1. INTRODUCTION
Soils are essential for agricultural purposes as they are the medium through which crops are grown. Soil quality is a crucial factor for human health, animal husbandry and the sustainability of all ecosystems. Globally speaking, soil quality is affected by the presence of potential toxic elements (PTEs), which is largely due to anthropogenic activity, particularly by agriculture use [1], [2], [3]. Indeed, soil may become polluted by heavy metals (HMs) and metalloids accumulating after receiving a significant number of pollutants from different land applications of fertilizers, animal manure, sewage sludge, pesticides and wastewater irrigation. Many farmers add large quantities of fertilizers to soils in intensive farming systems to provide adequate N, P and K supplies for crop growth. These fertilizers normally contain trace amounts of heavy metals (e.g., Cd, Pb, rare earths, etc.) that ultimately increase in soil [4]. For example, land application of biosolid materials is a common practice in many countries, normally by urban populations [5]. Thus, several common pesticides and fertilizers are fairly extensively employed in agriculture and horticulture [6], while certain animal waste types, such as poultry, cattle and pig manures, are also used in this way [7]. All this poses serious growing concern about the potential risk to human health via direct intake, bioaccumulation through the food chain and impacts on the ecological system.
Three different agricultural fertilizer categories are normally established: macronutrient fertilizer to supply major nutrients (N, P, K); macronutrient fertilizer to supply secondary nutrients [calcium (Ca), magnesium (Mg) and sulfur (S)] and micronutrients [copper (Cu), zinc (Zn), nickel (Ni), manganese (Mn), iron (Fe), molybdenum (Mo), boron (B), chlorine (Cl), sodium (Na), cobalt (Co) and silicon (Si)]. There is another classification based on essentiality of nutrients, where metals may be classified in this way: B, Cu, Fe, Mn, Mo and Zn as essentials; Co, Ni and V as beneficial; Cd, Cr, Hg and Pb as toxic. So, it should be noted that essential and beneficial elements can become toxic at high concentrations [8]. Heavy metals can be classified into two categories: essential (biometals) and nonessential (toxic metals). Essential metals (Co, Cr, Cu, Fe, Mn, V, Zn) at high levels can also have toxic effects on organisms, while toxic metals (Cd, Pb, Al) are toxic to human health and the environment even at low concentrations.
To understand this behavior, we must bear in mind that heavy metals in soils can appear in several forms: interchangeable (bound to organic and inorganic components), as soluble ionic forms in structural components of soil particles and in insoluble and/or precipitated states. Only the first form can be generally absorbed and used by plants in such a way that they also constitute an important point of connection between these elements and humans through the food chain and represent a human health risk [9]. The environmental risk of heavy metals depends on several factors, such as their total contents and soil characteristics. In any case several authors have shown that agricultural activity has become the main source of heavy metals in soils [3], [10]-[13].
According to Mahecha-Pulido et al. [14], soil studies in Colombia related to heavy metals have been conducted mainly in the Andean region. They have focused on the Departments of Cundinamarca, Antioquia, Valle del Cauca, Boyacá, Santander, Cauca and Nariño, and also on the Caribbean region. Here analyses have been performed to focus on the toxicological risk due to heavy metal concentrations in the middle and lower valleys of the Sinú River in the Department of Córdoba.
In the Orinoquia region, which is considered the main agricultural pantry of Colombia, very few studies have established the concentrations of these elements, or monitoring and follow-up plans in pursuit of sustainable soil resource use [3], [15]-[17]. Since this region represent unique soil and climate conditions on a global scale, our main objective was to take a look at the possible role of three agricultural production systems (oil palm, pastures, semi-annual crops) in the accumulation of some HMs in acid soils at Piedemonte Llanero in Colombia. In this way, the primary goals of this study were to: (1) provide a database for the studied HMs; (2) evaluate the effect of the agricultural production system on the concentration of metals; (3) gain a better understanding of the ecological risk assessment for the HMs that will contribute to the sustainable management of this vital hyperhumid area. For this purpose, the heavy metal concentrations in the soils of these three agricultural production systems were first determined, and later evaluated by means of the geo-accumulation index (I-geo), the contamination factor (CF) and the pollution load index (PLI). The ultimate purpose was for this information to become a basic tool to establish monitoring and follow-up processes in pursuit of sustainable soil resources management.
2. MATERIAL AND METHODS
2.1 Site description
The survey was conducted at Piedemonte Llanero, located in the central-eastern part of Colombia (latitude 03°58’26.2” N - 073°52’08.1” W; longitude 03°52’53.7” N - 073°06’43.7” W) (Figure 1). This area is located in the Acacias River Basin, which occupies approximately 93100 ha (Figure 1). Its highest point reaches 1800 m.a.s.l., and its lowest point lies at 200 m.a.s.l. [18]. Soils are also variable, and Inceptisols, Oxisols and Entisols are found [19]. The study area soil is strongly acidic in nature with mean pH values from 4.1 to 4.5, and organic matter content ranging from 4.4% to 0.3%. Rainfall is determined by the intertropical convergence zone (ITCZ), and its annual mean varies approximately from 6000 mm/year in mountainous areas to 2500 mm/year in flat piedmont areas. Temperatures vary between 22.5°C and 28.5°C. Nitrogen (urea), phosphate (superphosphate) and potash fertilizers, with organic manure, are applied to the farming areas in this zone.
2.2 Soil sample collection
Forty-seven farmland soil samples were collected in quintuples in August and October 2020 (Figure 1) following a multi-thematic model using the (Create Random Points) app in version 10.1 of the ArcGIS Software (ERSR, Redlands, CA, USA). At every sampling site, five subsamples in topsoils were mixed thoroughly to obtain a composite sample. Sampling was done at the 0-30 cm depth because the area is considered to be of agricultural importance, where HMs from anthropogenic sources accumulate [20]. Soil samples were air-dried, homogenized and passed through a 2-mm mesh sieve and placed inside a plastic bag to preserve them in the laboratory until the chemical analysis.
2.3 Sample analysis
The chemical and physico-chemical analyses of the fine earth samples were performed following standard procedures as follows: soil texture was determined by the hydrometer method [21] with three replicates. Soil pH was potentiometrically measured in H2O and 0.1 M KCl using a 1:2.5 soil: water suspension. Soil organic matter was determined as described by the Walkley-Black method following the protocol according to Jackson [22]. The chemical analysis was performed by acid digestion following the EPA 3050B method and the determination of HMs (Cr, Cu, Ni, Cd, Mn, Pb, Zn). Next 50 % v/v sub-boiling bidistilled HNO3 was added to all the samples and analytical blanks (Certified reference material of each element; 1000 mg L−1, Merck, Germany). Determination was performed using an Optima inductively coupled optical emission plasma spectrometer (ICP-OES; Perkin-Elmer 2100DV model). For quality assurance and quality control (QA/QC) purposes, accepted recoveries ranged from 70 % to 130 %. Standard reference material was used to ensure metal data reliability. Blanks (n=20) and duplicate samples (n=25) were evaluated for each set of samples during the assay method. The detection limits are 1 mg kg-1 for Zn, Cr, Cu, Ni, Mn, Pb and for Cd 0.2 mg kg-1. The relative deviation of duplicate samples was < 15 % for all the batch treatments.
2.4 Statistical analysis
Statistical methods were applied to analyze data using IBM SPSS 23.0 (Armonk, NY, USA) (IBM Corp 2015) and the real statistics resource pack for excel (Real Statistics ResourcePack for Excel 2019). Multivariate analysis methods (correlation analysis and PCA analysis) were used for source analysis and exploration. Basic statistical parameters were computed, such as mean, minimum, maximum, standard deviation (SD) and coefficient of variation (CV %). The Kruskal-Wallis H test was run at a 0.05 significance level.
2.5 Ecological risk assessment
Soil quality can be estimated by several indices. The I-geo, proposed by Müller [23], allowed environmental evaluations to be applied to the agricultural soils of the basin. This method evaluates enrichment of heavy metals by (1):
Where Cs refers to calculated values and Bn denotes background values. A factor of 1.5 was applied to control for variations in Bn values. Müller [23], García-Martínez and Poleto [24] and Trujillo-González et al. [25] classified enrichment levels into seven classes as: G0 uncontaminated if Igeo ≤ 0; G1 uncontaminated to moderately contaminated if 0 < Igeo < 1; G2 moderately contaminated if 1 < Igeo <2; G3 moderately to heavily contaminated if 2 < Igeo < 3; G4 highly contaminated if 3 < Igeo < 4; G5 very to extremely contaminated if 4 < Igeo < 5; G6 extremely contaminated if Igeo ≥ 5.
The CF was also used, which evaluates the direct relation between the determined concentration and the reference value and is represented by (2).
Where CFi. is the CF for metal i. Ci is the metal concentration in the sample; Coi denotes the background value of metal i. Hakanson [26] defined the following categories to interpret this index: low contamination (CF < 1), moderate contamination (1 < CF < 3), considerable contamination (3 < CF < 6) and very high contamination (CF > 6).
We employed also the PLI, [27], which is an index that can integrate more than two elements. It was calculated using (3).
Where CF corresponds to the CF of each considered element. Based on the PLI contamination levels, the classification standards were adopted as follows: nonpollution PLI (CF) ≤ 1; slight pollution 1<PLI (CF) ≤ 2; moderate pollution 2 <PLI (CF) ≤ 3; heavy pollution PLI (CF)> 3. For all the indices, the reference values proposed by Trujillo-González et al. [28] for the study area were taken into account.
Finally, Nemerov Integrated Pollution Index (NIPI) has been widely employed in assessing risk pollution potentials of metals in soils [29]. It was calculated using (4):
Where, PIave is pollution index average, while PImax is pollution index maximum. NIPI ≤ 0.7 = no pollution, 0.7 < NIPI ≤ 1 = warning line of pollution, 1 < NIPI ≤ 2 = low level of pollution, 2 < NIPI ≤ 3 = moderate level of pollution, NIPI > 3 = high level of pollution.
For all the indices, the reference values were the proposed by Trujillo-González et al. [28].
3. RESULTS AND DISCUSSION
As a consequence of the developing under tropical conditions, most of these soils have limitations for agriculture, such as acid, deep and highly weathered soils with low nutrient availability and high exchangeable acidity (Al3+), because they result in low cation exchange capacity and poor P availability [30].
Indeed, the granulometric distribution results (Table 1) showed that the soils cultivated with pastures and oil palm have a loamy-clayey texture, while the soils with semi-annual crops are loamy-sandy, loamy-clayey and loamy-clayey-sandy. These results agree with those reported by Trujillo-González et al. [31] and IGAC [32]. The soils with pastures presented a loam-clay-sandy (F-Ar-A) texture, the % of sand was 56.15, with 21.15 % silt and 22.70 % clay. In the oil palm crops, the results were 42.14 % sand, 26.64 % silt and 31.22 % clay for a loam-clay texture (F-Ar). In the semi-annual crops, a loam texture was found with 43.86 % sand, 30.33 % silt and 25.76 % clay.
Table 1 Descriptive statistics of pH, organic matter content and texture in the three agricultural production systems at Piedemonte Llanero in Colombia
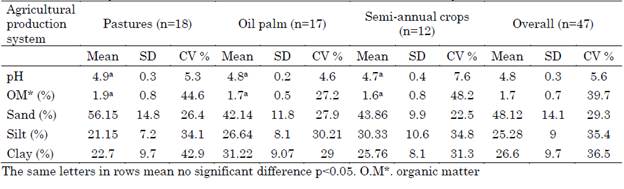
Source: created by the authors.
The soil pH for all the samples was strongly acidic (Table 1), with a mean of 4.8. Soils below pH 6.5 are considered acidic. According to the Kruskal-Wallis H test, there were no significant differences (p<0.05) between the pH values and the soils with the pasture, oil palm and semi-annual crop production systems. Mahecha-Pulido et al. [11] and Trujillo-González et al. [31] obtained similar soil pH values in the Piedemonte Llanero region. Organic matter presented variability with a CV % of 39.7 % and a mean of 1.73 (Table 1). For this variable, no significant difference (p <0.05) appeared in any of the studied soils.
In light of all this, carrying out fertilization and soil amendment practices seems necessary to make soil suitable for major crop development, to provide plants with the necessary nutrients and to lower soil acidity. Unquestionably fertilizer overuse can also provide soil pollution because it increases the concentration of a particular element or substance of environmental interest above its naturally-occurring concentration in soil.
The studied HMs have the same sequence in the pasture, oil palm and semi-annual crop systems and come in the following descending sequence: Mn > Zn > Cr > Ni > Cu > Pb > Cd. The Kruskal-Wallis H test showed no significant difference at 0.05 among the studied soils depending on the three crop types. A comparative analysis was also carried out using a box-plot for the three production systems (pastures, oil palm, semi-annual crops), which presented the highest concentrations of HMs, followed by the oil palm-cultivated soils (Figure 2). These findings can be explained by the continuous phosphorous and nitrogenous fertilizer applications in these production systems.
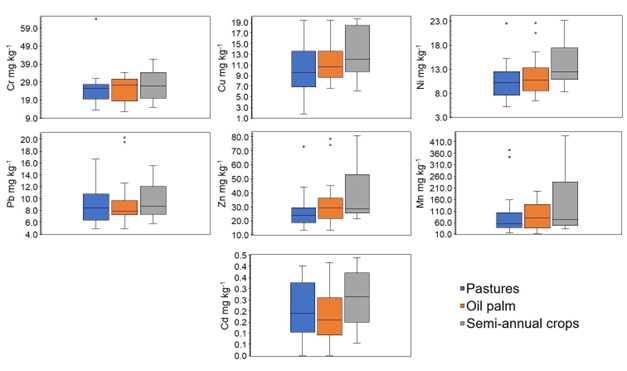
Source: created by the authors
Figure 2 Box-plot for metals and metalloid contents in the soils of three agricultural production systems at Piedemonte Llanero in Colombia. Outliers are indicated
Heavy metal pollution showing low CV is associated with natural sources, and high CV is typically sourced from human activities. Based on this criterion and the calculated CVs of the analyzed species, the CVs of Cr, Cu, Ni and Pb in the farmland soils in the study area were almost all between 25 % and 50 %, indicating moderate variability for these elements.
Mn, Zn and Cd showed a high variability for some crops, concerning their spatial distributions; this indicates that the concentrations of Mn, Zn and Cu vary significantly from one sampling site to the other, what we attribute to be affected by extrinsic factors, such as agricultural activities. In all cases, CV % exceeded 30 %, which indicates the wide variability of concentrations in the soils of each production system. García-Martínez and Poleto [24] and Trujillo-González and Torres-Mora [33] mention that this is due to the high heterogeneity of the distribution of metals in soil, which responds to these elements’ typical behavior.
The Zn in these agricultural soils obtained a mean value of 27.62 (mg kg-1) for the pasture production system, 32.79 (mg kg-1) for oil palm and 38.23 (mg kg-1) for semi-annual crops. These values are lower than those reported by Mahecha-Pulido et al. [11] for the same region with 58.6 (mg kg-1) but come close to the reference value of 28.2 (mg kg-1) obtained by Trujillo-González et al. [28]. The Cr concentrations in these soils were 25.68 (mg kg-1) for pastures, 25.15 (mg kg-1) for oil palm and 26.91 (mg kg-1) for semi-annual crops. These values are higher than those reported by Mahecha-Pulido et al. [11] of 11.9 (mg kg-1) but come close to the reference value of 21.1 (mg kg-1) [28] for the same region. The Cu values herein obtained were 10.16 (mg kg-1) for the pasture system, 11.42 (mg kg-1) for oil palm and 13.06 (mg kg-1) for semi-annual crops, which comes close to the reference value of 9.9 (mg kg-1) for the Piedemonte Llanero [28].
The mean values of Ni were 10.37 (mg kg-1) for pastures, 11.82 (mg kg-1) for oil palm and 13.80 (mg kg-1) for semi-annual crops. The soils with pastures came close to the reference of 10.2 (mg kg-1) [28]. With Pb, the three production systems remained below the reference value of 11.3 (mg kg-1) [28], with 9.24 (mg kg-1) for pastures, 9.46 (mg kg-1) for oil palm and 9.69 (mg kg-1) for semi-annual crops. Finally, the Cd concentrations in the pasture system were 0.21 (mg kg-1), 0.19 (mg kg-1) in oil palm and 0.26 (mg kg-1) in semi-annual crops. These values are lower than those reported by Yacomelo [33] in Candelaria (Atlantic) with a concentration of 1.29 (mg kg-1), and one of 1.66 (mg kg-1) in Manatí (Atlantic) and the reference value of 0.3 (mg kg-1) for the Piedemonte Llanero [28].
3.1 PCA results
As shown in Figure 3, four PCs were produced with an initial eigenvalue above 1, which represented 81.66 % of total variance. PC1 (explaining 44.01 % of total variance) comprised mainly Zn (0.91), Pb (0.83), Mn (0.71), Ni (0.86), Cu (0.77) and silt (0.66). These elements are associated with synthetic chemical fertilizers. So, it can be stated that the influence of agriculture is reflected on PC1. PC2 is represented by Cr and organic matter, possibly associated with domestic wastewater inputs. PC3 is represented by Cd (0.57), and is also associated with fertilizers, and the natural Cd concentrations in soil typically swings around 0.1 mg kg-1, and the value obtained in soil varies between 0.0 and 0.64 mg kg-1. Hence, we can affirm that Cd at Piedemonte Llanero of Colombia originated from natural sources.
3.2 Environmental evaluation
Globally speaking, ecological risk and contamination assessment of soil with HMs have been a widely concerned environmental issue [34]. There is currently a lack of knowledge regarding spatial distribution and contamination by HMs in the Piedemonte Llanero of Colombia. Multivariate analysis revealed significant anthropogenic, point and nonpoint pollution of selected metals in the area.
Three indices were used to evaluate ecological risk assessments for HMs in the agricultural soils in the study area (Table 2).
Table 2 The Geo-Accumulation Index (I-geo), the contamination factor (CF) and the Pollution Load Index (PLI).
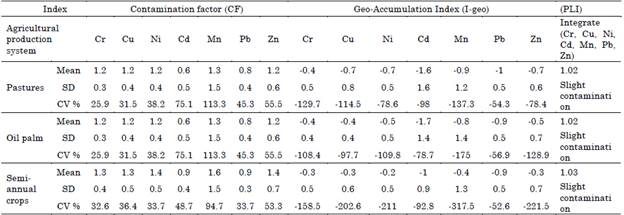
Source: created by the authors.
The CF reported low, moderate, and very high contamination categories in the soils of the three studied production systems. The Cd and Pb values for all three cases indicated low contamination. However, the soils with semi-annual crops obtained the highest CF values, with 0.9 CF units for Cd and Pb. Metals Cr, Cu, Ni, Mn, Zn fell in the moderate contamination category, and also had the highest values for the soils of the semi-annual systems. Therefore, they were influenced by anthropogenic impact and can almost be entirely explained by addition of fertilizers in each crop cycle, with two cycles a year in the area [35], [36].
The contamination levels of heavy metals in soils were also assessed by I-geo, introduced by Muller [23], which has been widely employed in European trace metal studies. Indeed, the Igeo allows the assessment of soil pollution by HMs based on the comparison of HMs contents in the surface layer with the geochemical background of the region. The I-geo mean values ranged from -0.4 to -0.3 I-geo units for Cr, -0.7 to -0.3 I-geo units for Cu, -0.7 to -0.2 I-geo units for Ni, -1.7 to -1.0 I-geo units for Cd, -0.9 to -0.4 I-geo units for Mn, -1.0 to -0.9 I-geo units for Pb and -0.7 to -0.3 I-geo units for Zn (for the three crops). The mean I-geo values indicate that agricultural soils are practically unpolluted by the presence of these elements. Only in some cases was it slightly higher than 0. Clearly, the ranking results of HMs pollution calculated by the I-geo method are the same as those calculated by the CF method. Based on the classification standard, the collected farmland soil samples in the study area were unpolluted and, in some cases, moderately polluted, but these concentrations were below the critical maximum levels above which toxicity is possible.
The PLI values calculated for the elements within the surface interval are presented in Table 2. The PLI proposed by Tomlinson et al. [27] (which integrates all the studied elements) showed that these soils fell in the slight contamination category for the three production systems. The mean values of the single pollution index and the integrated pollution index came close to 1 in the area (between 1.02 and 1.03 PLI units). Surface soil can be classified as nonpolluted in most of the study area. Thus, it was concluded that the quality of the soils of the Acacias-Pajure River Basin at Piedemonte Llanero in these three production systems (pasture, oil palm, semi-annual crops) is acceptable environmental, with no contamination traits that pose a risk for people’s health and ecosystems. In any given case it is found low soil pH values and moderate to low organic matter contents what enhance the leaching of some elements from the soil. Moreover, we also found that applied fertilizers were important sources leading to different accumulations of heavy metals in soils under the studied land use patterns.
In general, it was found that in all HMs in NIPI it was in the low level of pollution category. However, the Mn showed the highest NIPI values, where the oil palm and the semi-annual crops reached 2.0 and 1.8 NIPI units. The lowest NIPI values were presented by Pb and Cd between 1.0 and 1.1 NIPI units, other HMs varied between 1.2 and 1.5 NIPI units (Table 3).
Table 3 Nemerov Integrated Pollution Index (NIPI) in soils of three productive systems

Source: created by the authors.
Given the fragility of the soils studied due to their extreme acidity, low exchange capacity, etc. to achieve the sustainable development goals (SDGs), preservation of soil health is vital to food security and human existence itself [37]. In this sense, the agricultural practices habitually carried out in the region do not represent a risk of contamination.
4. CONCLUSIONS
This study, conducted to provide baseline information on the spatial variability and a risk assessment of selected heavy metals on the farmlands of the Piedemonte Llanero in Colombia, represent a preliminary advance in its knowledge. In this study, selected HMs (Cu, Cd, Cr, Mn, Ni, Pb, Mn and Zn) in surface soils of on farmlands at Piedemonte Llanero in Colombia were investigated by analytical methods; Also, their potential ecological risks were evaluated. The average HM concentrations in the collected topsoil samples were overall: Mn (110.5 mg kg-1), Zn (31.93 mg kg-1), Cr (8.85 mg kg-1), Ni (11.68 mg kg-1), Cu (11.28 mg kg-1), Pb (9.42 mg kg-1); Cd (0.21 mg kg-1). The study represents a preliminary advance in perhumid climate and acid soils. Despite concentrations generally being below or coming close to the reference value proposed for the Piedemonte Llanero region, the application of the I-geo, the CF, the PLI factor and NIPI index enabled us to indicate that these HMs do not currently pose environmental. Therefore, it is evident that the soil samples at the studied sites were safe for agricultural and environmental purposes. The results of this study are expected to shed light on the community’s understanding, and to enable the City Council to monitor environmental quality and to take appropriate actions at Piedemonte Llanero in Colombia.