1. INTRODUCTION
Health-care-associated infections, which are acquired during hospital stays, could represent a serious risk to human health [1]. The pathogens responsible for this kind of infections (also known as nosocomial) include bacteria, viruses, and fungi. The most common bacterium is Escherichia coli (E.Coli), and it causes acute urinary tract infections, urinary tract sepsis, and neonatal meningitis [2]. The World Health Organization (WHO) estimates that, in North America and Europe, nosocomial infections cause 5 % and 10 % of the hospitalizations, respectively. In Latin America and Asia, the proportion of hospitalizations due to the same cause is approximately 40 % [3].
Accordingly, the general interest in developing antibacterial materials has grown in recent years. Hybrid nanomaterials have potential in this regard because they facilitate the use of nanostructured materials with specific properties and excellent mechanical properties, as well as improved biocompatibility and antibacterial activity. This makes them good prospects in tissue engineering [4].
On the one hand, graphene oxide (GO) has attracted special attention because it is a carbon atom monolayer that forms a dense honeycomb structure containing carboxylic groups on the edges, as well as hydroxyl and epoxide groups on its two accessible sides. GO has excellent physicochemical properties, large specific surface, mechanical resistance, electrical conductivity, and stability in water [5], [6]. However, its antibacterial activity is quite controversial, and some authors have shown that it is related to the size and shape of GO [7], [8].
Nowadays, Hummers’ method is the most widely implemented strategy to synthesize GO used in bacterial applications [9], [10]. Nevertheless, it uses dangerous reagents that are harmful to the environment and humans [11]. Electrochemical exfoliation of graphite is an alternative method for obtaining graphene oxide; it is simple and requires less reagents for the synthesis process than Hummers’ method [12], [13].
On the other hand, titanium dioxide (TiO2) is considered a suitable material for medical applications due to its low toxicity, excellent thermal properties, and chemical stability. Additionally, TiO2 exhibits antibacterial activity against E. Coli due to oxidative damage to the cell membrane [14], [15]. Thanks to these properties, TiO2 has been used in several applications, such as microbatteries and as UV absorber in cosmetic products, anticorrosive coating, and antibacterial coatings [16], [17].
The main objective of this study was to evaluate the antibacterial activity of synthesized graphene oxide (GO), titanium dioxide (TiO2) and a TiO2/GO hybrid. The combination of two types of nanoparticles with different morphologies and dimensions is a new way to produce functional hybrid materials with a synergistic improvement in material performance.
Recently, several hybrids composed of GO and conventional semiconductors demonstrated significantly enhanced photocatalytic performance during pollutant degradation. Specifically, TiO2/GO is a hybrid that could be considered as a promising material photocatalysis and hydrogen evolution [18]-[20], solar mineralization [21], and others fields of engineering such as tissue engineering as hybrid filler of functional nanocomposites [22].
Escherichia coli was the model bacteria used here to evaluate the bactericidal effect of the three materials. This study was conducted to contribute to the ongoing discussion about the antibacterial properties of GO. In addition, to the best of the authors’ knowledge, the antibacterial properties of GO synthetized by the electrochemical method had not been reported before. The electrochemical method can be used as an alternative to produce GO because it is sustainable and more environmentally friendly.
2. METHODOLOGICAL ASPECTS
2.1 Materials
All the materials employed here were reagent-grade and used without any further purification. Titanium isopropoxide (Ti[OCH(CH3)2]4, 97 %, Sigma-Aldrich), ethanol (99.5 % J.T Baker), high-purity graphite rods (3x305 mm, 99.9 %, SPI supplies), sulfuric acid (H2SO4, 96 %, Fisher Scientific) and sodium sulfate (Na2SO4, 99 %, Chemi) were used for the synthesis of TiO2 and graphene oxide. All the solutions were prepared using deionized water.
2.2 Methods
2.2.1 TiO2 synthesis
TiO2 nanoparticles were synthesized by the hydrothermal method [23]. A total of 10 mL of titanium isopropoxide (TTIP) were added to 13.3 mL of ethanol under constant stirring; then, 16.6 mL of deionized water were added slowly drop by drop to the TTIP-ethanol solution under constant stirring. The white precipitate thus obtained was stirred for 2 h, transferred to a Teflon autoclave, and heated at 80 °C for 4 h in an oven furnace (Binder, KB 105). After that, the autoclave was allowed to cool down to room temperature, and the material was filtered and dried overnight at 80 °C. Finally, said material was calcinated at 400 °C for 4 h in a tube furnace (Nabertherm, P 330) to favor the formation of the crystalline phase anatase and remove waste products.
2.2.2 GO synthesis:
GO was synthesized by the electrochemical exfoliation method. An electrolyte solution of Na2SO4 at 0.1 M was prepared in deionized water and the pH was adjusted (~6.5 to 7) with H2SO4. Then, two graphite rods were immersed in the electrolyte solution using a working distance of 20 mm, and they were connected to a DC voltage source (10 V). Finally, the product was washed several times with water and ethanol and dried in an oven at 80 °C.
2.2.3 TiO2/GO composite synthesis
The TiO2/GO composite was prepared mixing the materials previously synthesized in a 1:3 wt. % ratio using a Q500 Sonicator (20 kHz, Qsonica LLC, USA). The ultra-sonication probe tip was immersed directly in the suspension, and the supplied energy was adjusted at 30 % of the maximum capacity in order to avoid heating problems. Ultrasound pulses were applied 1 s ON and 2 s OFF during 15 minutes. Finally, the sample was filtered and dried in an oven at 80 °C.
2.2.4 Antimicrobial test
The antimicrobial activity of TiO2,GO and the TiO2/GO hybrid nanomaterial was evaluated using the macrodilution method, as described in our previous paper [24]. Escherichia coli (ATCC 25922) was selected as gram-negative bacteria model. For each experiment, bacterial stocks were grown in BHI (Brain Heart Infusion) for 24 hours in an incubator at 37 °C and 80 % relative humidity. Later, the inoculum was prepared in the range reported in McFarland’s standard No. 0.5 (1-2 x 108 CFU/ml; OD at 625 nm: 0.08-0.13) [25]. For the test of bactericidal activity, dilutions of different concentrations (1000, 500, 250 and 125 µg/mL) of TiO2, GO and TiO2/GO were prepared. After that, 10 μL of the inoculum were placed into 2 mL tubes containing 1.5 mL of each dilution prepared. As control sample, 10 μL of bacteria inoculum were placed in BHI. After that, the materials were incubated at 37 °C and 80 % relative humidity for 24 h under constant agitation. Bacterial concentrations were determined by measuring optical densities (ODs) at 625 nm with a spectrophotometer (8453A, Agilent). All the experiments were performed in triplicate. Bacterial viability was calculated using (1).
Where sample OD is the absorbance of each sample tested with the material dilutions, and control OD is the optical absorbance of the untreated sample.
The Minimum Inhibitory Concentration (MIC) required to inhibit the growth of 50 % of organisms (MIC50) was calculated using and the selection criterion was R2 ≥ 0.99.
2.3 Physical-chemical characterization
The phase identification and lattice structure characterization of the synthesized TiO2 were performed using an X-ray diffractometer (PANalytical Empyrean Series II), operated with Cu Kα radiation (λ = 1,540 Å). The thermal stability was characterized by thermogravimetric analysis (TGA) using a discovery 550 Thermogravimetric Analyzer with a heating program, initially, under a nitrogen atmosphere up to 800 °C. Subsequently, the atmosphere was changed to air. The functional groups present in the samples were analyzed by infrared spectroscopy in a IRTracer-100 spectrophotometer with wavelengths between 500 and 4000 cm-1. The numbers of defects and layers in the GO were estimated by a LabRAM HR Raman spectrometer (Horiba-Jobin Yvon). [26] The structural morphology of the particles was evaluated using a JEOL 7100F scanning electron microscope (FE-SEM) and transmission electron microscopy (TEM) in a TMP-FEI equipment.
3. RESULTS AND DISCUSSION
Figure 1a and 1b show the X-ray diffraction pattern of TiO2 nanoparticles and the Raman spectra of graphene oxide, respectively. The XRD pattern (Figure. 1a) shows anatase-phase TiO2 with characteristic diffraction peaks of 20 values at about 25.3°, 37.8°, 48°, 53.9° and 55°, they are attributed to the (101), (004), (200), (105) and (211) planes, respectively. These results are in agreement with those reported by. [27] and match standard ICDD card No. 01-073-1764 for anatase.
Figure 1b shows the Raman spectra of graphene oxide, with three characteristic bands: D-band (~1339 cm-1), G- band (~1583 cm-1) and 2D-band (~2686 cm-1). The G-band represents the sp2 hybridization of carbon atoms [28], while the D-band is related to changes in the hybridization of carbon atoms (sp2 to sp3) [29]. The change in the hybridization can be related to oxygen functionalities in graphene oxide [30]. The D/G ratio is commonly used to evaluate the number of defects in graphene oxide. The GO obtained here exhibited a D/G ratio of 0.61, which is consistent with what was found [31]. Additionally, this D/G ratio is lower than that of the GO obtained by Hummers’ method [32], [33]. The 2D/G ratio is used to estimate the number of layers in graphene oxide. In this case, the GO presented a 2D/G ratio of 1.41, which suggests the presence of bilayer graphene oxide [34].
Figure 2 shows the FTIR spectra of TiO2, GO and the TiO2/GO composite obtained in this study. All the samples present a band between 3000 cm-1 and 3377 cm-1, which corresponds to the stretching vibration of the O-H bond. The spectrum of TiO2 shows a band around 1610 cm-1 related to the bending vibration mode of the O-H bond, and characteristic bands of titanium dioxide are located at 400 cm-1 and 800 cm-1. The spectrum of GO shows several bands, but the one at 2939 cm-1 is due to C-H stretching vibration, which occurs during the synthesis process [35]. The bands at 1380 cm-1, 1220 cm-1 and 1060 cm-1 correspond to C-O, C-OH and C=O functional groups [36], [37]. The spectrum of TiO2/GO exhibits new bands at 1690 cm-1 and 1155 cm-1, which are related to C=O and Ti-O-C cm-1, respectively [38]. The presence of Ti-O-C and C=O functional groups demonstrated the interaction between oxygen functionalities of graphene oxide and TiO2 nanoparticles [39].
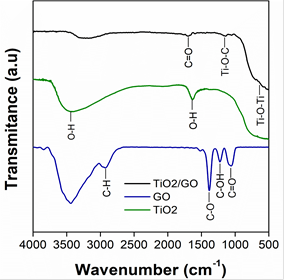
Source: Own elaboration
Figure. 2 FTIR of the materials synthesized in this study: TiO2/GO, GO and TiO2
The thermal stability of the GO and TiO2/GO was examined by TGA. Figures 3a and 3b show the TGA and DTG results of each type of sample. The weight loss below 100 °C is due to the decomposition of interstitial water [40]. Also, the weight losses between 130 °C and 250 °C correspond to hydroxyl groups (OH) and the initial degradation of carbonyl groups (C=O) [41], [42]. All the samples presented a weight loss in the thermogravimetric curve between 290 °C and 800 °C; however, the change is not significant, and it cannot be observed in the DTG curve. This weight loss is due to the partial elimination of epoxy groups (C-O) [43]. The amount of TiO2 was determined at 23.2 wt. % by the weight of the inorganic residues at the end of the TGA analysis. The increase in the thermal stability of TiO2/GO could be due to the presence of Ti-O-C bonds [44].
TGA and FTIR analyses confirmed the presence of oxygen-containing functional groups. These oxygen functionalities can be related to the oxidation of graphite by hydroxyl anions. The OH- anion attacks the sp2 carbon atoms at the edge of graphite rods, causing the expansion of layers [45]. The presence of oxygenated functional groups favors the stability of GO in an aqueous medium since these groups make GO hydrophilic [46], [47].
The morphology of the materials analyzed in this study was evaluated by SEM and TEM. Figures 4a, 4b, and 4c are SEM micrographs of TiO2, GO and the TiO2/GO composite, respectively. Figure 4a reveals that TiO2 nanoparticles have a spherical morphology. On the other hand, the micrograph of GO (Figure 4b) shows a thin graphene oxide layer; as a result, the background is visible. The micrograph of TiO2/GO (Figure 4c) presents TiO2 nanoparticles deposited on a GO layer. In addition, the TEM micrograph of GO (Figure 4f) confirms the exfoliation of graphite rods, which is a that involves the evolution of several gaseous species (SO2, O2, CO and CO2) between layers. These species come from the reduction of the SO4 -2 anion, water oxidation and carbon corrosion [48]. In addition, the TEM micrographs and the Raman analysis confirm the presence of few-layer graphene oxide. The average diameter of the TiO2 particles in the SEM images was measured by ImageJ software using more than 250 nanoparticles. Figure 4g shows the mean particle size: 24.1 ± 4.6 nm.
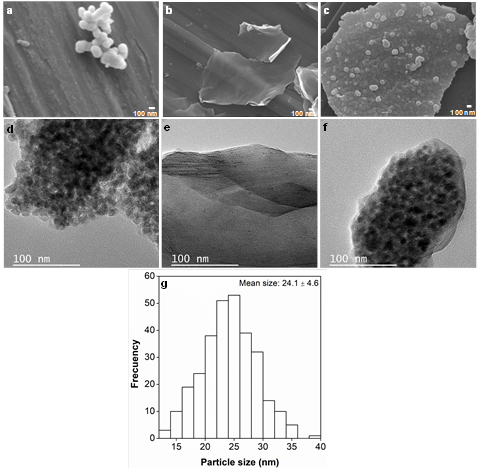
Source: Own elaboration
Figure 4 SEM micrographs of (a) TiO2, (b) GO and (c) the TiO2/GO composite. TEM micrographs of (d) TiO2, (e) GO and (f) the TiO2/GO composite (g) Histogram of TiO2 particle size distribution
The chemical composition of the TiO2/GO composite was obtained by Energy Dispersive X-ray Spectroscopy (EDS). Figure 5 shows the EDS spectrum of the hybrid nanomaterial NM and Ti; O and C were found in the sample. Additionally, sodium (Na), which can also be observed in the spectrum, is related to remnants of electrolyte (Na2SO4) used during the synthesis of graphene oxide.
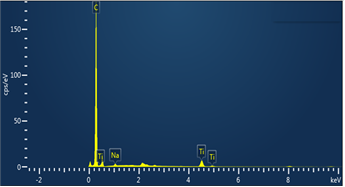
Source: Own elaboration
Figure 5 Representative energy dispersive spectrum (EDS) of the TiO2/GO composite
Figure 6 shows the element distribution map obtained from the TiO2/GO composite. The map identified the same elements found in the EDS spectrum (C, Ti, O and Na). In addition, the maps clearly show that the nanoparticles of titanium dioxide are located on the surface of the GO layers.
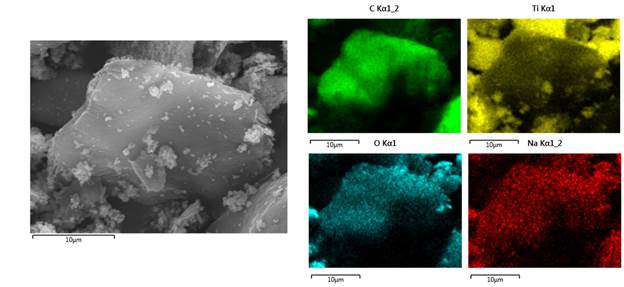
Source: Own elaboration
Figure 6 Representative element distribution map of the TiO2/GO composite. Source: Own elaboration
The antibacterial activity of the samples prepared here was determined by the macrodilution method. Figure 7 shows the bacterial viability of E. coli under different concentrations of GO, TiO2 and TiO2/GO. In the results, the graphene oxide sample presents an increase in the viability of E. Coli when the concentration of the treatment is higher.
However, the TiO2 nanoparticles show an inverse trend, i.e., the viability of E. Coli decreases as the concentration of nanoparticles increases. At the lowest concentration (i.e., 125 µg/mL), the viability of the bacteria in the TiO2/GO hybrid material was 23 % and 17 % lower than in the GO and TiO2 samples, respectively. Nonetheless, at higher concentrations (250, 500 and 1000 µg / mL), the antibacterial activity increased in GO samples but decreased in samples containing TiO2 nanoparticles. These results indicate that each treatment follows a different trend in terms of bactericidal activity as a function of concentration.
The antibacterial activity of graphene oxide (GO) reported in several papers is controversial. Nevertheless, such activity can be explained by three main mechanisms: membrane rupture, oxidative stress and isolation of bacteria envelope [49]-[51]. The high antibacterial efficacy of graphene oxide is due to the damage it causes to cell membranes through the generation of reactive oxygen species and sharp edges of graphene oxide [49], [52], [53]. In contrast, a large number of studies indicate that this material can promote bacterial growth due to its surface functional groups and because it can act as a scaffold for bacterial attachment, proliferation, and biofilm [49], [54]-[57], which could explain the results reported in this paper.
On the other hand, TiO2 nanoparticles affect biological systems since their photocatalytic activity generates potential reactive oxygen species (ROS) on their surfaces. ROS cause peroxidation of phospholipids in the cell membrane, inducing its breakdown [58], [59]. The membrane rupture causes the interruption of cellular respiration. For this reason, TiO2 nanoparticles have a strong antibacterial activity, which is in line with the results reported here, where they achieved the greatest reduction in bacterial viability.
Finally, the behavior of the composite material was similar to that reported by [50] and [60] because carbon blocks the active sites on the surface of TiO2. Therefore, the contact between the TiO2 surface and the bacteria can be very limited.
In addition, MIC50 is the minimum amount of drug/compound to inhibit 50 % of microorganism growth and was calculated. GO MIC50 could not be determined because bacterial viability does not decrease with increasing concentration. The MIC50 of TiO2/GO and TiO2 was 4575 µg/mL and 1728 µg/ mL, respectively, which confirms that TiO2 shows a higher bactericidal activity than GO and TiO2/GO.
4. CONCLUSIONS
This study assessed the effect of TiO2, GO, and TiO2/GO nanocompounds on bacterial activity against Escherichia coli. Using the hydrothermal method, TiO2 nanoparticles were obtained with an anatase phase and an average size of 24.1 ± 4.6 nm. Graphene oxide was obtained through electrochemical exfoliation, a simple and environmentally friendly method that induced a lower amount of oxygen-containing functional groups on the GO surface. A 23.2 % TiO2 content was evidenced in the developed hybrid nanomaterial. Furthermore, GO promoted bacterial growth due to its surface functional groups. TiO2 exhibited stronger bactericidal activity than the TiO2/GO compound. This behavior may be associated with the decreased contact between TiO2 and bacterial cells due to the blocking of active sites on the TiO2 surface by graphene sheets.