact on soil organic carbon storage by land use changes (Mg CO2/ha), in el corregimiento of Tacarimena, municipality of Yopal - Casanare, Colombia.
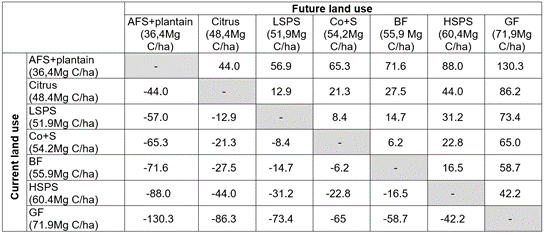
AFS+plantain: plantain with shade; LSPS: low silvopastoral systems; Co+S: cocoa with shade; BF: bush forests; HSPS: high silvopastoral systems; GF: gallery forests.
According to Ordóñez & Masera (2001), and Valenzuela & Visconti (2018), it is important to perform quantification studies of atmospheric carbon emission/sequestration in forest ecosystems or of land uses, with emphasis on the dynamics of land use change. This could permit estimation of carbon density associated to vegetation types and develop estimation and prediction methods for carbon emission or sequestration, under the dynamics of land use. Thus, GHG mitigation options could be offered in the short, medium, and long term. Meaning that carbon sequestration contributes to the mitigation of climate change.
In this context, Andrade-Castañeda et al. (2016) have studied and documented the potential impact due to land use changes, claiming that the biggest changes in SOC are held when pasture areas are changed into forest. On the other hand, when riparian forests are cut to establish rice fields, SOC tends to reduce, causing GHG emissions in 12.3Mg CO2/ha. In the semi-arid region of Brazil, Medeiros et al. (2020) found that conventional farming systems reduced SOC storage in the first meter of soil. In the basin of Combeima river, in Tolima, Colombia, Andrade et al. (2018) showed that land use systems without tree cover affect SOC through time. Likewise, Lozano Botache et al. (2011) claim that, currently, one of the main environmental problems addresses to coverage loss and, with it, to fragmentation of forests, alteration of soil resources; specifically, concerning erosion, to CO2 generation into the atmosphere, in terms of emissions.
The study allowed the assessment of how the land uses tested offer the ecosystem service of carbon storage intending to mitigate climate change. However, GF soils stored more SOC in contrast with AFS+plantain. HSPS is an important carbon sump that also allows cattle production at the same time.
Every use change would cause alterations in SOC storage. Land use changes that should be promoted to mitigate climate change can be moving from agricultural production systems to forest ecosystems, such as GF and BF. In silvopastoral systems, density increase of tree component enhanced SOC storage, with the consequent additionality in this ecosystem service. Pasture improvement and tree cover increase AFS-intended, can introduce them with carbon storage potential at the area of study level, in addition to having forests that bring protection of hydric resources. Management measures for sumps and CO2 sources that increase sequestration in the first cases and reducing emission in the second are required, so that conversion of sumps into sources is avoided.
In general, production systems that involve trees in proper densities are suggested as a guarantee in carbon sequestration and, with this, offer the possibility of mitigating climate change. Given that soils studied are representative of the Orinoquia foothill, results could be extrapolated to this area, which would provide an estimate of the importance of these land uses and changes in this ecosystem service.