1. INTRODUCTION
Renewable energies have gained a position as a solid alternative for achieving the decarbonization objectives and meeting the climate change challenges. Thus, models of power generation, transmission, and distribution, have experienced a significant transformation owed to the incorporation of 1) advanced technologies, such as smart metering and power electronic converters, 2) new structures, such as smart grids and microgrids, and 3) new concepts such as distributed generation and distributed control [1]. Conventional passive devices used to connect power grids' elements are not suitable for future grids due to their limitations of modularity, plug-and-play capabilities, and voltage level compatibility for sub-grids interconnection [2]. In this sense, the necessity of including power converters in these evolving systems is imperative due to their capacity for modularity and decentralized control[3].
The continuous research on microgrids and power generation based on non-conventional renewable energy sources (NCRES) has broadened the areas of study, including operation, planning, design, protection, and reliability of microgrids. Then, the evolution from the phase of study and simulation into a commissioning phase was possible. The latter includes implementation, project deployment, and commercial exploration [4]. The economic aspect is highly relevant to encouraging the financing of renewable projects, as well as their research and development. Although the deployment of non-conventional renewable energy sources was driven by environmental reasons, its economic profitability has made it a focus of attention from most sectors, including industry, academia, banking, and government. For instance, business models are emerging around that kind of projects, such as the engineering, procurement, and construction model (EPC) [5], the power purchase agreement [6], the leasing, the High-Capacity Generation Model, among others.
Although the experimentation and implementation phase of microgrids is a fact, it still requires the study of some technical aspects for an optimal and reliable operation [4]. The structure of electrical grids has suffered transformations, as well as the demand profiles with continuous load variability, affecting not only the operation of the main grid but the microgrids in immature development. Additionally, the generation intermittence from NCRES causes, among other aspects, fluctuation in the frequency and voltage signals [2], [7]. Hence, there are substantial challenges in terms of the design of controllers to guarantee the synchronization and interconnection of different power converters [2]. Also, in this emerging generation system where the grid elements interconnection is done through power converters, the problem of rotational inertia loss is inherent. Low inertia in these systems makes them prone to instability. In this sense, some control techniques based on the use of electronic power converters that emulate the behavior of a synchronous machine have been proposed [8]-[12]. For instance, grid-feeding and grid-supporting converters provide inertia issues eventually solved through droop control techniques. On the other hand, in grid-forming converters, low inertia is not a prevalent issue [8]-[10].
All last situations are been tackled by engineers, practitioners, and researchers producing and reporting a big number of solutions and even new open problems. To facilitate the incorporation of new members in the development of power converters used in microgrids, it is necessary to provide a literature review that summarizes the estate of the art in power converters used in microgrids, identifies the open problems in the topic, and makes son recommendations for future work.
The main objective of this paper is to present an overview and critical discussion about the utilization of power converters in the several microgrid configurations and its interconnections with power distribution systems. The review takes into account 69 works published in this research topic. The papers are selected from databases and editorials that classify papers in electrical engineering or more specifically distribution systems and microgrids, e.g., IEEExplore, ScienceDirect, Springer, MDPI, etc. The papers are read and classified depending of its focus, i.e. power converter in microgrids or power converters in distribution systems. The result of the review classifies the paper in the next way: in the first part, two classification of microgrids were presented using their voltage i.e., AC, DC or AC/DC and the operation mode i.e., grid-connected, islanded or both. Specifically, both classifications were derived from six reviewed papers. Subsequently, a power converters classification is proposed based on the kind of microgrid where they are used and the converters operation mode, which correspond to grid-forming, grid-feeding and grid-supporting. The last classification is made over more than 40 papers about power converters used in microgrids and electric distribution systems. Also, in that classification the type of converters is discussed in detail indicating the kind of microgrid where the converter is used, the variables that are measured, the control system topology, and the equivalent circuit that is used for representing the power converter. The equivalent circuits or reduced models are useful to designing the controllers for each converter. Then, a final classification is presented where the kind of converter, i.e., grid forming, greed feeding, grid supporting and interlinking power converters, are associated to the renewable energy application such as photovoltaic, wind, energy storage, electric vehicles, etc. Also, the advantages and disadvantages offered by those converters are highlighted and particularly the implementation domain is also included (model simulation or physical implementation).
The rest of the paper is organized as follows: Section 2 introduces the fundamental concepts of power converters (PCs), presents a classification of the most relevant PCs used in microgrids (MGs), and presents the associated control techniques. Section 3 proposes a comparative analysis of the PCs types used in different scenarios of microgrids including AC, DC, and AC/DC topologies. This section also includes a discussion about control reactive power in microgrids using smart inverters. Finally, in Section 4 the main conclusions and the future word are presented.
2. POWER CONVERTERS FOR MICROGRIDS
In this section a classification of power converters used in microgrids is presented. The classification start selecting papers of well-known databases or editorials such as IEEExplore, ScienceDirect, Springer, MDPI, etc. In the review it is evident that there are several converters used in several types of microgrids, which can be classified based on the nature of the bus voltage and based of their operation mode. It is necessary to know if any converter cab be used in any type of microgrid or which is the power converter more used in a specific type of microgrid understanding the advantages and disadvantages offered by the converter. Power converters are the main elements that enable the interconnection of Renewable Energy Sources (RES) and Energy Storage Systems (ESS) with the power grid and microgrids. In addition, to allow the interconnection of the different elements of the microgrid, the PCs are capable of other functions such as guaranteeing the power quality and reliability of the microgrid [2], [13], [14]. The classification of power converters is based on a variety of categories. But their use increased significantly with the introduction of microgrids in the power system, which, in turn, are also classified into several categories.
The nature of microgrids can vary based on the type of voltage present at the busbar, which, in the context of power quality, can be called Point of Common Coupling (PCC), i.e., based on the voltage nature at the point where all renewable energy sources and loads are connected. Microgrids can also vary in terms of their operation mode [15]. Based on the voltage at the busbar, microgrids are classified as AC, DC, and AC/DC also called hybrid microgrids. On the other hand, based on their operation mode, microgrids are classified as grid-connected (or on-grid, i.e., those connected to the main power grid) and islanded (or off-grid, i.e., those that operate autonomously) [2], [15]. Additionally, some microgrids can operate in both modes, i.e., in grid-connected mode or islanded mode for determined periods. This classification is summarized in Figure 1.
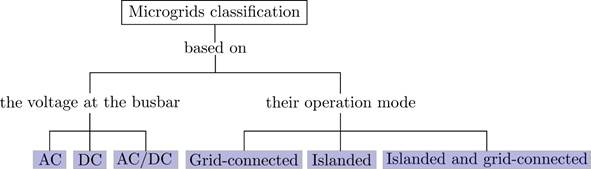
Source: Created by the authors.
Figure 1 Microgrids classification. PCC is the point of common coupling
The last classification of microgrids allows to introduce a power converter with a very clear function which is to interconnect AC/DC or hybrid microgrid. That kind of converter can be called Interlinking Power Converter (IPC), its function is illustrated in Figure 2. As can be inferred, an interlinking power converter should have the capacity to invert or rectify voltage allowing the power flow in any direction or both directions; also, when inverting the power, it has to has a specific frequency, voltage, and even more demanding, a specific power quality. Additionally, that hybrid microgrid should be connected to the utility grid or main power system through the PCC. The concept and classification of the IPC would be described forward in Subsection 2.4.
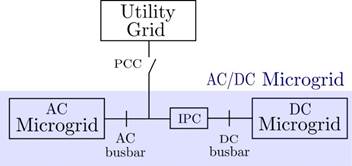
Source: Created by the authors.
Figure 2 Interconnection between DC and AC sides of an AC/DC microgrid and connection with the utility grid. IPC is an Interlinking Power Converter; PCC is the Point of Common Coupling
Since the power converters analyzed in this paper would be used in power systems that include local generation with RES and local loads, the converters must be studied and selected in this specific context. In this sense, the first step is to define the configuration and operation mode of the microgrid, to select the appropriate set of converters. As mentioned above, there are many categories of power converters, and some of them are described in the following subsections.
The synthesis of reviewed papers also shows that there is possible to classify the converters based on the form of the generated energy from the RES, i.e., DC or AC; The categories of that classification correspond to 1) DC/DC and DC/AC if the RES generates in DC and 2) AC/DC, AC/DC/AC, and AC/AC if the RES generates in AC. Those categories are well-known and therefore are not discussed here in detail, but it can be summarized in the left side of Figure 3. On the other hand, according to the operation mode, power converters can be classified into 1) grid-forming, 2) grid-feeding, and 3) grid-supporting converters [16]; see the right side of Figure 3. Because the last classification is less common, it is selected to explain the converters in the next four subsections.
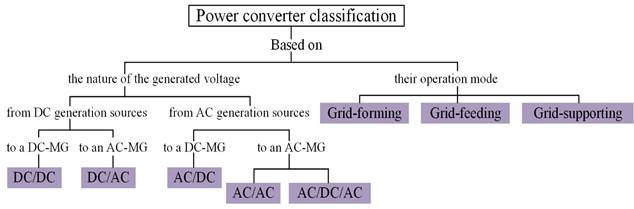
Source: Created by the authors.
Figure 3 Power converters classification. MG is the abbreviation for “microgrid”
Moreover, converters connected to the grid usually operate in Current Control Mode (CCM), which is robust to voltage disturbances in the grid. Most renewable energy-based distributed generation converters currently operate in grid-feeding mode [15]. Nevertheless, converters in island mode usually operate in Voltage Control Mode (VCM) providing the voltage and frequency references.
In the next four subsections, the grid-forming, grid-feeding, and grid-supporting and interlinking converters are presented. Each subsection describes the common representation of the converter, the renewable resource associated, the microgrid application, and the regulated variable associated with the type of microgrid. Also, a complete structure is presented to describe the type of microgrid, the type of control system, the manipulated and regulated variables and the low order representation. Because the results of the robustness and reliability of control systems is presented in the analyzed papers, those results are not presented here in sake of brevity.
2.1 Grid-forming power converters
Grid-forming power converters can be set up as an ideal AC voltage source with a low series impedance as illustrated in the green frame of Figure 4. These converters are associated with ESS. They commonly operate in VCM since they are essential in isolated microgrids, although they can also operate in grid-connected mode in AC and DC networks. These converters are essential in the operation of isolated microgrids to form the network, especially when other PCs, such as grid-feeding (described later in Section 2.2), are connected to the grid, i.e., at least one of the PCs connected to the grid is required to operate as grid-forming [2], [8], [16]-[18]. The illustration of this converter operating in VCM is shown in Figure 4 with the differentiation for both microgrid types, i.e., AC-MG in blue dotted line and DC-MG in green solid line. The terms that apply to both microgrid types are represented with a black solid line. In Figure 4, |v o|* is the magnitude of the voltage reference, ω * is the angular frequency of reference, i.e., the grid frequency, and i o * is the current reference. v o and i o are the output values of voltage and current, respectively. The term “MG bus” represents the busbar of either the AC microgrid or the DC microgrid.
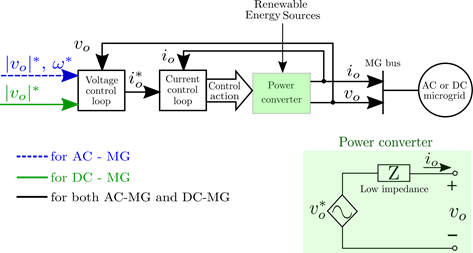
Source: Created by the authors.
Figure 4 Grid-forming power converter scheme in VCM mode. MG is the abbreviation for “microgrid”
2.1.1 Grid-connected operation
When a grid-forming converter is connected to the grid, it is responsible for adjusting the power values of the sub-networks. In the case of AC networks, it adjusts the values of active power, P, and reactive power, Q; while in the case of DC networks, it adjusts the value of active power, P. Additionally, in this operation mode, the grid-forming converter helps to maintain the State of Charge (SoC) of the ESS and, at the same time, improving the voltage profiles in DC networks or the power quality in AC networks [2].
2.1.2 Islanded operation
In this mode, grid-forming converters are responsible for setting the local network references. In the case of AC networks, they generate a sinusoidal voltage with the desired amplitude and frequency; while in DC networks, they set the DC voltage with the desired reference [2].
2.2 Grid-feeding power converters
These converters can be set up as an ideal AC current source with a high parallel impedance as illustrated in the green frame of Figure 5. These converters are also associated with non-dispatchable RES. They commonly operate in CCM since they are frequently used in grid-connected microgrids. Though they can operate with several paralleled grid-feeding converters, their behavior tends to be unstable [2], [15]-[17], [19], [20]. The illustration of this converter operating in CCM is shown in Figure 5, with the differentiation for both microgrid types, i.e., AC-MG in blue dotted line and DC-MG in green solid line. The terms that apply to both microgrid types are represented with a black solid line. In Figure 5, P * and Q * are the reference values for active and reactive power, respectively, i o * is the reference value of current, and v o and i o are the output values of voltage and current, respectively. The term “MG bus” represents the busbar of either the AC microgrid or the DC microgrid.
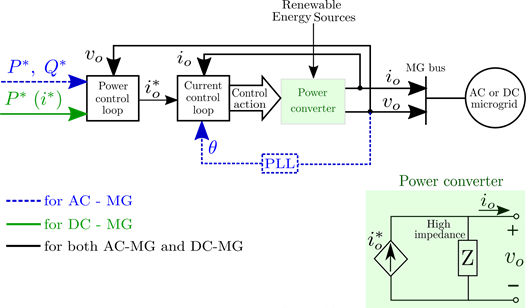
Source: Created by the authors.
Figure 5 Grid-feeding power converter scheme in CCM mode. MG is the abbreviation for “microgrid.” PLL is the Phase-Locked Loop
2.2.1 Grid-connected operation
In this mode, grid-feeding converters are responsible for injecting a specific amount of either power or current to the sub-grid while following the reference values. For instance, in the case of AC networks, these PCs inject active power P and reactive power Q, while following the voltage and frequency references V and f. In the case of DC networks, these PCs inject either active power or current while following the voltage reference V.
2.2.2 Islanded operation
In this mode, grid-feeding converters can only inject power or current if there is at least one grid-forming converter (or one synchronous generator) operating and generating the AC or DC voltages in the MG’s bus. Otherwise, in island mode, grid-feeding converters are unable to operate because they need a voltage reference to follow [2], [17], [18].
2.3 Grid-supporting power converters
Grid-supporting converters can operate in both control modes, i.e., CCM and VCM. In VCM, they can operate autonomously either in islanded (IS) or grid-connected (GC) mode. However, in CCM these converters require at least one grid-former converter that provides the synchronous generator behavior and the proper references [2], [15], [16]. Moreover, those converters can contribute to the stabilization of the grid in both control modes (VCM and CCM). So, they contribute to the primary regulation through droop control strategies, as will be discussed in the next subsections 2.3.1 and 2.3.2 [2], [21]-[23].
2.3.1 Current control mode operation
As discussed in Section 2.2, grid-feeding converters cannot operate in IS mode without assistance from either other PCs or a synchronous generator. Then, grid-supporting converters are suitable to provide support for the function’s performance of these converters, such as the regulation of active and reactive power (only active power for DC microgrids) and setting the voltage and frequency references (only voltage for DC microgrids). In GC mode, grid-supporting converters can also aid the grid-feeding converters in the load supply function and contribute to the regulation of voltage and frequency for both the microgrid and the main grid. The illustration of this converter operating in CCM is shown in Figure 6, with the differentiation for both microgrid types, i.e., AC-MG in blue dotted line and DC-MG in green solid line. The terms that apply to both microgrid types are represented with a black solid line. In Figure 6, P ** and Q ** are the reference values generated through the droop control equations for active and reactive power, respectively. P * and Q * are the reference values for active and reactive power, respectively, which are externally provided. Likewise, v o *is the magnitude of the voltage reference and ω * is the angular frequency reference, i.e., the grid frequency. i o * is the reference value of current, and v o and i o are the output values of voltage and current, respectively. Additionally, in an AC-MG the terms ω *− P * and v o *−Q * represent the droop curve of ω * against P * and the droop curve of v o * against Q *, respectively. v o * − i o * represent the droop curve of v o * against i o * in the DC-MG scenario.
2.3.2 Voltage control mode operation
Grid-forming converters discussed in Subsection 2.1, are occasionally unable either to maintain the voltage and frequency references in IS mode or to preserve the power quality in GC mode. Then, grid-supporting converters are also a proper alternative for supporting these converters' functionality. In IS mode they act as an additional distributed generator (DG) with similar features to a synchronous generator providing the voltage and frequency references (only voltage for DC microgrids). In GC mode they contribute to the regulation of voltage and frequency (voltage for DC microgrids) values and also contribute to the improvement of the power quality (voltage profile for DC microgrids) in the microgrid [2], [16], [18]. The illustration of this converter operating in VCM is shown in Figure 7, with the differentiation for both microgrid types, i.e., AC-MG in blue dotted line and DC-MG in green solid line. The terms that apply to both microgrid types are represented with a black solid line. In Figure 7, P ** and Q ** are the reference values generated through the droop control equations for active and reactive power. P * and Q * are the reference values, which are externally provided. Likewise, v o * is the magnitude of the voltage reference and ω * is the angular frequency reference, i.e., the grid frequency. i o * is the reference value of current, and v o and i o are the output values of voltage and current. In an AC-MG the terms ω * v o * P * and v o * − Q * represent the droop curve of ω * against P * and the droop curve of v o * against Q *, respectively. v o *− i o * represent the droop curve of v o * against i o * in the DC-MG scenario.
2.4. Interlinking power converters
Interlinking power converters (IPCs) are power electronic devices that facilitate the connectivity of multiple sub-grids with either the same nature or different nature and architectures, i.e., AC/AC, DC/DC, AC/DC, even AC/AC with different frequencies, and DC/DC with different voltages. But, their most frequent use is in the interconnection of AC/DC sub-grids, i.e., in hybrid microgrids [2], [21]. For instance, the IPC in Figure 2 interconnects a DC sub-grid with an AC sub-grid for an AC/DC microgrid. IPCs also operate as energy routers in the grid, i.e., provide the energy management of all renewable energy sources to distribute it dynamically along the grid [24], [25]. A remarkable characteristic of IPCs is that the primary and secondary sides are not necessarily fixed compared to the conventional power converters.
IPCs can even perform the equivalent functions of other PCs like the grid-forming and the grid-supporting converters. These converters provide a high quality of power flow, as well as the PCs mentioned. However, this fact limits the maximum utilization of the energy generated by the RES, which compromises the RES efficiency [2], [26], [27]. These power converters can be classified as shown in Figure 8.
The following subsections describe some of these topologies, their most distinctive features, and their use. For instance, the Bidirectional Power Converter (BPC), the Buck/Boost converter, the Isolated Bidirectional DC/DC Converter (IBDC), the Back-to-Back (B2B) converter, and finally the Solid-State Transformer (SST).
2.4.1 Bidirectional power converter
Bidirectional Power Converters (BPC) are devices capable to support the power between AC and DC sub-grids, maintaining a dynamic power equilibrium in the system. These converters can operate in parallel with other BPCs. Today's power systems use bidirectional converters broadly given their bidirectional power flow capability, specifically in microgrids' applications, such as, the charge of electric vehicles (EVs), renewable energy sources (PV, Wind, etc.), and ESS, as well as, in uninterruptible power supplies (UPS) and in aerospace. The primary control techniques for these converters are studied based on their configuration, i.e., single BPC or parallel BPCs [28]-[30].
2.4.2 Buck/boost power converter
The Buck/Boost is a bidirectional converter used in the isolated DC/DC sub-grids interconnection where the voltage levels are similar but not equal. In the case of the same voltage levels, additional capabilities are required. These converters do not provide galvanic isolation [31], [32]. For the AC/DC sub-grids interconnection, this converter can be used through the inclusion of a voltage source inverter (VSI) on the AC side. This configuration is known as Buck/Boost + VSI [33]- [35].
2.4.3 Isolated bidirectional DC/DC converter
The Isolated Bidirectional DC/DC Converter (IBDC) is a variety of bidirectional converters used in DC/DC microgrids. This classification includes the dual active bridge (DAB) and the Flyback converters. Compared to the Book/Boost converter, the IBDC provides galvanic isolation. The DAB is the IBDC most used as suggested in several papers [36]-[39], although flyback is also used [40]. The application of these converters is also possible in the AC-DC interconnection. For instance, in [41] the configuration of a microgrid connected to the grid is studied, where a bidirectional AC-DC converter is used to integrate a DC distribution network to the utility grid.
2.4.4 Back-to-back converter
The Back-to-Back (B2B) converter has been mostly used in the interconnection of AC sub-grids, either an AC sub-grid to an AC sub-grid or an AC sub-grid to the main grid [21], [42]. Also, back-to-back converters are often used in the transmission of high voltage DC power systems [43]. In the interconnection of AC/AC sub-grids, the B2B topology is formed with two stages (2-stage), where a DC bus is between each AC side. Then, one stage of conversion goes from one of the AC sides to the DC bus, and the other conversion stage continues from the DC bus to the other AC side. This 2-stage structure enables the interconnection of multiple AC asynchronous sub-grids and is illustrated in Figure 9. On the other hand, this topology can be used in the scenario of AC/DC sub-grids interconnection. Here the DC sub-grid would be used as a DC-MG where energy storage systems, distributed generators, and loads can be connected to [23], [42], [44]-[53].
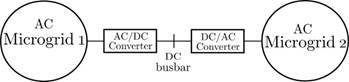
Source: Created by the authors.
Figure 9 Back-to-back topology of two stages for an AC-AC sub-grids interconnection
Despite back-to-back assuring the backup capability under a grid failure and maximizing the use of RES, the efficiency of the power conversion is not the best feature, due to the two power conversion stages. In this sense, this type of IPCs is usually used for ancillary services in combination with direct connections, i.e., without power conversion stages.
2.4.5 Solid-state transformer
A solid-state transformer (SST), also known as a smart transformer, is a power electronic-based transformer with the capability of transforming power and providing power control services. In the SST topology is usual to find three stages of conversion, where an IBDC is incorporated in the middle [21].
As in the back-to-back topology, the SST permits the integration of energy storage systems and distributed generators. But it also allows galvanic isolation compared to back-to-back, which facilitates the connection of sub-grids with different voltage levels. Figure 10 illustrates the three stages topology of an SST with galvanic isolation, which is represented for the DC/DC converter in green color. This topology can be used in AC/AC sub-grids interconnection but is not the best choice given the incorporation necessity of a DC link, not commonly found in these systems [54]-[59].

Source: Created by the authors.
Figure 10 Solid State Transformer topology of three stages for an AC-AC sub-grids interconnection
Some important aspects regarding the IPCs presented are: The 3-stage SST topology described in Subsection 2.4.5 provides many advantages to solving different issues in the AC/AC sub-grids connection. However, it is still an expensive and complex topology to implement, while using simpler topologies can work effectively in this microgrid configuration. If the use of DC links in these scenarios increments in the future, then the 3-stage SST topology would be a suitable choice.
In the DC/DC isolated microgrids, Buck/Boost, DAB, and flyback converters are some alternatives for use in this scenario. If voltage levels are similar, Buck/Boost can be used, but if galvanic isolation is required, either DAB or flyback converter are suitable options. However, the most used BPCs topologies are the full-bridge ones, i.e., the DABs, and more research is desired for flyback converters.
The back-to-back converter does not include galvanic isolation. For this purpose, a power transformer must be included. This fact makes this choice expensive and less efficient. It is desirable to choose either a DAB or a flyback topology for the DC/DC interconnection.
3. CLASSIFICATION OF POWER CONVERTERS BASED ON THEIR APPLICATION, ADVANTAGES, DISADVANTAGES, AND IMPLEMENTATION
Another classification of a group of papers related with power converters is in Table 1. In this case the power converters are presented according to their role in the microgrid or distributed energy system. Then, the applications, which correspond to distributed generators, energy storage systems, photovoltaic arrays, wind generators, and electric vehicles. The more relevant advantages and disadvantages of the converters are listed for each role; Finally, the type of implementation between simulation and laboratory is presented citing the analyzed papers.
Table 1 Power converters classification based on their application, advantages, disadvantages, and implementation
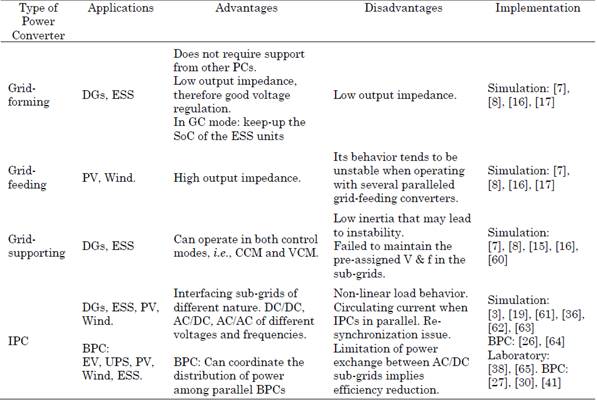
Source: Created by the authors.
Table 1 shows that power converters in the role of grid-forming do not require support for others converters and are typically used with distributed generators and energy storage systems. Those converters are high power, and the low output impedance allow them to give high current without affecting their voltages, i.e., they are capable to regulate the voltage, and frequency in AC or hybrid microgrids. However, low output impedance has some disadvantages if the converter is required to operate in parallel with other sources generating circulating currents among the sources in parallel. Also, the low impedance makes the converter more susceptible to disturbance in the microgrid. On the other hand, among the reviewed papers related with grid-forming converters, the simulation was the preferred way to develop the implementation. There is an opportunity to test the several published solutions in real environments or laboratories.
Grid-feeding converters are used to connect photovoltaic arrays and wind generators in parallel with other sources thanks to its high output impedance, which limits circulating currents improving the stability of the microgrid. However, in the same parallel operation, the behavior of the grid-feeding converters tends to be unstable if the other sources have high output impedance or they are grid-feeding converters. Simulation is preferred over laboratory implementation among the reviewed papers.
Grid-supporting converters are used with distributed generators and energy storage systems or battery packages. They can operate in both control modes, voltage control mode and current control mode. Those control modes offer the possibilities to protect the source or the load as required, i.e. grid-supporting configuration is flexible to connect sources in the microgrid. Among other disadvantages, as cost, complexity, efficiency and dependence of weather conditions, there is the low inertia that makes the microgrid susceptible to instability.
The type of converter that reunite most of the converters used in microgrids is the interlinking power converter. As can be seen in Table I, this converter is used to connect distributed generators, storage energy systems, photovoltaic arrays and wind generators. As bidirectional power converter can be used to connect electric vehicles, photovoltaic arrays, wind generators, and uninterruptible power supplies with battery packs. By far the main advantage of IPCs is to interconnect subsystems or microgrids with different voltages and frequencies in the case of AC/AC microgrids. When those converters are used to interconnect AC/DC microgrids, the efficiency can be reduced due the different size of the systems. When interconnecting AC/AC microgrids the problem issue is the resynchronization of the systems. It is normal that the most spread type of converter presents a lot of problems in contrast with a big number of solutions.
For islanded microgrids a hierarchical control strategy was proposed and three inverters were used as actuators to share the reactive and real powers. The control strategy consists of an inner control loop, primary control, and secondary control [66]. Also, the improvement of power quality in distribution networks is achieved using smart inverters through the control of voltage and reactive power [67]. Classical control techniques, predictive control techniques, and iterative algorithms are proposed to control a DC microgrid. The microgrid and the control systems are modeled and implemented in Simulink [68]. A review on optimal control applied to smart power substations was presented in [69]. The review highlights the area, the problem, constrains, and approaches identified in 20 papers about optimal control in substations.
4. CONCLUSIONS
This paper presented the review of papers related with power converters used in microgrids and distribution systems. First, the classification of microgrids were presented using the voltage nature i.e., alternate current, direct current, or alternate current/ direct current and the operation mode i.e., grid-connected, islanded or both. Subsequently, the classification of power converters was presented based on the kind of microgrid where the power converters were connected and their operation mode, which correspond to grid-forming, grid-feeding and grid-supporting. For the last classification, the converters are discussed in detail indicating the next: the kind of microgrid where they are used, the variables that are commonly measured, the structure of the control system, and the equivalent models that are used for their representation.
From the literature review five topics were identified: First, a gap was identified in the study of direct current/ direct current microgrids despite being the simplest configuration among the three analyzed configurations. This is because hybrid and AC microgrids are more widely used since they allow taking advantage of the infrastructure of the current electrical systems. Another conclusion was made with grid-supporting converters, which offer low inertia; however, the incorporation of droop control techniques in their operation can solve this issue, e.g., the use of interlinking power converters is a recommended option if primary regulation is required, since it can include droop control strategies in its operation. The third topic is also related with interlinking power converters, which are a recommended choice if asynchronous interconnection of sub-grids is required, even if the sub-grids are different in voltage nature, levels, or frequency.
For future work, the literature review showed that a main focus should be in back-to-back converters because they are flexible connecting systems of different nature. The development of detailed models of the power converters will allow the design of sophisticated control systems increasing efficiency and increasing the range of operation of the power converters.